Characterization of 18F-fluorodeoxyglucose metabolic spatial distribution improves the differential diagnosis of indeterminate pulmonary nodules and masses with high fluorodeoxyglucose uptake
Introduction
With the increase in lung cancer screening and the growing global aging population, the incidence of lung cancer is increasing rapidly. Lung cancer has become the leading cause of cancer-associated death in the United States (1). Early detection strategies and surgical resection are the key to reducing lung cancer mortality (2).
Positron emission tomography/computed tomography (PET/CT), a noninvasive imaging technique, is widely used in the diagnosis and staging of pulmonary nodules (3,4). The maximum standard uptake value (SUVmax) is a key semiquantitative parameter for PET that measures the metabolic activity of a lesion. An SUVmax of 2.5 is usually used as the cut-off value for differentiating malignant from benign pulmonary lesions. However, 18F-fluorodeoxyglucose (18F-FDG) is not a tumor-specific agent; thus, intense FDG uptake can be observed in a significant percentage of benign pulmonary lesions, including inflammatory pseudotumors, cryptococcosis, and tuberculosis (5-7). Previous studies on the diagnosis of pulmonary lesions have reported high false positive rates associated with SUV (8,9). In particular, when the morphological characteristics of benign and malignant lesions are atypical, the false positive rate is higher. The high rates of false positive results have seen a corresponding increase in the rates of unnecessary invasive lung surgery and associated morbidity (10).
Therefore, a novel, alternative PET/CT-derived method is urgently needed to compensate for the high false positive rates resulting from the SUV method. In tumor development and progression, cancer cells migrate to and invade blood vessels (11); therefore, it can be speculated that cell metabolic activity will be higher in the proximal part of malignant tumors than in the distal part. Our previous findings have confirmed that the relative activity distribution (RAD) of 18F-FDG in malignant and benign solitary pulmonary nodules (SPNs) are markedly different. Furthermore, the use of SPN characterization with 18F-FDG RAD was much more specific than conventional SUV diagnostic methods in differentiating malignant from benign SPNs (12). However, the previous study only analyzed local metabolic distribution on small pulmonary nodules, and data needed to be obtained by performing complex diameter measurements and calculations. Therefore, the clinical feasibility of 18F-FDG metabolic spatial distribution (FMSD) in differentiating pulmonary nodules requires a wider range of samples and further simplification.
Therefore, the aim of the present study was to evaluate whether visual assessment of 18F-FDG metabolic spatial distribution (V-FMSD), an easy-to-operate method, could improve the differential diagnosis of hypermetabolic pulmonary nodules and masses.
Methods
Patients
This retrospective study included 301 consecutive subjects (186 men and 115 women; mean age, 61.6±11.2 years; range, 21–89 years) who underwent FDG PET/ CT for suspected malignant pulmonary nodules or masses between January 1, 2015 and April 26, 2017. The exclusion criteria were as follows: (I) 18F-FDG SUVmax of the lesion <2.5; (II) typical benign signs (central laminated or diffuse calcification, or popcorn pattern of calcification) or malignant signs (any three of the following: densely spiculated margin, vacuole sign, air bronchogram, vessel convergence, pleural indentation, and lesion with cavitation and wall thickness >16 mm) detected by chest CT; (III) metastatic lesions or evidence of metastasis; (IV) multiple hypermetabolic nodules or masses in both lungs; (V) a previous history of lung biopsy or neoadjuvant chemoradiotherapy; (VI) a previous history of other cancer; (VII) obvious motion artifacts.
The pathological specimens were obtained by fiber optic bronchoscopy, percutaneous biopsy, resection by thoracotomy, or video-assisted thoracoscopic surgery after PET/CT examination. All malignant lesions were finally confirmed by pathology, and a definitive diagnosis of a benign lesion was made based on pathological analysis or at least 2 years of follow-up by chest CT. This study was approved by the Institutional Ethics Committee of the First Affiliated Hospital of Wenzhou Medical University, and written informed consent was obtained from all participants.
