White matter in temporal lobe epilepsy: clinico-pathological correlates of water diffusion abnormalities
Introduction
One goal of neuroimaging in epilepsy is to identify focal lesions that are responsible for the disorder, with the hope that it can be surgically resected, rendering the patient seizure-free. Temporal lobe epilepsy (TLE), the most common form of focal epilepsy (1), is often accompanied by mesial temporal lobe sclerosis (MTS), a particular lesion of the hippocampus, amygdala, and entorhinal cortex characterized by specific patterns of neuronal loss and gliosis (2). The identification of an epileptogenic lesion such as MTS considerably improves the chances of seizure freedom following surgery (3). However, this specific lesion (identified in routine magnetic resonance imaging (MRI) as hippocampal atrophy, T2 signal hyperintensity, or both) is seen in only 25% of outpatients in a hospital-based setting (4), and in around 70% of TLE patients referred to a tertiary care center (5-8). For these reasons, there have been great efforts to improve neuroimaging techniques such that subtle lesions become apparent and surgery can be considered.
Having revolutionized the field of stroke, diffusion-weighted magnetic resonance imaging (dMRI) entered the field of epilepsy, with many hoping it would shed light on epileptogenic lesions and aid treatment decisions. While in some cases dMRI can accomplish this goal (9-12), it also showed that TLE patients have white matter abnormalities in several brain regions within and beyond the temporal lobe. Suddenly, TLE ceased to be a focal disorder with a clear unilateral lesion; these findings force us to conclude that TLE is truly a brain network disorder, and that the classically recognized MTS lesion is accompanied by micro-architectural abnormalities of several fiber bundles that interconnect remote brain areas.
In highly organized tissue such as white matter, the membranes and overall coherence of biological structures modulate the behavior of diffusing water molecules, and thus impart a particular signature to their displacement (13). In voxels occupied by a single fiber population, the resulting preferred direction of diffusion corresponds to the orientation of the fibers therein, which can be used to reconstruct the trajectories of macroscopic fiber tracts using tractography (14). Thus, dMRI is able to simultaneously provide information regarding macroscopic brain connectivity and microscopic features of the underlying tissue. There are numerous methods to analyze the behavior of water diffusion, but diffusion tensor imaging (DTI) (15) has been, by far, the most widely used for the study of TLE due to its relatively low demands in terms of data acquisition (which is generally performed in a clinical setting and therefore limited in time), but also due to its seemingly straightforward biological interpretation. In the tensor model, the diffusion profile is portrayed as an ellipsoid; its long axis (eigenvalue) is oriented parallel to the direction of fibers, and its magnitude relative to the two orthogonal eigenvalues provides a measure of anisotropy (i.e., the degree of directionality of diffusion imposed by the tissue). Analysis of the spatial profile of the diffusion of water, such as the shape of the tensor, provides important information that can be intuitively related to tissue characteristics. For instance, the largest eigenvalue is generally thought to reflect axonal integrity, while the remaining eigenvalues are modulated by axonal density, axonal membranes and myelin sheaths, with mean diffusivity (MD) and fractional anisotropy (FA) (the mean and a normalized ratio of eigenvalues, respectively) often used as summary metrics (16-18). These are, however, over-simplistic biological interpretations of the tensor model; as many different and concomitant underlying factors may account for particular metrics derived from the tensor (16,19-21).
The numerous reports of white matter diffusion abnormalities in patients with TLE have been reviewed before (22,23) and it is not necessary to repeat them here, except to provide context for the most commonly found alterations of white matter. The two objectives of this article are (I) to comment on some recent findings that extend our knowledge of white matter abnormalities in TLE; and (II) to collate histological confirmation of white matter abnormalities that could underlie the abnormal water diffusion patterns seen in TLE patients. As we focus on the microscopic features of tissue revealed by dMRI, we will not comment on the use of tractography to infer long-range anatomical connectivity to aid surgical resections or to infer the network properties of the epileptic brain, as those topics have been reviewed recently (24,25).
Diffusion abnormalities of white matter in TLE patients
Abnormalities of the three-dimensional diffusion profile of water molecules have been reported in several white matter structures of TLE patients for more than a decade, since the first demonstration of reduced FA of the external capsule and corpus callosum (26), with a large degree of consistency among publications. Most studies have shown that diffusion abnormalities are not confined to the epileptogenic temporal lobe, with many association, projection and commissural fibers showing alterations. Recently, Otte et al. performed a meta-analysis that included 13 cross-sectional studies and confirmed the existence of temporal and extra-temporal white matter DTI abnormalities (27). The evidence showed that reductions of FA and increases of MD are most prominent in white matter structures closely related to the epileptogenic temporal lobe, such as the uncinate and arcuate fasciculi, cingulum and external capsule, but that contralateral structures as well as the corpus callosum are also affected, albeit to a lesser degree. Further, there seems to be a centrifugal decrease of the degree of abnormalities as tracts extend away from the epileptogenic temporal lobe (27,28). The fornix is the main connection to and from the hippocampus and in some patients it shows volumetric abnormalities (29); notably, the fornix did not prove to be significantly abnormal in this meta-analysis. However, most reports included in the analysis did not use any form of correction for partial volume averaging with the neighboring cerebro-spinal fluid (CSF), an artifact that can on its own mask underlying tissue characteristics and potentially obscure differences between groups (30,31). Indeed, following suppression of the CSF signal, DTI metrics of the fornix in patients with unilateral TLE show clear bilateral abnormalities that do not resolve following seizure freedom and that have been validated by histology of the ipsilateral tract (32-35). Further, the fornix abnormalities seen in adult TLE patients are partially mirrored in an animal model of post-status epilepticus TLE of juvenile rats, who developed transient DTI abnormalities in the corpus callosum, but also chronic reductions of FA in the fornix that corresponded to decreased myelin content (36). A similar study also showed early reductions of FA of the fornix bilaterally after status epilepticus but, notably, these abnormalities were exclusive to the subgroup of rats who ultimately developed spontaneous seizures (37).
Most DTI studies of TLE had, until recently, included patients with TLE regardless of the presence or absence of MTS. However, there is now considerable evidence supporting the idea that DTI abnormalities are more pronounced in patients with radiological evidence of MTS than in patients with non-lesional TLE (nlTLE). Widespread and bilateral reductions of FA do occur in nlTLE patients (38) but, for example, fornix and cingulum DTI abnormalities are much milder in nlTLE patients (34) than in patients with MTS and more evident in the ipsilateral tract (39). Using tract-based spatial statistics (TBSS), Scanlon et al., showed that TLE patients with MTS have more widespread DTI abnormalities than nlTLE patients (40), a finding that was also observed using tractography of several structures, with nlTLE patients showing DTI abnormalities only in the inferior portion of the cingulum and the tapetum (41). Moreover, in patients with MTS, several white matter structures exhibit progressive white matter diffusion abnormalities, suggesting a gradual degenerative process in response to recurrent seizures (42).
