Polysaccharide-based near-infrared fluorescence nanoprobes for cancer diagnosis
Abstract
Near-infrared fluorescence (NIRF) imaging is most exciting and rapidly progressing area for sensitive cancer detection at the early stage and management of tumors. Recent advances of nanobiotechnology allow researchers to combine new nanoprobes with NIRF imaging techniques. Among a variety of nanomaterials, polysaccharide-based nanoparticles have been extensively investigated for biomedical applications due to their biocompatibility and biodegradability, cost effectiveness, and the ease of modifications, and so on. The main focus of this article is to describe the targeting approaches (i.e., passive targeting via enhanced permeability and retention effects and microenvironments, and active targeting) of polysaccharide-based NIRF nanoprobes, and review their primary applications for cancer in vivo molecular imaging.
Key words
Polysaccharide nanoparticles; near-infrared fluorescence; nanoprobes; cancer; imaging
Introduction
Molecular imaging techniques are very powerful tools in early cancer detection, drug discovery and development as well as monitoring response to treatment by offering information about biological changes in living subjects (1,2). There are various types of imaging modalities, including positron-emission tomography (PET), single photon emission computed tomography (SPECT), magnetic resonance imaging (MRI) and optical fluorescence imaging. Recently, in vivo near-infrared fluorescence (NIRF) imaging techniques are most exciting for early detection of cancer in living organisms because biological tissues show very low absorption and autofluorescence in the NIR spectrum window (650-900 nm) (3).
As an emerging research area, nanobiotechnology offers abundant opportunities for discovering new materials and tools for biomedicine. Recently, most exciting and rapidly progressing area in nanobiotechnology is the development of nanoprobes capable of improving detection sensitivity and specificity (4). These nanoprobes could target tumors either through the ‘enhanced permeability and retention’ (EPR) effect of the hallmark of tumor microvasculatures or by the specific binding with tumor-associated biomarkers (5,6).
A variety of nanoprobes (i.e., quantum dots, polymer, gold, silica and paramagnetic nanoparticles) have been applied in biomedical applications. Among them, polymeric nanoparticles have been attractively used as both drug carriers and imaging agents. More specifically, the application of polysaccharides in biomedicine area is rapidly growing because of their biocompatibility, biodegradability, low toxicity, and low cost. In addition, versatile physicochemical properties and facile chemical modifications enable the preparation of a variety of nanosystems. This research highlight article focuses on the latest progress in polysaccharide-based NIRF nanoprobes. More specifically, we summarize the different targeting approaches and in vivo cancer imaging applications of polysaccharide-based NIRF nanoprobes.
Targeting approaches of nanoprobes
The key issue in cancer imaging is how to specifically deliver the adequate concentrations of nanoprobes at the target sites. To specifically deliver nanoprobes and achieve excellent detection efficiency, the ideal nanoprobes should be able to more preferentially reach the desired tumor lesion, and have also the ability to selectively detect cancerous cells without affecting normal cells. The delivery of nanoprobes to the intended sites can be achieved primarily by two targeting approaches such as passive and active targeting (Figure 1).
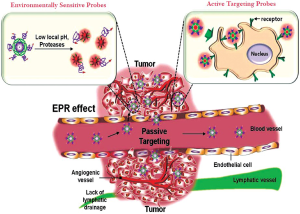
Passive targeting takes advantage of the inherent size of nanoprobes and the hallmarks of tumor vasculature (EPR effect) (5) and the tumor microenvironment. Figure 1 shows that rapidly growing tumors are characterized by extensive angiogenesis, defective vasculature architecture and the lack of tumor lymphatic drainage. Due to these features, nanoprobes can reach levels 10 to 100 times higher in tumor tissues in comparison with the small molecule drugs. Another passive targeting approach is the unique microenvironment surrounding tumors. In general, cancer cells highly over-express proteases that act important roles to tumor migration, invasion and metastasis (7). On the other hand, due to anaerobic metabolism of hyperproliferative cancer cells, cancer cells adapt to use glycolysis to obtain extra energy, resulting in an acidic environment. In this regards, the detection of biomolecules or specific environmental changes surrounding tumors are attractive targets for the development of nanoprobes (Figure 1). Nanoprobes have been targeted to sites of interest via a number of different affinity ligands. Active targeting is usually achieved by coupling the surface of nanoprobes with targeting moieties that specifically bind to surface epitopes or receptors, and thereby allowing more selectively delivering nanoprobes in the target sites. The addition of specific targeting moieties can facilitate far more sensitive cancer detection. Based on these targeting approaches, nanoprobes can achieve increased concentration and facilitate detection efficiency in cancer cells without damaging in normal cells.