PET/CT acquisition
Patients fasted for at least 6 hours, and their serum glucose levels were <110 mg/dL before intravenous injection of 18F-FDG (3.7 MBq/kg). Approximately 60 minutes after the injection, images were acquired using a hybrid PET/CT scanner (GEMINI TF 64, Philips, Best, Netherlands). The detailed PET/CT protocol can be found in our previous published study (13). The dual time point scans of the pulmonary lesions were performed 120 minutes after the injection. PET images were reconstructed using the ordered subset expectation maximization (OSEM) method (33 subsets per iteration). All collected data were transferred onto the Philips Extend Brilliance Workstation (EBW, Philips, Best, Netherlands) 3.0 for reconstruction of PET, CT, and PET/CT fusion images.
Image analysis
Data measurement was independently performed by a nuclear medicine physician with 3 years of experience in PET/CT diagnosis. For each lesion, the SUVmax values of early and delayed scans were quantified. The retention index (RI) was calculated according to the following formula:
[1]
In the assessment of SUV, the findings were considered positive when SUVmax ≥2.5, and for delayed PET scans, RI > 0 was defined as positive.
The combined PET/CT images were independently assessed by two radiologists, each with over 30 years’ experience in radiological diagnosis, who were blinded to the FMSD characteristics of the lesions. First, definitely positive findings were considered when 18F-FDG uptake in lesions was significantly higher than that in the mediastinal blood pool. Second, for lesions with a slightly high uptake of 18F-FDG, the morphological and metabolic lesion characteristics were combined to draw a comprehensive diagnosis. The diagnostic results were divided into probably positive or probably negative. When the findings were inconsistent between the two readers, a consensus was reached by discussion. Finally, definitely or possibly positive lesions were recorded as positive, and possibly negative lesions were recorded as negative.
The V-FMSD was analyzed by two nuclear medicine physicians each with 10 years of experience in PET/CT diagnosis, who were blinded to the information of the CT images and the final diagnoses of the patients. The proximal region of the lesion was defined as the area near the ipsilateral hilar, and the area far from the ipsilateral hilar was defined as the distal region (14). A 5-point scoring system was used to interpret the characteristics of FMSD, which represent the likelihood of the lesion being benign or malignant (Table 1, Figure 1). When the characteristics of FMSD in lesions were not obvious, the metabolic threshold could be manually adjusted on transverse PET images to assist the interpretation. When the interpretation scores were inconsistent between the two readers, a consensus was reached by discussion. Finally, a score of ≤2 was recorded as negative, while a score of ≥3 was recorded as positive.
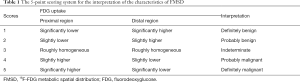
Full table
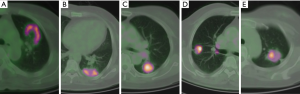
Statistical analysis
Statistical analyses were performed with SPSS software, version 23.0 (IBM Corp., Armonk, New York, USA). The Mann–Whitney U-test was used to evaluate the continuous variables between malignant and benign lesions, and the results were described as mean ± standard deviation. The Chi-squared test was performed to analyze the categorical data, and the results were presented as frequencies and percentages. Receiver operating characteristic (ROC) curve analysis was used to assess the performance of SUVmax, RI, combined PET/CT assessment, and V-FMSD for distinguishing malignant and benign lesions. The sensitivity, specificity, accuracy, positive predictive value (PPV), and negative predictive value (NPV) for each diagnostic method were also calculated. McNemar’s test was used to compare the sensitivity, specificity, and accuracy of V-FMSD with those of the other methods. The intra-observer variability in visual scores was determined using Cohen’s kappa coefficient (κ). A P value of <0.05 was considered to be statistically significant.
Results
General characteristic of subjects
Of 301 nodules and masses, 194 (64.5%) were malignant, and 107 (35.5%) were benign. The average maximum diameter of the lesions was 27.7±13.2 mm (range, 8–63 mm). The malignant lesions were pathologically confirmed as adenocarcinoma (129, 66.5%), squamous cell carcinoma (41, 21.1%), adenosquamous carcinoma (13, 6.7%), small cell carcinoma (4, 2.1%), neuroendocrine carcinoma (4, 2.1%), and sarcomatoid carcinoma (3, 1.6%). The benign lesions, confirmed by pathology or follow-up, included tuberculosis (45, 42.1%), cryptococcal disease (18, 16.8%), chronic inflammation (12, 11.2%), hemangioma (9, 8.4%), focal organizing pneumonia (8, 7.5%), granulomatous inflammation (6, 5.6%), inflammatory pseudotumor (3, 2.8%), interstitial pneumonia (2, 1.9%), fungal infection (2, 1.9%), angiofollicular hyperplasia (1, 0.9%), and sclerotic silicotic nodule (1, 0.9%). The general characteristics of benign and malignant lesions are shown in Table 2. Compared with benign lesions, malignant tumors presented with significantly higher values for lesion size, SUVmax, delayed SUVmax, and RI.