Previous studies have pooled TLE patients with left and right hemisphere onset as a homogeneous group, although it has long been recognized that clinical signs and symptoms of TLE differ depending on the hemisphere of onset. While both groups of TLE patients exhibit white matter DTI abnormalities, they are distinguished by degree and spatial pattern. Tractography of eight paired tracts indicated that those most affected are ipsilateral to seizure onset; however, TLE patients with right hemisphere onset showed a more marked tendency to have ipsilateral abnormalities, while patients with left hemisphere onset tend to have bilateral involvement. This information was enough to correctly lateralize 90% of the patients included using a linear discriminant analysis (43). Another study, in contrast, showed that right TLE patients had DTI abnormalities in more tracts than patients with left TLE, with bilateral involvement more prevalent in right TLE (41). A greater involvement of the limbic system, as well as more widespread abnormalities were seen in left TLE patients in a TBSS study (44), but TLE patients with right hemisphere onset had bilateral temporal lobe DTI abnormalities. FA of the tapetum was recently found to correlate with contralateral interictal spikes in TLE only in patients with left hemisphere onset, suggestive of chronic white matter damage by ongoing seizures and distinct neuroplastic mechanisms depending on hemispheric seizure onset (45). Other studies have corroborated the widespread affections and stronger lateralization of DTI abnormalities in left TLE, as compared to right TLE (42,46,47), and an increased inter-tract correlation in left TLE, such that FA reductions in one tract are likely to be accompanied by reduced FA in other tracts (48). The somewhat conflicting results do not allow us to conclude if left or right onset TLE shows greater severity. Moreover, the experimental design needed to answer this question is complex: by discriminating patients according to seizure onset side, patients with or without MTS are often grouped together although, as we reviewed above, the presence of MTS also influences the degree and spatial pattern of abnormalities, and some patients show bilateral electrophysiological evidence of seizure activity. In a voxel-based analysis that included patients with and without MTS who were also grouped by seizure onset side, the most widespread and prominent DTI abnormalities were seen in patients with MTS and left hemispheric onset; no DTI abnormalities were detected in right nlTLE patients (49). As patient groups were not compared directly but only contrasted to healthy controls, it is difficult to ascertain the interaction between MTS and hemispheric seizure onset. Further studies are needed, in which an adequate number of patients are included in each of these four groups, ideally with clear and objective confirmation of both the presence of MTS (e.g., post-surgical histological confirmation or pre-surgical T2 relaxometry), and unequivocal electrophysiological lateralization.
Some studies have directly interrogated the effect of surgical resection on the structure of white matter (Figure 1). In the ipsilateral hemisphere, the fornix and other tracts intimately related to the resected tissue show DTI changes compatible with expected Wallerian degeneration (33,52-55). What is most intriguing, however, is that more superiorly, particularly in the corona radiata, there is an increase of FA (due to increased parallel and reduced radial diffusivities) (50,55). Nearby tracts such as the uncinate fasciculus and external capsule also showed FA increase post-surgically. Similar findings were also reported by Pustina et al. (51), with the post-surgical FA increase in the coronal radiata exceeding the normal values of healthy controls; this, along with analysis of the tensor mode, suggests a reorganization of fibers in that region, with degeneration of a group of fibers in this region of crossing fibers. Moreover, patients with left TLE had a post-surgical normalization of FA in the contralateral superior longitudinal and uncinate fascicles, with the former showing a correlation with postoperative verbal fluency; this phenomenon was not observed in right TLE patients, which points to a certain degree of plasticity of the non-dominant language tracts. While it is certainly possible that some white matter fascicles undergo plastic changes following surgery, the paradoxical improvement of FA values in regions of known fiber crossings highlights the inability of the tensor model to fully capture information in regions of complex architectural configurations, and the importance of careful biological interpretations of non-invasive assessments of white matter through diffusion MRI in general (16,20,56).
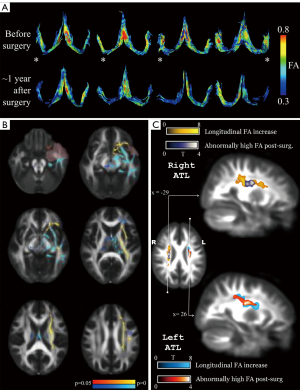
DTI of pediatric TLE
An intriguing question regarding white matter diffusion abnormalities is whether they precede or are a consequence of seizures, and pediatric studies begin to provide information in this regard. Although dMRI and tractography can be reliably performed in pediatric epilepsy (57,58), their use in pediatric TLE has not been as extensive as in adults. The shorter duration of disease in pediatric TLE patients should minimize any potential progressive abnormalities of white matter secondary to seizures, but it must be considered that unlike adult TLE, the etiology of pediatric TLE is often secondary to specific lesions such as focal cortical dysplasia (FCD), tumors or malformations, making a direct comparison to adult TLE less straightforward (59,60). Despite this limitation, TLE patients have shown bilateral temporal lobe white matter diffusion abnormalities characterized by an increase of MD, but no changes of FA (61), as well as bilateral extra-temporal DTI abnormalities (62). In another study, the ipsilateral uncinate, arcuate and inferior longitudinal fascicles, as well as the corticospinal tract, showed bilateral diffusion abnormalities, with correlations of left uncinate and arcuate fascicles with disease duration (63). Diffusion kurtosis imaging (DKI) seems to be more sensitive than DTI in children with TLE, who showed normal tensor-derived parameters, but bilateral diffusion kurtosis abnormalities of the temporal lobe white matter, with greater reductions of radial kurtosis on the ipsilateral hemisphere (64). As in adults (65), other forms of pediatric epilepsy also show diffusion abnormalities of white matter. The cingulum and splenium are abnormal in children of 8-18 years, with several forms of idiopathic epilepsies (66) and reduced FA has been found in right fronto-occipital white matter in children with frontal lobe epilepsy, which was associated with poorer cognitive abilities (67). White matter DTI abnormalities are found in the same lobe as FCD in slightly more than half of pediatric patients (12) and in white matter adjacent to areas of hypometabolism (68). It is very interesting that in some forms of idiopathic epilepsy, white matter diffusion parameters normalize following remission but not in patients with ongoing seizures; while this finding cannot be directly extrapolated to adult TLE patients, who show persistent abnormalities of the fornix after seizure freedom (33), it is possible that some diffusion abnormalities are transient and secondary to epileptic activity.
Whether childhood febrile status epilepticus (FSE) leads to TLE is still unknown and debated. Hippocampal T2 hyperintensities and increased volume are seen soon after the occurrence of febrile status epilepticus in 11.5% of patients, many of whom show hippocampal sclerosis one year later (69). Moreover, children with a history of FSE have a larger prevalence of hippocampal malformations, particularly malrotation (70). In a longitudinal study (71), children who presented FSE had FA reductions throughout the brain one month after the event, but these abnormalities essentially disappeared after one year. The authors of said study suggest that these findings could reflect a temporary pause of white matter development which resumes if the insult is not repeated, or they may indicate an underlying pre-existing structural abnormality that renders these children more prone to FSE. As unprovoked seizures occur only several years after FSE, it is still too early to know how many of those patients will develop TLE.