Passively targeted polysaccharide-based NIRF nanoprobes
The unique property of nanoprobes to preferentially accumulate in tumor tissues provides a platform for improved cancer imaging. Due to safety, cost effectiveness, versatile physicochemical characteristics and facile modifications, polysaccharide-based nanoprobes have been of great interest as intrinsically targeted drug delivery carriers and imaging agents. By exploring the inherent EPR effect, the nanoprobes that are conjugated to NIR fluorophores (i.e, Cy5.5 and Cy7) have been used with great success in imaging cancerous tissue using various NIRF imaging modalities.
Glycol chitosan-based nanopaticles (GC NPs) have been extensively studied as NIRF nanoprobes for cancer diagnosis. Kwon’s group developed Cy5.5-labeled glycol chitosan-5ß cholanic acid nanoparticles (Cy5.5-GC NPs) with a diameter of 260±30 nm (Figure 2A and B) (8). In vivo NIRF images showed that Cy5.5-GC NPs showed prolonged blood circulation time and preferentially accumulate at tumor tissues compared to other organs (Figure 2C and D). They also verified that highly deformable Cy5.5-GC NPs can successfully pass through in vivo filtration systems in the liver or spleen, but rigid/ non-deformable polystyrene NPs do not (8). Moreover, due to positive surface charge and lipophilicity, Cy5.5- GC NPs can be rapidly internalized in the cytoplasm of cancer cells and accumulated in tumor tissues within five minutes after systemic administration. Taken together, due to these characteristics, Cy5.5-GC NPs may have the excellent tumor targeting characteristics. Based on these results, Kwon group suggested that Cy5.5-GC NPs can be usefully utilized to detect solid tumors or tumor-associated angiogenesis. Indeed, they demonstrated that Cy5.5-GC NPs as nanoprobes can be widely applied to detect other cancers (i.e, brain and liver, and metastatic cancer) (8). When Cy5.5-GC NPs were injected at 5 mg/kg into mice bearing U87 MG tumor, they preferentially accumulated in brain tumors due to partial breakage of the blood brain barrier structure by tumor growth (Figure 2E). Also, they can detect orthotopic liver tumor in living mice (Figure 2F). In vivo NIRF signal increased more significantly than normal mouse after systemic administration of Cy5.5-GC NPs into orthotopic liver tumors. Ex vivo images of livers showed the intense NIRF signal in right side, not in whole liver, significantly proving the targeted localization of Cy5.5-GC NPs in liver tumor (Figure 2F). As another example, Cy5.5- GC NPs (5 mg/kg) can detect metastatic tumor in the lung induced by red fluorescence protein-expressing B16F10 cells. The intense NIRF signal was detected in the entire lung. Interestingly, an unintended secondary metastatic tumor was also detected, as an RFP signal was observed on the left forefoot 2 weeks post-injection of RFP-B16F10 (Figure 2G and H). The whole body and ex vivo images revealed that a 2.6 mm second metastatic tumor driven from the first metastatic tumor was successfully found using Cy5.5-labeled GC NPs. These results suggest that Cy5.5- labeled GC NPs are useful imaging nanoprobes to detect small size metastatic tumors during tumor progression.
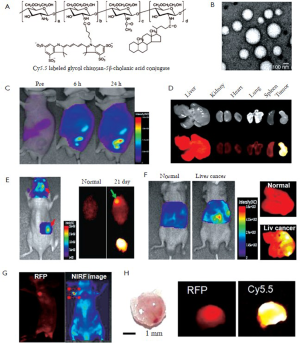
Environmentally sensitive NIRF nanoprobes
Despite the success of cancer imaging by fluorophorelabeled nanoprobes, one of issued concerns is limited tumor background ratio (TBR). To overcome this concern, more highly sensitive nanoprobes are required to more accurately detect biomolecules or specific environmental changes.