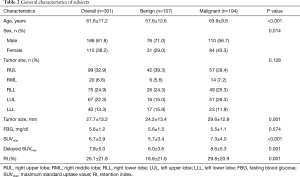
Full table
The V-FMSD scoring results
The visual scoring results of FMSD in the benign and malignant lesions are shown in Table 3. There were 180 (92.8%) malignant lesions with a score of ≥3, which indicated that FDG metabolic distribution in the proximal region of the malignant lesions was significantly higher than that in the distal region (Figure 2). There were 78 (72.9%) benign lesions with a score of ≤2, which indicated that FDG metabolic distribution in the proximal region of benign lesions was significantly lower than that in the distal region (Figure 3). The intra-observer variability in visual scores by the two observers is shown in Table 4. The Cohen’s kappa coefficient demonstrated good agreement between the two observers’ visual scores, with a kappa value of 0.635.

Full table

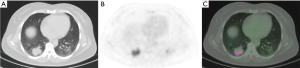
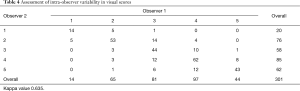
Full table
The diagnostic performance of different methods
The performance of V-FMSD for distinguishing malignant from benign lesions is shown in Table 5. When V-FMSD score ≥3 was used as the cut-off value, the sensitivity, specificity, PPV, NPV, and diagnostic accuracy of V-FMSD were 92.8%, 72.9%, 85.7%, 86.1%, and 89.2%, respectively. The diagnostic performance of V-FMSD showed no significant difference in distinguishing malignant and benign lesions in nodules of different sizes (all P>0.05). Although the sensitivity of V-FMSD did not differ significantly from those of the other three methods (all P>0.05), its specificity (72.9%) was significantly higher than those of SUVmax (0%, P<0.001) and RI (26.2%, P<0.001) (Table 6).
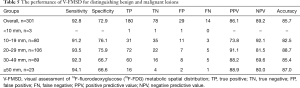
Full table
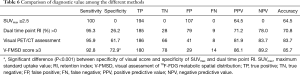
Full table
The ROC curves for the different methods in the diagnosis of malignant and benign lesions are shown in Figure 4. The areas under the ROC curves (AUCs) of SUVmax, RI, combined PET/CT assessment, and V-FMSD were 0.626, 0.670, 0.788, and 0.886, respectively. The AUC of V-FMSD was significantly larger than those of SUVmax, RI, and combined PET/CT (all P<0.05) (Table 7).
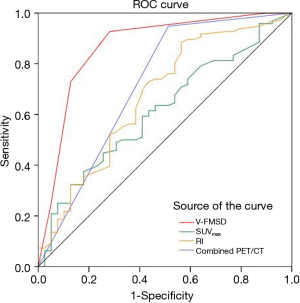

Full table
Discussion
The present study firstly evaluated the performance of V-FMSD for distinguishing pulmonary malignant and benign lesions. The results indicated that FDG uptake in the proximal region of malignant lesions was significantly higher than that in the distal region, and the FMSD of benign lesions was opposite to that of malignant lesions. Furthermore, FMSD demonstrated higher diagnostic efficacy than SUVmax and RI in the diagnosis of pulmonary hypermetabolic lesions, especially in terms of specificity. Therefore, this method should be considered as a valuable investigative addition in the assessment of indeterminate pulmonary lesions.