Neuropsychological implications of white matter abnormalities
Cognitive functions rely on the association of multimodal information, a task that is supported by the long-range connections provided by white matter fascicles. With the robust evidence of widespread white matter microstructural abnormalities in TLE patients, it should not come as a surprise that these patients exhibit cognitive disabilities in several domains (72,73). Memory, both delayed and immediate, correlates with FA of the anterior and medial portions of the temporal lobe, respectively (74), and verbal memory deficits are associated with diffusion abnormalities of the uncinate, arcuate and inferior fronto-occipital fascicles, the inferior portion of the cingulum and the white matter directly underneath the entorhinal cortex (75-77). Patients with left TLE tend to have more pronounced deficits of working memory (78), which could be due to the extensive white matter abnormalities seen in this patient group (42). Additionally, patients with hyperintense temporal lobe white matter and blurring of the gray/white matter interface show more neuropsychological deficiencies than patients without blurring (79). The fronto-parietal network that supports working memory is known to be disrupted in patients with TLE (80), with specific diffusion abnormalities in the interconnecting white matter. Specifically, the diffusion characteristics of the superior longitudinal fasciculus, cingulum and contralateral temporal lobe were associated with working memory performance in patients with left TLE and MTS (81). Although the fornix is intimately associated with the hippocampus, its diffusion parameters do not correlate with memory performance (77,82), yet they do correlate with processing speed, particularly in TLE patients without MTS (82).
There is considerable evidence of language impairments in patients with TLE (83), which is somewhat to be expected considering the alterations of white matter bundles involved in its perception and production (84). The spatial organization of the functional cortical language networks are disrupted in TLE patients, particularly with left hemispheric onset, who show increased right hemisphere structural connections and reduced ipsilateral connectivity (85). These differences in brain connectivity are likely explained by the altered diffusion parameters (namely reduced FA and increased MD) seen in the uncinate, arcuate and inferior fronto-occipital fasicles, as well as the inferior portion of the cingulum (75,77,86). Additionally, hemispheric dominance can sometimes be difficult in TLE patients and may require the use of the invasive Wada test, but this test can be superseded by imaging. Combining dMRI metrics of frontal and temporal white matter bundles and morphologic data, language hemispheric dominance can be lateralized non-invasively (87), and language function can be accurately predicted (88). Finally, the extent of surgical resection of the fiber bundles involved in language predicts postsurgical naming deficits (89).
As dMRI becomes more widely utilized during pre- and postsurgical evaluation of TLE patients, it will provide more information on the association of white matter pathology and cognitive decline associated with or caused by this neurological disorder.
Histological white matter features behind dMRI abnormalities
The interpretation of white matter diffusion abnormalities (seen in epilepsy or other conditions) is generally based on previous studies that related tissue microstructure and ultrastructural characteristics to the spatial profile of water diffusion (13), but often the diffusion abnormalities are over-simplistically related to a “loss of tissue integrity” (56). Few studies have directly correlated dMRI metrics with histological features of human specimens in specific white matter regions within the temporal lobe (35,79). These studies, along with the histopathological features described in brain specimens of TLE patients provide relevant information regarding the mechanisms that cause water diffusion to behave abnormally in several white matter bundles in TLE patients. The most common histological abnormalities within the temporal lobes are described below; notably, each of these features is seen in only 17-36% of surgical specimens, but evidence of at least one abnormality is detected in nearly 63% of all cases (90).
White matter gliosis
Gliosis is observed in most FCD, but it is also a common finding in the white matter of TLE patients (91-93) although it has no apparent relation to the severity of the disorder (94). Astrocytic gliosis, traditionally assessed by the expression of glial fibrillary acidic protein (GFAP), has been found in 18% to 100% of specimens of TLE patients, with severity ranging from mild to marked gliosis (79,94,95) and associated with enlarged glial cell nuclear volume (96,97). Even though gliosis is often seen in TLE specimens, it does not seem to be related to T2 signal changes or diffusion metrics of the temporal lobe white matter (98). Nevertheless it was proposed that the diffuse gliosis found in the temporal lobe white matter of patients with TLE could be an extension of temporal lobe dysplasia in patients with MTS (99), or an adaptive compensatory reaction to frequent seizures (92,94).
Oligodendroglia is prominently increased in the white matter of TLE patients as compared to controls, but it is equally high in TLE patients with or without MTS (100). Oligodendrogliosis in white matter is often found as clusters of cells (90,91,100-103), and they appear to be slightly more common in patients with gray/white matter blurring and related to reduced myelin content (91). Further, oligodendrocytes (OL) can be observed as groups or rows of small round cells along white matter vessels (perivascular clusters) and around a single neuron (perineural OL) (92,100-102,104). Even though the main function of OL in the central nervous system is myelination, their increased numbers in white matter tissue in TLE needs further investigation, as it could be due to a pathophysiological or compensatory mechanism in epileptogenesis, and their increased number may be a sign of an active attempt to compensate for ongoing myelin loss (79,91,105).
Heterotopic neurons in white matter
Normally, a few neurons are interspersed within the white matter fascicles (106,107), but their density is often increased in brain specimens of TLE patients as compared to controls (92,93,108-111) and is independent of white matter gliosis (90,93). These neurons are frequently seen in the yuxtacortical white matter; they blur the border between the gray and white matter in specimens of FCD type IIa, IIb, and IIIa (112), but they do not seem to cause blurring in TLE specimens (79). Similar to white matter gliosis, these ectopic white matter neurons do not seem to be associated with temporal lobe white matter hyperintensities or reduced diffusion anisotropy, likely because their dimensions and numbers are not sufficient to substantially affect the diffusion of water in white matter.
Temporal gray/white matter demarcation (blurring)
Blurring of the border between gray and white matter is a common finding in conventional MRI of patients with TLE, particularly in patients with earlier onset of the disorder (79,110,113), and in patients with FCD type IIa, IIb, and IIIa. As previously stated, heterotopic neurons can be responsible for this feature, although blurring in TLE specimens is also secondary to reduced myelin content and reduced axonal density (79,93,112). Blurring is present in MRI images of 32-65% of TLE patients with MTS (91,113,114), and it is of great lateralizing value, as it tends to be on in the same hemisphere as the sclerotic hippocampus and, in cases of bilateral hippocampal damage, it follows the more severely affected mesial temporal lobe (113).