Proteases play key roles in various diseases such as cardiovascular diseases, cancers, and other inflammatory diseases. Because specific proteases are present at high levels in tumors and elevated at an early stage of tumor progression, they are attractive targets for the development of novel nanoprobes. Knowledge that certain protease selectively cleaves specific peptide substrates can be applied to achieve enzyme-sensitive nanoprobes (9). As one of a large number of proteases, MMPs are potentially useful targets for imaging or drug delivery in various diseases. Recently, Kim group developed MMP-sensitive NIRF nanoprobes consisting of a self-assembled GC NPs and activatable dark-quenched peptide-based probes (Figure 3A) (10). These MMP-activatable nanoprobes can deliver the probes effectively to tumor sites (i.e, flank tumor and colon cancer) by the EPR effect and have higher sensitivity because the peptide substrate-mediated fluorescence labeling of nanoparticles can be strongly dual-quenched by both the dye-dark quencher and NIR dye-dye selfquenching mechanisms (Figure 3A). After exposure to specific MMPs in tumor sites, NIR-dye substrate was degraded by the recognition of specific MMP and a pronounced NIRF signal recovery in tumor sites was observed due to dequenching of the dye (Figure 3B, C, and D). The nanoprobe can be applied for the detection of other proteases by simply replacing the specific peptide substrate. In similar way, they also developed cathepsin B-sensitive nanoprobe by conjugating GC NPs with cathepsin B-activatable dark-quenched peptide substrate (11). The cathepsin B-sensitive nanoprobe can also detect cancer and is more useful to evaluate the cytoplasmic targeting. The design of these nanoplatforms is flexible and finetunable for a wide array of applications such as detection of biomolecules, early diagnosis of disease, monitoring therapeutic efficacy, and theranostic nanoplatforms.
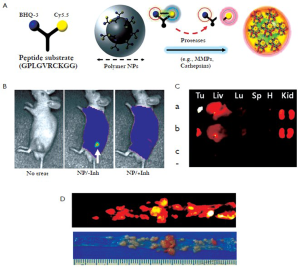
Hypoxia in the tumor microenvironment results in lactic acid production and hence acidic conditions. Indeed, solid tumors with pH ranging from 5.8 to 7.7 are on average 0.5 units lower than the pH of normal tissue. Thus, the use of different pH environments has been a promising avenue for cancer imaging and therapy. Recently, Park et al. developed novel imaging and therapeutic system that quickly switched into an aggressive molecule for tumor imaging and destruction within the acidic environment of tumor (12). The pH-sensitive system consisted of GC backbone, DEAP (pH sensitive moiety), Ce6 (photosensitizer), and PEG. It exhibited an intelligent switch from a self-assembly (i.e., self-quenched state of photosensitizer) into extended random molecules (i.e., dequenched state for singlet oxygen production), which corresponds to a change in surface charge at the extracellular acidic pH. At physiological pH 7.4, it showed negligible fluorescence signal and singlet-oxygen production as well as no noticeable cell apoptosis. However, at extracellular acidic pH (pH 6.8 or 6.4), it becomes disentangled and reached to the dequenched state, and thereby producing significant NIRF signal and singlet-oxygen generation allowing higher phototoxicity for HeLa cells. This smart system will be expected to simultaneously provide targeted cancer imaging and high-dose cancer therapy.
Actively targeted polysaccharide-based NIRF probes
To more improve cancer detection efficiency, nanoprobes are usually functionalized with targeting moieties that can specifically recognize and interact with target molecules produced by cancer cells. In addition, it allows for targeting of the nanoprobes to much smaller and earlier stage tumors, as well as to cancerous cells.
Through phage display screening, Lee group discovered an interleukin-4 receptor-binding peptide (termed the I4R peptide), which can selectively bind to IL-4R (13). Recently, they developed the I4R peptide-guided Cy5.5- GC NPs for the detection of IL-4R over-expressed in human cancer cells (13). Through in vitro binding study, cellular uptake, and in vivo tumor imaging studies, they showed consistently that I4R-conjugated GC NPs have high binding affinity, cellular uptake and tumor imaging ability with respect to IL-4R expression levels of cancer cells.