18F-FDG PET/CT is a well-established imaging modality for the diagnosis of patients with suspected lung cancer. However, the overlap of SUVmax between benign and malignant pulmonary lesions limits PET/CT diagnostic value. Previous studies have reported the specificity of PET for the diagnosis of malignant pulmonary nodules to vary between 13% and 89% (15-19). Inflammatory lesions have been shown to account for 73% of benign non-neoplastic lesions with increased 18F-FDG uptake (20). The 18F-FDG uptake which represents the levels of cellular glucose metabolism in inflammatory and oncological diseases shares the similar mechanism and is mediated by the glucose transporters GLUT1–5 (21,22). In active inflammation and granulomatous diseases, such as tuberculosis and sarcoidosis, increased 18F-FDG accumulation appears in cells due to the expression of glucose transporters, especially GLUT1 in activated leucocytes, macrophages, and T lymphocytes (23-25).
Since the prevalence of tuberculosis in China is higher than in Western countries, the accuracy of 18F-FDG PET/CT for the identification of pulmonary abnormalities may be decreased. In the present study, almost half (42.1%) of benign lesions were tuberculosis, and the average SUVmax of those tubercular lesions was 6.7. All of the cases included in this study had hypermetabolic lesions with an SUVmax >2.5. When SUVmax 2.5 was used as the cut-off value to diagnose lung cancer, the number of false positive cases was 107, and the specificity and accuracy were 0% and 64.5%, respectively. This showed that the value of SUVmax in the diagnosis of hypermetabolic lung nodules and masses was extremely limited. PET/CT combines lesion morphology and metabolic information. However, for hypermetabolic pulmonary nodules and masses with atypical CT morphology, the diagnostic basis is more dependent on the metabolic information of the lesion. In this study, the number of false positive cases with PET/CT combined analysis was 41, of which tuberculosis and cryptococcal bacteria accounted for a large proportion. Therefore, to further improve diagnostic accuracy for hypermetabolic nodules and masses, it is necessary to explore novel auxiliary analysis methods.
Our previous study confirmed the difference in RAD of 18F-FDG between malignant and benign SPNs (12). To further simplify the evaluation, the present study used the hilar as a reference for the division of lesions into proximal and distal regions, and visually interpreted the spatial distribution of 18F-FDG uptake in these two regions of benign and malignant lesions. The results of this study showed that in the malignant lesions, the 18F-FDG uptake in the proximal region was significantly higher than that of the distal region. In benign lesions, the spatial distribution of 18F-FDG was opposite to that in malignant lesions, with the 18F-FDG uptake lower in the proximal region than in the distal region. In regard to the pathological mechanisms underlying the metabolic spatial heterogeneity between benign and malignant lesions, one of the possible reasons may be related to angiogenesis and its distribution. Recent studies have indicated that angiogenesis plays an important role in tumor formation, growth, metastasis, and recurrence (26,27). Abnormal tumor vasculature may lead to altered perfusion, which is believed to be the major extrinsic driver of metabolism through its effects on oxygen and substrate delivery (28). Also, the main blood supply for lung tumors comes from the bronchial artery, while inflammation is more likely to stimulate the extrapulmonary circulation artery to participate in blood supply. Therefore, the blood supply in the proximal region of lung tumors is more abundant, and the same phenomenon can be observed in the distal region of lung inflammation. However, the metabolic distribution in a lesion is influenced by many factors, including the tumor microenvironment, genetic heterogeneity, and immune factors (29-31).
The hallmarks of the V-FMSD method are its simplicity and easy operability in clinic. Furthermore, this simple visual analysis can achieve a high diagnostic performance. Although the sensitivity of V-FMSD was similar to those of the PET/CT conventional markers, the specificity of this new method was significantly higher than those of SUVmax and RI. However, the visual difference in metabolic distribution in small nodules was not obvious due to their small volume, especially for nodules <10 mm in size. However, the diagnostic performance of V-FMSD for nodules of different sizes (>10 mm) showed no statistical significance in distinguishing between malignant and benign lesions. The pathologies of the 14 malignant nodules with FMSD grade 2 were adenocarcinoma (9, 64.3%), squamous cell carcinoma (2, 14.3%), adenosquamous carcinoma (2, 14.3%), and small cell carcinoma (1, 7.1%), respectively. Of the 29 cases of benign nodules with FMSD grade 3–5, almost half were tuberculosis (13, 44.8%). Compared with the findings of previous studies, our results still showed the conventional PET/CT markers to have lower sensitivity and specificity (3,32,33). The major reason for this might be the high proportion of benign hypermetabolic lesions included in this study. However, this novel visual assessment can still improve the diagnostic performance when the CT morphology is atypical. Therefore, V-FMSD can be considered a novel routine auxiliary diagnostic method for the diagnosis of indeterminate pulmonary nodules and masses with high 18F-FDG uptake.