Myelin and axon abnormalities
Hypomyelination or dysmyelination are presumed to be responsible for temporal lobe white matter hyperintensities and blurring of the temporal grey/white matter border (79,91,113) and are associated with FCD Type II (115). Overall myelin content can be reduced by decreasing its production, increasing its degradation, or reducing the number of myelinated axons (116), with the latter being the most likely in patients with TLE. A study by Garbelli et al. of surgical specimens of TLE patients showed evidence of large patches of reduced myelin content in the white matter underlying the temporal neocortex, as well as prominently reduced axonal density of both myelinated and non-myelinated axons (Figure 2A-J) (79). Reduced axonal density decreases the overall coherence of the white matter and minimizes the barriers that hinder water diffusion, thus allowing a more isotropic diffusion profile, which was also demonstrated in this study by acquiring dMRI of the excised temporal lobes at 7 T. These findings confirm the previously reported positive correlation between cummulative axonal membrane circumference and pre-surgical FA of the fornix of TLE patients with and without MTS (Figure 2K-O) (35). Supporting the finding of axonal loss in TLE, an electron microscopy study of the fornix of cadavers of patients who had TLE showed axonal density to be significantly reduced bilaterally, as compared to non-TLE specimens, with myelinated and non-myelinated axons affected equally (117). An interesting finding in these and other studies (105,111) is the preferential loss of small-diameter axons; the large caliber axons are spared, albeit with abnormal morphology and alterations in their myelin sheaths (Figure 3). In an animal model of TLE, myelinated fibers within the hippocampus showed loss of fibers with diameters of less than one μm, reduced myelin basic protein content, and stratification, collapse and disruption of the myelin sheaths (105). Although these architectural abnormalities of myelin are evident and have been reported repeatedly (Figure 3), it is important to mention that myelin layers are particularly sensitive to differences in tissue processing methods (118) and therefore abnormalities can only be assessed with judiciously selected control tissue that has undergone equal procedures, and caution must be exercised when attempting to compare the characteristics of myelin across studies.
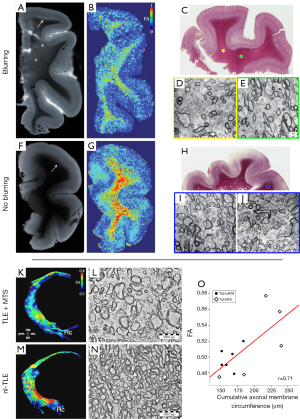
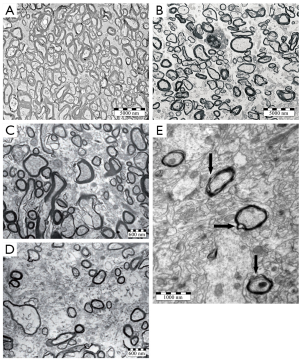
Despite differences in methodological procedures, reduced axonal density is a robust finding in the temporal lobe white matter of TLE patients, and the subsequent increase of extra-cellular space is sufficient to reduce diffusion anisotropy and increase MD at the expense of increased diffusivity perpendicular to the axons, which are precisely the characteristic findings of DTI studies in TLE patients. The nature, origin and function of the affected axons are unknown, as is the mechanism that underlies progressive structural damage in TLE, which could include excitotoxic effects from seizure activity (63,119,120), or deafferentation resulting from mesial temporal cell loss (121,122).
Conclusions
While the impact of dMRI on clinical decisions regarding patients with TLE has not been as dramatic as with stroke patients, it has certainly proved to be an invaluable research tool in the study of white matter in TLE. It has enabled us to shift our perception of this particular form of epilepsy from a focal to a brain network disorder, which in turn allows us to better understand its associated cognitive, psychological and psychiatric repercussions. There is considerable evidence of extensive white matter diffusion abnormalities in many brain regions which, backed by direct correlations with histology and a wealth of information derived from histopathological examinations of surgical and post-mortem specimens, very likely reflect reduced axonal density and disruption of myelin sheaths.
Given the low data requirements of the tensor model, DTI has played a prominent role in the field. However, its theoretical assumptions limit its use and interpretability in regions of white matter crossings, which are in reality the majority of white matter (123). The problems of DTI can be overcome by new analytical methods that allow sufficient data to be acquired in time intervals suitable for the clinical setting (124). Moreover, many of these methods provide more insight into tissue microstructure than the tensor model, and they are increasingly being refined and extended.
As more information regarding the microstructure of white matter in TLE patients is gathered through dMRI and extended by tractography and network-based analyses, we will be able to form a picture of their nature, temporal evolution, and whether they represent secondary damage due to ongoing seizures or a predisposing factor for their presentation. Further studies may subdivide and correlate the spectrum of TLE according to specific white matter abnormalities and the underlying epileptogenic process, be it acquired, congenital or developmental.
Acknowledgements
The authors thank Dr. Jorge A. Larriva-Sahd for useful discussions about electron microscopy, and Dorothy Pless for proofreading and editing. This work was partially funded by grants UNAM-DGAPA IB201712 and Conacyt 181508. We also thank the Posgrado en Ciencias Biomédicas of the Universidad Nacional Autónoma de México (UNAM) and the Conacyt for Graduate Fellowship 329866 to R.R.C.
Disclosure: The authors declare no conflict of interest.
References
- Engel J. Report of the ILAE classification core group. Epilepsia 2006;47:1558-68. [PubMed]