Hyaluronan (HA)-bsed nanoprobes are also of great interest as imaging agents. Because HA itself is the major ligand for CD44 and CD168 (14), HA-based nanoprobes are suitable for the targeting CD44 and CD168-expressing cells. Choi et al., developed HA-based nanoprobes that were modified with the 5ß-cholanic acid to form nanoprobes that combine both passive tumor targeting based on the EPR effect and active targeting towards HA receptors over-expressing tumors (Figure 4A) (15). Cy5.5-labeled- HA nanoprobes could detect CD44 over-expressing cells (SCC7) to a much greater extent than CV-1 cells with low CD44 expression. The HA-based nanoprobes selectively accumulate in SCC7 tumor tissues in vivo (Figure 4B). In addition, the concentration of the NPs in the tumor site was dramatically reduced when mice were pretreated with free HA. This suggests an additional active targeting mechanism, beyond the passive targeting of the EPR effect.
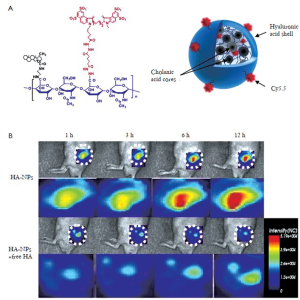
Conclusions
This article discusses recent progress of the development of polysaccharide-based imaging nanoprobes. Until now, polysaccharide-based NIRF imaging nanoprobes have not been much extensively studied in comparison with synthetic polymer- or metallic-based imaging nanoprobes. Although not much described in this article, it is clear that polysaccharide-based imaging nanoprobes are an emerging and potent instrument in cancer diagnosis because they are ‘biologically friendly’ carrier systems and will overcome many of the toxicity and accumulation concerns of other nanoagents. Furthermore, one of the greatest advantages is that most of polysaccharide-based nanoprobes used to target imaging and diagnostic agents can also be applied as therapeutic drugs to treat cancer. These theranostic (therapeutic and diagnostic) nanoplatforms allow for the determination of the localization, release, and their therapeutic efficacies and may benefit in the near future for the enhanced diagnosis and treatment of cancers.
Acknowledgements
This study was supported by KBSI grant (T32513) to KP.
Disclosure: The author declares no conflict of interest.
References
- Weissleder R. Molecular imaging in cancer. Science 2006;312:1168-71.
- Weissleder R, Pittet MJ. Imaging in the era of molecular oncolocy. Nature 2008;452:580-9.
- Ballou B, Ernst LA, Waggoner AS. Fluorescence imaging of tumors in vivo. Curr Med Chem 2005;12:795-805.
- Minchin RF, Martin DJ. Nanoparticles for molecular imaging: an overview. Endocrinology 2010;151:474-81.
- Maeda H, Wu J, Sawa T, et al. Tumor vascular permeability and the EPR effect in macromolecular therapeutics: a review. J Control Release 2000;65:271-84.
- Kong G, Braun RD, Dewhirst MW. Hyperthermia enables tumor-specific nanoparticle delivery: effect of particle size. Cancer Res 2000;60:4440-5.
- López-Otín C, Hunter T. The regulatory crosstalk between kinases and proteases in cancer. Nat Rev Cancer 2010;10:278-92.
- Na JH, Koo H, Lee S, et al. Real-time and non-invasive optical imaging of tumor-targeting glycol chitosan nanoparticles in various tumor models. Biomaterials 2011:32:5252-61.
- Weissleder R, Tung CH, Mahmood U, et al. In vivo imaging of tumors with protease-activated near-infraredfluorescent probes. Nat Biotechnol 1999;17:375-8.
- Lee S, Ryu JH, Park K, et al. Polymeric nanoparticlebased activatable near-infrared nanosensor for protease determination in vivo. Nano Lett 2009;9:4412-6.
- Yhee Jy, Kim SA, Koo H, et al. Optical imaging of cancerrelated proteases using near-infrared fluorescence matrix metalloproteinase-sensitive and cathepsin B-sensitive probes. Theranostics 2012;2:179-89.
- Park SY, Baik HJ, Oh YT, et al. A smart polysaccharide/ drug conjugate for photodynamic therapy. Angew Chem Int Ed Engl 2011;50:1644-7.
- Kim JH, Bae SM, Na MH, et al. Facilitated intracellular delivery of peptide-guided nanoparticles in tumor tissues. J Control Release 2012;157:493-9.
- Platt VM, Szoka FC Jr. Anticancer therapeutics: targeting macromolecules and nanocarriers to hyaluronan or CD44, a hyaluronan receptor. Mol Pharm 2008;5:474-86.
- Choi KY, Chung H, Min KH, et al. Self-assembled hyaluronic acid nanoparticles for active tumor targeting. Biomaterials 2010;31:106-14.