The main limitation of this study is that visual analysis is a subjective empirical judgment which may introduce subjective bias. In the present study, the Kappa value for intra-observer agreement was 0.635, which suggested that consistency could be further improved. In clinical practice, multiple comparative analysis of FMSD by adjusting the SUV threshold can be used to improve the repeatability of the assessment. In patient selection, we only included pulmonary nodules and masses with avid 18F-FDG uptake, which might have introduced selection bias. Moreover, this study did not explore the related molecular mechanisms. However, our pre-experiments showed that the expression levels of CD31 and epidermal growth factor receptor (EGFR) were different between the proximal and distal regions of benign and malignant lung lesions, which suggested that the difference in FMSD may be correlated with tumor angiogenesis and its distribution. Therefore, future studies will explore the possible related mechanisms.
Conclusions
In summary, we found that pulmonary benign and malignant lesions had distinct FMSD characteristics. FDG uptake in the proximal region of malignant lesions was significantly higher than that in the distal region, and the FMSD of benign lesions was opposite to that of malignant lesions. V-FMSD is a simple and easy procedure; therefore, it can be used as a novel auxiliary marker in clinical practice to improve the diagnostic accuracy for indeterminate pulmonary nodules and masses with avid 18F-FDG uptake.
Acknowledgments
Funding: This work was supported by Zhejiang Province Public Welfare Technology Application Research Project (No. LGF19H180012) and Wenzhou Science and Technology Program (No. Y20170833).
Footnote
Conflicts of Interest: All authors have completed the ICMJE uniform disclosure form (available at http://dx.doi.org/10.21037/qims-20-768). The authors have no conflicts of interest to declare.
Ethical Statement: This study was approved by the Institutional Ethics Committee of the First Affiliated Hospital of Wenzhou Medical University. Written informed consent was obtained from all patients.
Open Access Statement: This is an Open Access article distributed in accordance with the Creative Commons Attribution-NonCommercial-NoDerivs 4.0 International License (CC BY-NC-ND 4.0), which permits the non-commercial replication and distribution of the article with the strict proviso that no changes or edits are made and the original work is properly cited (including links to both the formal publication through the relevant DOI and the license). See: https://creativecommons.org/licenses/by-nc-nd/4.0/.
References
- Siegel R, Naishadham D, Jemal A. Cancer statistics, 2012. CA Cancer J Clin 2012;62:10-29. [Crossref] [PubMed]
- Patz EF Jr, Rossi S, Harpole DH Jr, Herndon JE, Goodman PC. Correlation of tumor size and survival in patients with stage IA non-small cell lung cancer. Chest 2000;117:1568-71. [Crossref] [PubMed]
- Garcia-Velloso MJ, Bastarrika G, de-Torres JP, Lozano MD, Sanchez-Salcedo P, Sancho L, Nunez-Cordoba JM, Campo A, Alcaide AB, Torre W, Richter JA, Zulueta JJ. Assessment of indeterminate pulmonary nodules detected in lung cancer screening: Diagnostic accuracy of FDG PET/CT. Lung cancer (Amsterdam, Netherlands) 2016;97:81-6. [Crossref] [PubMed]
- Zhang Y, Ni J, Wei K, Tian J, Sun S. CT. MRI, and F-18 FDG PET for the detection of non-small-cell lung cancer (NSCLC): A protocol for a network meta-analysis of diagnostic test accuracy. Medicine (Baltimore) 2018;97:e12387. [Crossref] [PubMed]