- Blümcke I, Thom M, Aronica E, Armstrong DD, Bartolomei F, Bernasconi A, Bernasconi N, Bien CG, Cendes F, Coras R, Cross JH, Jacques TS, Kahane P, Mathern GW, Miyata H, Moshé SL, Oz B, Özkara Ç, Perucca E, Sisodiya S, Wiebe S, Spreafico R. International consensus classification of hippocampal sclerosis in temporal lobe epilepsy: a Task Force report from the ILAE Commission on Diagnostic Methods. Epilepsia 2013;54:1315-29. [PubMed]
- Engel J Jr, Wiebe S. Who is a surgical candidate? Handb Clin Neurol 2012;108:821-8. [PubMed]
- Semah F, Picot MC, Adam C, Broglin D, Arzimanoglou A, Bazin B, Cavalcanti D, Baulac M. Is the underlying cause of epilepsy a major prognostic factor for recurrence? Neurology 1998;51:1256-62. [PubMed]
- Bernasconi A. Magnetic resonance imaging in intractable epilepsy: focus on structural image analysis. Adv Neurol 2006;97:273-8. [PubMed]
- Bernasconi N, Bernasconi A, Caramanos Z, Antel SB, Andermann F, Arnold DL. Mesial temporal damage in temporal lobe epilepsy: a volumetric MRI study of the hippocampus, amygdala and parahippocampal region. Brain 2003;126:462-9. [PubMed]
- Cendes F, Caramanos Z, Andermann F, Dubeau F, Arnold DL. Proton magnetic resonance spectroscopic imaging and magnetic resonance imaging volumetry in the lateralization of temporal lobe epilepsy: a series of 100 patients. Ann Neurol 1997;42:737-46. [PubMed]
- Jackson GD, Connelly A, Duncan JS, Grunewald RA, Gadian DG. Detection of hippocampal pathology in intractable partial epilepsy: increased sensitivity with quantitative magnetic resonance T2 relaxometry. Neurology 1993;43:1793-9. [PubMed]
- Diehl B, Symms MR, Boulby PA, Salmenpera T, Wheeler-Kingshott CA, Barker GJ, Duncan JS. Postictal diffusion tensor imaging. Epilepsy Res 2005;65:137-46. [PubMed]
- Gong G, Shi F, Concha L, Beaulieu C, Gross DW. Insights into the Sequence of Structural Consequences of Convulsive Status Epilepticus: a Longitudinal Mri Study. Epilepsia 2008;49:1941-5. [PubMed]
- Salmenpera TM, Symms MR, Boulby PA, Barker GJ, Duncan JS. Postictal diffusion weighted imaging. Epilepsy Res 2006;70:133-43. [PubMed]
- Widjaja E, Geibprasert S, Otsubo H, Snead OC, Mahmoodabadi SZ. Diffusion Tensor Imaging Assessment of the Epileptogenic Zone in Children with Localization-Related Epilepsy. Am J Neuroradiol 2011;32:1789-94. [PubMed]
- Beaulieu C. The basis of anisotropic water diffusion in the nervous system - a technical review. NMR Biomed 2002;15:435-55. [PubMed]
- Mori S, van Zijl PC. Fiber tracking: principles and strategies - a technical review. NMR Biomed 2002;15:468-80. [PubMed]
- Basser PJ, Mattiello J, LeBihan D. MR diffusion tensor spectroscopy and imaging. Biophys J 1994;66:259-67. [PubMed]
- Concha L. A macroscopic view of microstructure: Using diffusion-weighted images to infer damage, repair, and plasticity of white matter. Neuroscience 2014;276:14-28. [PubMed]
- Hagmann P, Jonasson L, Maeder P, Thiran J-P, Wedeen VJ, Meuli R. Understanding Diffusion MR Imaging Techniques: From Scalar Diffusion-weighted Imaging to Diffusion Tensor Imaging and Beyond1. Radiographics 2006;26:S205-23. [PubMed]
- Winston GP. The physical and biological basis of quantitative parameters derived from diffusion MRI. Quant Imaging Med Surg 2012;2:254-65. [PubMed]
- Jones DK, Cercignani M. Twenty-five pitfalls in the analysis of diffusion MRI data. NMR Biomed 2010;23:803-20. [PubMed]
- Walhovd KB, Johansen-Berg H, Káradóttir RT. Unraveling the secrets of white matter - Bridging the gap between cellular, animal and human imaging studies. Neuroscience 2014;276:2-13. [PubMed]
- O’Donnell LJ, Pasternak O. Does diffusion MRI tell us anything about the white matter? An overview of methods and pitfalls. Schizophr Res 2015;161:133-41. [PubMed]
- Yogarajah M, Duncan JS. Diffusion-based magnetic resonance imaging and tractography in epilepsy. Epilepsia 2008;49:189-200. [PubMed]
- Gross DW. Diffusion tensor imaging in temporal lobe epilepsy. Epilepsia 2011;52 Suppl 4:32-4. [PubMed]
- Bernhardt BC, Hong S, Bernasconi A, Bernasconi N. Imaging structural and functional brain networks in temporal lobe epilepsy. Front Hum Neurosci 2013;7:624. [PubMed]
- Winston GP. Epilepsy surgery, vision, and driving: What has surgery taught us and could modern imaging reduce the risk of visual deficits? Epilepsia 2013;54:1877-88. [PubMed]
- Arfanakis K, Hermann BP, Rogers BP, Carew JD, Seidenberg M, Meyerand ME. Diffusion tensor MRI in temporal lobe epilepsy. Magn Reson Imaging 2002;20:511-9. [PubMed]
- Otte WM, van Eijsden P, Sander JW, Duncan JS, Dijkhuizen RM, Braun KP. A meta-analysis of white matter changes in temporal lobe epilepsy as studied with diffusion tensor imaging. Epilepsia 2012;53:659-67. [PubMed]
- Concha L, Kim H, Bernasconi A, Bernhardt BC, Bernasconi N. Spatial patterns of water diffusion along white matter tracts in temporal lobe epilepsy. Neurology 2012;79:455-62. [PubMed]
- Kuzniecky R, Bilir E, Gilliam F, Faught E, Martin R, Hugg J. Quantitative MRI in temporal lobe epilepsy: Evidence for fornix atrophy. Neurology 1999;53:496. [PubMed]
- Concha L, Gross DW, Beaulieu C. Diffusion Tensor Tractography of the Limbic System. AJNR Am J Neuroradiol 2005;26:2267-74. [PubMed]
- Vos SB, Jones DK, Viergever MA, Leemans A. Partial volume effect as a hidden covariate in DTI analyses. NeuroImage 2011;55:1566-76. [PubMed]
- Concha L, Beaulieu C, Gross DW. Bilateral limbic diffusion abnormalities in unilateral temporal lobe epilepsy. Ann Neurol 2005;57:188-96. [PubMed]
- Concha L, Beaulieu C, Wheatley BM, Gross DW. Bilateral White Matter Diffusion Changes Persist after Epilepsy Surgery. Epilepsia 2007;48:931-40. [PubMed]
- Concha L, Beaulieu C, Collins DL, Gross DW. White matter diffusion abnormalities in temporal lobe epilepsy with and without mesial temporal sclerosis. J Neurol Neurosurg Psychiatry 2009;80:312-9. [PubMed]
- Concha L, Livy DJ, Beaulieu C, Wheatley BM, Gross DW. In Vivo Diffusion Tensor Imaging and Histopathology of the Fimbria-Fornix in Temporal Lobe Epilepsy. J Neurosci 2010;30:996-1002. [PubMed]
- van Eijsden P, Otte WM, van der Hel WS, van Nieuwenhuizen O, Dijkhuizen RM, de Graaf RA, Braun KP. In vivo diffusion tensor imaging and ex vivo histologic characterization of white matter pathology in a post-status epilepticus model of temporal lobe epilepsy. Epilepsia 2011;52:841-5. [PubMed]
- Parekh MB, Carney PR, Sepulveda H, Norman W, King M, Mareci TH. Early MR diffusion and relaxation changes in the parahippocampal gyrus precede the onset of spontaneous seizures in an animal model of chronic limbic epilepsy. Exp Neurol 2010;224:258-70. [PubMed]
- Keller SS, Ahrens T, Mohammadi S, Gerdes JS, Möddel G, Kellinghaus C, Kugel H, Weber B, Ringelstein EB, Deppe M. Voxel-Based Statistical Analysis of Fractional Anisotropy and Mean Diffusivity in Patients with Unilateral Temporal Lobe Epilepsy of Unknown Cause. J Neuroimaging 2013;23:352-9. [PubMed]
- Liacu D, Idy-Peretti I, Ducreux D, Bouilleret V, de Marco G. Diffusion tensor imaging tractography parameters of limbic system bundles in temporal lobe epilepsy patients. J Magn Reson Imaging 2012;36:561-8. [PubMed]
- Scanlon C, Mueller SG, Cheong I, Hartig M, Weiner MW, Laxer KD. Grey and white matter abnormalities in temporal lobe epilepsy with and without mesial temporal sclerosis. J Neurol 2013;260:2320-9. [PubMed]
- Liu M, Concha L, Lebel C, Beaulieu C, Gross DW. Mesial temporal sclerosis is linked with more widespread white matter changes in temporal lobe epilepsy. NeuroImage Clin 2012;1:99-105. [PubMed]
- Keller SS, Schoene-Bake JC, Gerdes JS, Weber B, Deppe M. Concomitant Fractional Anisotropy and Volumetric Abnormalities in Temporal Lobe Epilepsy: Cross-Sectional Evidence for Progressive Neurologic Injury. PLoS ONE 2012;7:e46791. [PubMed]
- Ahmadi ME, Hagler DJ Jr, McDonald CR, Tecoma ES, Iragui VJ, Dale AM, Halgren E. Side matters: diffusion tensor imaging tractography in left and right temporal lobe epilepsy. AJNR Am J Neuroradiol 2009;30:1740-7. [PubMed]
- Focke NK, Yogarajah M, Bonelli SB, Bartlett PA, Symms MR, Duncan JS. Voxel-based diffusion tensor imaging in patients with mesial temporal lobe epilepsy and hippocampal sclerosis. NeuroImage 2008;40:728-37. [PubMed]
- Pustina D, Doucet G, Skidmore C, Sperling M, Tracy J. Contralateral interictal spikes are related to tapetum damage in left temporal lobe epilepsy. Epilepsia 2014;55:1406-14. [PubMed]
- Besson P, Dinkelacker V, Valabregue R, Thivard L, Leclerc X, Baulac M, Sammler D, Colliot O, Lehéricy S, Samson S, Dupont S. Structural connectivity differences in left and right temporal lobe epilepsy. NeuroImage 2014;100:135-44. [PubMed]
- Kemmotsu N, Girard HM, Bernhardt BC, Bonilha L, Lin JJ, Tecoma ES, Iragui VJ, Hagler DJ Jr, Halgren E, McDonald CR. MRI analysis in temporal lobe epilepsy: Cortical thinning and white matter disruptions are related to side of seizure onset. Epilepsia 2011;52:2257-66. [PubMed]
- Pustina D, Doucet G, Sperling M, Sharan A, Tracy J. Increased microstructural white matter correlations in left, but not right, temporal lobe epilepsy. Hum Brain Mapp 2015;36:85-98. [PubMed]
- Shon YM, Kim YI, Koo BB, Lee JM, Kim HJ, Kim WJ, Ahn KJ, Yang DW. Group-specific regional white matter abnormality revealed in diffusion tensor imaging of medial temporal lobe epilepsy without hippocampal sclerosis. Epilepsia 2010;51:529-35. [PubMed]
- Winston GP, Stretton J, Sidhu MK, Symms MR, Duncan JS. Progressive white matter changes following anterior temporal lobe resection for epilepsy. NeuroImage Clin 2014;4:190-200. [PubMed]
- Pustina D, Doucet G, Evans J, Sharan A, Sperling M, Skidmore C, Tracy J. Distinct Types of White Matter Changes Are Observed after Anterior Temporal Lobectomy in Epilepsy. PLoS ONE 2014;9:e104211. [PubMed]
- Faber J, Schoene-Bake JC, Trautner P, von Lehe M, Elger CE, Weber B. Progressive fiber tract affections after temporal lobe surgery. Epilepsia 2013;54:e53-7. [PubMed]
- Liu M, Gross DW, Wheatley BM, Concha L, Beaulieu C. The acute phase of Wallerian degeneration: Longitudinal diffusion tensor imaging of the fornix following temporal lobe surgery. NeuroImage 2013;74:128-39. [PubMed]
- Schoene-Bake JC, Faber J, Trautner P, Kaaden S, Tittgemeyer M, Elger CE, Weber B. Widespread affections of large fiber tracts in postoperative temporal lobe epilepsy. NeuroImage 2009;46:569-76. [PubMed]
- Yogarajah M, Focke NK, Bonelli SB, Thompson P, Vollmar C, McEvoy AW, Alexander DC, Symms MR, Koepp MJ, Duncan JS. The structural plasticity of white matter networks following anterior temporal lobe resection. Brain 2010;133:2348-64. [PubMed]
- Jones DK, Knösche TR, Turner R. White matter integrity, fiber count, and other fallacies: The do’s and don’ts of diffusion MRI. NeuroImage 2013;73:239-54. [PubMed]
- Carlson HL, Laliberté C, Brooks BL, Hodge J, Kirton A, Bello-Espinosa L, Hader W, Sherman EM. Reliability and variability of diffusion tensor imaging (DTI) tractography in pediatric epilepsy. Epilepsy Behav 2014;37:116-22. [PubMed]
- Malfait D, Tucholka A, Lippé S. Diffusion-weighted magnetic resonance imaging and pediatric epilepsy. J Pediatr Epilepsy 2013;2:49-61.
- Mohamed A, Wyllie E, Ruggieri P, Kotagal P, Babb T, Hilbig A, Wylie C, Ying Z, Staugaitis S, Najm I, Bulacio J, Foldvary N, Lüders H, Bingaman W. Temporal lobe epilepsy due to hippocampal sclerosis in pediatric candidates for epilepsy surgery. Neurology 2001;56:1643-9. [PubMed]
- Nickels KC, Wong-Kisiel LC, Moseley BD, Wirrell EC. Temporal Lobe Epilepsy in Children. Epilepsy Res Treat 2012;2012:849540.
- Nilsson D, Go C, Rutka JT, Rydenhag B, Mabbott DJ, Snead OC 3rd, Raybaud CR, Widjaja E. Bilateral diffusion tensor abnormalities of temporal lobe and cingulate gyrus white matter in children with temporal lobe epilepsy. Epilepsy Res 2008;81:128-35. [PubMed]
- Meng L, Xiang J, Rose D, Kotecha R, Vannest J, Byars A, Degrauw T. White Matter Abnormalities in Children with Temporal Lobe Epilepsy: A DTI and MEG Study. In: Supek S, Sušac A. eds. 17th International Conference on Biomagnetism Advances in Biomagnetism - Biomag2010. Springer Berlin Heidelberg, 2010;28:397-400.