- Sánchez-Montalvá A, Barios M, Salvador F, Villar A, Tórtola T, Molina-Morant D, Lorenzo-Bosquet C, Espinosa-Pereiro J, Molina I. Usefulness of FDG PET/CT in the management of tuberculosis. PLoS One 2019;14:e0221516. [Crossref] [PubMed]
- Chang JM, Lee HJ, Goo JM, Lee HY, Lee JJ, Chung JK, Im JG. False positive and false negative FDG-PET scans in various thoracic diseases. Korean J Radiol 2006;7:57-69. [Crossref] [PubMed]
- Yu WY, Lu PX, Assadi M, Huang XL, Skrahin A, Rosenthal A, Gabrielian A, Tartakovsky M, Wang YXJ. Updates on (18)F-FDG-PET/CT as a clinical tool for tuberculosis evaluation and therapeutic monitoring. Quant Imaging Med Surg 2019;9:1132-46. [Crossref] [PubMed]
- Feng M, Yang X, Ma Q, He Y. Retrospective analysis for the false positive diagnosis of PET-CT scan in lung cancer patients. Medicine (Baltimore) 2017;96:e7415. [Crossref] [PubMed]
- Maiga AW, Deppen SA, Mercaldo SF, Blume JD, Montgomery C, Vaszar LT, Williamson C, Isbell JM, Rickman OB, Pinkerman R, Lambright ES, Nesbitt JC, Grogan EL. Assessment of Fluorodeoxyglucose F18-Labeled Positron Emission Tomography for Diagnosis of High-Risk Lung Nodules. JAMA Surg 2018;153:329-34. [Crossref] [PubMed]
- Grogan EL, Deppen SA, Ballman KV, Andrade GM, Verdial FC, Aldrich MC, Chen CL, Decker PA, Harpole DH, Cerfolio RJ, Keenan RJ, Jones DR, D'Amico TA, Shrager JB, Meyers BF, Putnam JB Jr. Accuracy of fluorodeoxyglucose-positron emission tomography within the clinical practice of the American College of Surgeons Oncology Group Z4031 trial to diagnose clinical stage I non-small cell lung cancer. Ann Thorac Surg 2014;97:1142-8. [Crossref] [PubMed]
- Wu PH, Giri A, Sun SX, Wirtz D. Three-dimensional cell migration does not follow a random walk. Proc Natl Acad Sci U S A 2014;111:3949-54. [Crossref] [PubMed]
- Zhao L, Tong L, Lin J, Tang K, Zheng S, Li W, Cheng D, Yin W, Zheng X. Characterization of solitary pulmonary nodules with 18F-FDG PET/CT relative activity distribution analysis. Eur Radiol 2015;25:1837-44. [Crossref] [PubMed]
- Xu M, Wang L, Ouyang M, Lin J, Wang L, Zheng X, Miao S, Tang K. Prediction of lymph node metastasis by PET/CT metabolic parameters in patients with esophageal squamous cell carcinoma. Nucl Med Commun 2019;40:933-9. [Crossref] [PubMed]
- Wu L, Cao G, Zhao L, Tang K, Lin J, Miao S, Lin T, Sun J, Zheng X., Spectral CT. Analysis of Solitary Pulmonary Nodules for Differentiating Malignancy from Benignancy: The Value of Iodine Concentration Spatial Distribution Difference. Biomed Res Int 2018;2018:4830659. [Crossref] [PubMed]
- van Gómez López O, García Vicente AM, Honguero Martínez AF, Jiménez Londoño GA, Vega Caicedo CH, León Atance P, Soriano Castrejón ÁM. (18)F-FDG-PET/CT in the assessment of pulmonary solitary nodules: comparison of different analysis methods and risk variables in the prediction of malignancy. Transl Lung Cancer Res 2015;4:228-35. [PubMed]
- Li Y, Su M, Li F, Kuang A, Tian R. The value of (1)(8)F-FDG-PET/CT in the differential diagnosis of solitary pulmonary nodules in areas with a high incidence of tuberculosis. Ann Nucl Med 2011;25:804-11. [Crossref] [PubMed]
- Hashimoto Y, Tsujikawa T, Kondo C, Maki M, Momose M, Nagai A, Ohnuki T, Nishikawa T, Kusakabe K. Accuracy of PET for diagnosis of solid pulmonary lesions with 18F-FDG uptake below the standardized uptake value of 2.5. J Nucl Med 2006;47:426-31. [PubMed]