- Govindan RM, Makki MI, Sundaram SK, Juhász C, Chugani HT. Diffusion tensor analysis of temporal and extra-temporal lobe tracts in temporal lobe epilepsy. Epilepsy Res 2008;80:30-41. [PubMed]
- Gao Y, Zhang Y, Wong CS, Wu PM, Zhang Z, Gao J, Qiu D, Huang B. Diffusion abnormalities in temporal lobes of children with temporal lobe epilepsy: a preliminary diffusional kurtosis imaging study and comparison with diffusion tensor imaging. NMR Biomed 2012;25:1369-77. [PubMed]
- Liu M, Concha L, Beaulieu C, Gross DW. Distinct white matter abnormalities in different idiopathic generalized epilepsy syndromes. Epilepsia 2011;52:2267-75. [PubMed]
- Hutchinson E, Pulsipher D, Dabbs K, Myers y Gutierrez A, Sheth R, Jones J, Seidenberg M, Meyerand E, Hermann B. Children with new-onset epilepsy exhibit diffusion abnormalities in cerebral white matter in the absence of volumetric differences. Epilepsy Res 2010;88:208-14. [PubMed]
- Braakman HM, Vaessen MJ, Jansen JF, Debeij-van Hall MH, de Louw A, Hofman PA, Vles JS, Aldenkamp AP, Backes WH. Pediatric frontal lobe epilepsy: white matter abnormalities and cognitive impairment. Acta Neurol Scand 2014;129:252-62. [PubMed]
- Lippé S, Poupon C, Cachia A, Archambaud F, Rodrigo S, Dorfmuller G, Chiron C, Hertz-Pannier L. White matter abnormalities revealed by DTI correlate with interictal grey matter FDG-PET metabolism in focal childhood epilepsies. Epileptic Disord 2012;14:404-13. [PubMed]
- Lewis DV, Shinnar S, Hesdorffer DC, Bagiella E, Bello JA, Chan S, Xu Y, MacFall J, Gomes WA, Moshé SL, Mathern GW, Pellock JM, Nordli DR Jr, Frank LM, Provenzale J, Shinnar RC, Epstein LG, Masur D, Litherland C, Sun S; FEBSTAT Study Team. Hippocampal sclerosis after febrile status epilepticus: The FEBSTAT study. Ann Neurol 2014;75:178-85. [PubMed]
- Shinnar S, Bello JA, Chan S, Hesdorffer DC, Lewis DV, Macfall J, Pellock JM, Nordli DR, Frank LM, Moshe SL, Gomes W, Shinnar RC, Sun S; FEBSTAT Study Team. MRI abnormalities following febrile status epilepticus in children The FEBSTAT study. Neurology 2012;79:871-7. [PubMed]
- Yoong M, Seunarine K, Martinos M, Chin RF, Clark CA, Scott RC. Prolonged febrile seizures cause reversible reductions in white matter integrity. NeuroImage Clin 2013;3:515-21. [PubMed]
- Bell B, Lin JJ, Seidenberg M, Hermann B. The neurobiology of cognitive disorders in temporal lobe epilepsy. Nat Rev Neurol 2011;7:154-64. [PubMed]
- Hermann B, Seidenberg M, Lee EJ, Chan F, Rutecki P. Cognitive phenotypes in temporal lobe epilepsy. J Int Neuropsychol Soc 2007;13:12-20. [PubMed]
- Riley JD, Franklin DL, Choi V, Kim RC, Binder DK, Cramer SC, Lin JJ. Altered white matter integrity in temporal lobe epilepsy: Association with cognitive and clinical profiles. Epilepsia 2010;51:536-45. [PubMed]
- Diehl B, Busch RM, Duncan JS, Piao Z, Tkach J, Luders HO. Abnormalities in Diffusion Tensor Imaging of the Uncinate Fasciculus Relate to Reduced Memory in Temporal Lobe Epilepsy. Epilepsia 2008;49:1409-18. [PubMed]
- McDonald CR, Leyden KM, Hagler DJ, Kucukboyaci NE, Kemmotsu N, Tecoma ES, Iragui VJ. White matter microstructure complements morphometry for predicting verbal memory in epilepsy. Cortex 2014;58:139-50. [PubMed]
- McDonald CR, Ahmadi ME, Hagler DJ, Tecoma ES, Iragui VJ, Gharapetian L, Dale AM, Halgren E. Diffusion tensor imaging correlates of memory and language impairments in temporal lobe epilepsy. Neurology 2008;71:1869-76. [PubMed]
- Tudesco Ide S, Vaz LJ, Mantoan MA, Belzunces E, Noffs MH, Caboclo LO, Yacubian EM, Sakamoto AC, Bueno OF. Assessment of working memory in patients with mesial temporal lobe epilepsy associated with unilateral hippocampal sclerosis. Epilepsy Behav 2010;18:223-8. [PubMed]
- Garbelli R, Milesi G, Medici V, Villani F, Didato G, Deleo F, D’Incerti L, Morbin M, Mazzoleni G, Giovagnoli AR, Parente A, Zucca I, Mastropietro A, Spreafico R. Blurring in patients with temporal lobe epilepsy: clinical, high-field imaging and ultrastructural study. Brain 2012;135:2337-49. [PubMed]
- Stretton J, Winston G, Sidhu M, Centeno M, Vollmar C, Bonelli S, Symms M, Koepp M, Duncan JS, Thompson PJ. Neural correlates of working memory in Temporal Lobe Epilepsy — An fMRI study. NeuroImage 2012;60:1696-703. [PubMed]
- Winston GP, Stretton J, Sidhu MK, Symms MR, Thompson PJ, Duncan JS. Structural correlates of impaired working memory in hippocampal sclerosis. Epilepsia 2013;54:1143-53. [PubMed]
- Alexander RP, Concha L, Snyder TJ, Beaulieu C, Gross DW. Correlations between Limbic White Matter and Cognitive Function in Temporal-Lobe Epilepsy, Preliminary Findings. Front Aging Neurosci 2014;6:142. [PubMed]
- McAndrews MP, Cohn M. Neuropsychology in temporal lobe epilepsy: influences from cognitive neuroscience and functional neuroimaging. Epilepsy Res Treat 2012;2012:925238.
- Duffau H. The anatomo-functional connectivity of language revisited: New insights provided by electrostimulation and tractography. Neuropsychologia 2008;46:927-34. [PubMed]
- Powell HW, Parker GJ, Alexander DC, Symms MR, Boulby PA, Wheeler-Kingshott CA, Barker GJ, Koepp MJ, Duncan JS. Abnormalities of language networks in temporal lobe epilepsy. Neuroimage 2007;36:209-21. [PubMed]
- Kim CH, Chung CK, Koo BB, Lee JM, Kim JS, Lee SK. Changes in Language Pathways in Patients with Temporal Lobe Epilepsy: Diffusion Tensor Imaging Analysis of the Uncinate and Arcuate Fasciculi. World Neurosurg 2011;75:509-16. [PubMed]
- Ellmore TM, Beauchamp MS, Breier JI, Slater JD, Kalamangalam GP, O’Neill TJ, Disano MA, Tandon N. Temporal lobe white matter asymmetry and language laterality in epilepsy patients. NeuroImage 2010;49:2033-44. [PubMed]
- Kucukboyaci NE, Kemmotsu N, Leyden KM, Girard HM, Tecoma ES, Iragui VJ, McDonald CR. Integration of multimodal MRI data via PCA to explain language performance. NeuroImage Clin 2014;5:197-207. [PubMed]
- Powell HW, Parker GJ, Alexander DC, Symms MR, Boulby PA, Barker GJ, Thompson PJ, Koepp MJ, Duncan JS. Imaging language pathways predicts postoperative naming deficits. J Neurol Neurosurg Psychiatry 2008;79:327-30. [PubMed]
- Kasper BS, Stefan H, Buchfelder M, Paulus W. Temporal lobe microdysgenesis in epilepsy versus control brains. J Neuropathol Exp Neurol 1999;58:22-8. [PubMed]