- Taralli S, Scolozzi V, Foti M, Ricciardi S, Forcione AR, Cardillo G, Calcagni ML. (18)F-FDG PET/CT diagnostic performance in solitary and multiple pulmonary nodules detected in patients with previous cancer history: reports of 182 nodules. Eur J Nucl Med Mol Imaging 2019;46:429-36. [Crossref] [PubMed]
- Matthies A, Hickeson M, Cuchiara A, Alavi A. Dual time point 18F-FDG PET for the evaluation of pulmonary nodules. J Nucl Med 2002;43:871-5. [PubMed]
- Metser U, Even-Sapir E. Increased (18)F-fluorodeoxyglucose uptake in benign, nonphysiologic lesions found on whole-body positron emission tomography/computed tomography (PET/CT): accumulated data from four years of experience with PET/CT. Semin Nucl Med 2007;37:206-22. [Crossref] [PubMed]
- Brown RS, Leung JY, Kison PV, Zasadny KR, Flint A, Wahl RL. Glucose transporters and FDG uptake in untreated primary human non-small cell lung cancer. J Nucl Med 1999;40:556-65. [PubMed]
- Rivlin M, Navon G. Molecular imaging of tumors by chemical exchange saturation transfer MRI of glucose analogs. Quant Imaging Med Surg 2019;9:1731-46. [Crossref] [PubMed]
- Huber H, Hodolic M, Stelzmuller I, Wunn R, Hatzl M, Fellner F, Lamprecht B, Rubello D, Colletti PM, Gabriel M. Malignant disease as an incidental finding at F-18-FDG-PET/CT scanning in patients with granulomatous lung disease. Nucl Med Commun 2015;36:430-7. [Crossref] [PubMed]
- Mochizuki T, Tsukamoto E, Kuge Y, Kanegae K, Zhao S, Hikosaka K, Hosokawa M, Kohanawa M, Tamaki N. FDG uptake and glucose transporter subtype expressions in experimental tumor and inflammation models. J Nucl Med 2001;42:1551-5. [PubMed]
- Keijsers RG, van den Heuvel DA, Grutters JC. Imaging the inflammatory activity of sarcoidosis. Eur Respir J 2013;41:743-51. [Crossref] [PubMed]
- Herbst RS, Onn A, Sandler A. Angiogenesis and lung cancer: prognostic and therapeutic implications. J Clin Oncol 2005;23:3243-56. [Crossref] [PubMed]
- Folkman J. Angiogenesis in cancer, vascular, rheumatoid and other disease. Nat Med 1995;1:27-31. [Crossref] [PubMed]
- Guillaumond F, Leca J, Olivares O, Lavaut MN, Vidal N, Berthezene P, Dusetti NJ, Loncle C, Calvo E, Turrini O, Iovanna JL, Tomasini R, Vasseur S. Strengthened glycolysis under hypoxia supports tumor symbiosis and hexosamine biosynthesis in pancreatic adenocarcinoma. Proc Natl Acad Sci U S A 2013;110:3919-24. [Crossref] [PubMed]
- Navin N, Kendall J, Troge J, Andrews P, Rodgers L, McIndoo J, Cook K, Stepansky A, Levy D, Esposito D, Muthuswamy L, Krasnitz A, McCombie WR, Hicks J, Wigler M. Tumour evolution inferred by single-cell sequencing. Nature 2011;472:90-4. [Crossref] [PubMed]
- Hensley CT, Faubert B, Yuan Q, Lev-Cohain N, Jin E, Kim J, Jiang L, Ko B, Skelton R, Loudat L, Wodzak M, Klimko C, McMillan E, Butt Y, Ni M, Oliver D, Torrealba J, Malloy CR, Kernstine K, Lenkinski RE, DeBerardinis RJ. Metabolic Heterogeneity in Human Lung Tumors. Cell 2016;164:681-94. [Crossref] [PubMed]
- Wang L, Zhu B, Zhang M, Wang X. Roles of immune microenvironment heterogeneity in therapy-associated biomarkers in lung cancer. Semin Cell Dev Biol 2017;64:90-7. [Crossref] [PubMed]
- Gould MK, Maclean CC, Kuschner WG, Rydzak CE, Owens DK. Accuracy of positron emission tomography for diagnosis of pulmonary nodules and mass lesions: a meta-analysis. JAMA 2001;285:914-24. [Crossref] [PubMed]
- Graeber GM, Gupta NC, Murray GF. Positron emission tomographic imaging with fluorodeoxyglucose isefficacious in evaluating malignant pulmonary disease. J Thorac Cardiovasc Surg 1999;117:719-27. [Crossref] [PubMed]