- Meiners LC, Witkamp TD, de Kort GA, van Huffelen AC, van der Graaf Y, Jansen GH, van der Grond J, van Veelen CW. Relevance of temporal lobe white matter changes in hippocampal sclerosis. Magnetic resonance imaging and histology. Invest Radiol 1999;34:38-45. [PubMed]
- Nishio S, Morioka T, Hisada K, Fukui M. Temporal lobe epilepsy: a clinicopathological study with special reference to temporal neocortical changes. Neurosurg Rev 2000;23:84-9. [PubMed]
- Thom M, Sisodiya S, Harkness W, Scaravilli F. Microdysgenesis in temporal lobe epilepsy. A quantitative and immunohistochemical study of white matter neurones. Brain 2001;124:2299-309. [PubMed]
- Novozhilova AP, Gaikova ON. Cellular Gliosis of the White Matter of the Human Brain and Its Importance in the Pathogenesis of Focal Epilepsy. Neurosci Behav Physiol 2002;32:135-9. [PubMed]
- Salanova V, Markand O, Worth R. Temporal lobe epilepsy: analysis of patients with dual pathology. Acta Neurol Scand 2004;109:126-31. [PubMed]
- Bothwell S, Meredith GE, Phillips J, Staunton H, Doherty C, Grigorenko E, Glazier S, Deadwyler SA, O’Donovan CA, Farrell M. Neuronal Hypertrophy in the Neocortex of Patients with Temporal Lobe Epilepsy. J Neurosci 2001;21:4789-800. [PubMed]
- Kendal C, Everall I, Polkey C, Al-Sarraj S. Glial cell changes in the white matter in temporal lobe epilepsy. Epilepsy Res 1999;36:43-51. [PubMed]
- Lockwood-Estrin G, Thom M, Focke NK, Symms MR, Martinian L, Sisodiya SM, Duncan JS, Eriksson SH. Correlating 3T MRI and histopathology in patients undergoing epilepsy surgery. J Neurosci Methods 2012;205:182-9. [PubMed]
- Thom M, Eriksson S, Martinian L, Caboclo LO, McEvoy AW, Duncan JS, Sisodiya SM. Temporal lobe sclerosis associated with hippocampal sclerosis in temporal lobe epilepsy: neuropathological features. J Neuropathol Exp Neurol 2009;68:928-38. [PubMed]
- Stefanits H, Czech T, Pataraia E, Baumgartner C, Derhaschnig N, Slana A, Kovacs GG. Prominent oligodendroglial response in surgical specimens of patients with temporal lobe epilepsy. Clin Neuropathol 2012;31:409-17. [PubMed]
- Kasper BS, Paulus W. Perivascular clustering in temporal lobe epilepsy: oligodendroglial cells of unknown function. Acta Neuropathol 2004;108:471-5. [PubMed]
- Komori T, Arai N, Shimizu H, Yagishita A, Mizutani T, Oda M. Cortical perivascular satellitosis in intractable epilepsy; a form of cortical dysplasia? Acta Neuropathol (Berl) 2002;104:149-54. [PubMed]
- Sakuma S, Halliday WC, Nomura R, Ochi A, Otsubo H. Increased population of oligodendroglia-like cells in pediatric intractable epilepsy. Neurosci Lett 2014;566:188-93. [PubMed]
- Arai N, Umitsu R, Komori T, Hayashi M, Kurata K, Nagata J, Tamagawa K, Mizutani T, Oda M, Morimatsu Y. Peculiar form of cerebral microdysgenesis characterized by white matter neurons with perineuronal and perivascular glial satellitosis: A study using a variety of human autopsied brains. Pathol Int 2003;53:345-52. [PubMed]
- Ye Y, Xiong J, Hu J, Kong M, Cheng L, Chen H, Li T, Jiang L. Altered hippocampal myelinated fiber integrity in a lithium-pilocarpine model of temporal lobe epilepsy: A histopathological and stereological investigation. Brain Res 2013;1522:76-87. [PubMed]
- Defelipe J, Fields RD, Hof PR, Höistad M, Kostovic I, Meyer G, Rockland KS. Cortical white matter: beyond the pale remarks, main conclusions and discussion. Front Neuroanat 2010;4:4. [PubMed]
- Larriva-Sahd J, Condés Lara M, Martínez-Cabrera G, Varela-Echavarria A. Histological and ultrastructural characterization of interfascicular neurons in the rat anterior commissure. Brain Res 2002;931:81-91. [PubMed]
- Emery JA, Roper SN, Rojiani AM. White matter neuronal heterotopia in temporal lobe epilepsy: a morphometric and immunohistochemical study. J Neuropathol Exp Neurol 1997;56:1276-82. [PubMed]
- Eriksson SH, Nordborg C, Rydenhag B, Malmgren K. Parenchymal lesions in pharmacoresistant temporal lobe epilepsy: dual and multiple pathology. Acta Neurol Scand 2005;112:151-6. [PubMed]
- Schijns OE, Bien CG, Majores M, von Lehe M, Urbach H, Becker A, Schramm J, Elger CE, Clusmann H. Presence of temporal gray-white matter abnormalities does not influence epilepsy surgery outcome in temporal lobe epilepsy with hippocampal sclerosis. Neurosurgery 2011;68:98-106; discussion 107. [PubMed]
- Tassi L, Meroni A, Deleo F, Villani F, Mai R, Russo GL, Colombo N, Avanzini G, Falcone C, Bramerio M, Citterio A, Garbelli R, Spreafico R. Temporal lobe epilepsy: neuropathological and clinical correlations in 243 surgically treated patients. Epileptic Disord 2009;11:281-92. [PubMed]
- Blümcke I, Thom M, Aronica E, Armstrong DD, Vinters HV, Palmini A, Jacques TS, Avanzini G, Barkovich AJ, Battaglia G, Becker A, Cepeda C, Cendes F, Colombo N, Crino P, Cross JH, Delalande O, Dubeau F, Duncan J, Guerrini R, Kahane P, Mathern G, Najm I, Ozkara C, Raybaud C, Represa A, Roper SN, Salamon N, Schulze-Bonhage A, Tassi L, Vezzani A, Spreafico R. The clinicopathologic spectrum of focal cortical dysplasias: A consensus classification proposed by an ad hoc Task Force of the ILAE Diagnostic Methods Commission1. Epilepsia 2011;52:158-74. [PubMed]
- Mitchell LA, Harvey AS, Coleman LT, Mandelstam SA, Jackson GD. Anterior Temporal Changes on MR Images of Children with Hippocampal Sclerosis: An Effect of Seizures on the Immature Brain? Am J Neuroradiol 2003;24:1670-7. [PubMed]
- Choi D, Na DG, Byun HS, Suh YL, Kim SE, Ro DW, Chung IG, Hong SC, Hong SB. White-matter change in mesial temporal sclerosis: correlation of MRI with PET, pathology, and clinical features. Epilepsia 1999;40:1634-41. [PubMed]
- Shepherd C, Liu J, Goc J, Martinian L, Jacques TS, Sisodiya SM, Thom M. A quantitative study of white matter hypomyelination and oligodendroglial maturation in focal cortical dysplasia type II. Epilepsia 2013;54:898-908. [PubMed]
- Bradl M, Lassmann H. Oligodendrocytes: biology and pathology. Acta Neuropathol (Berl) 2010;119:37-53. [PubMed]
- Ozdogmus O, Cavdar S, Ersoy Y, Ercan F, Uzun I. A preliminary study, using electron and light-microscopic methods, of axon numbers in the fornix in autopsies of patients with temporal lobe epilepsy. Anat Sci Int 2009;84:2-6. [PubMed]
- Peters A. The Fixation of Central Nervous Tissue and the Analysis of Electron Micrographs of the Neuropil, with Special Reference to the Cerebral Cortex. In: Nauta WJH, Ebbesson SOE. eds. Contemporary Research Methods in Neuroanatomy. Springer-Verlag, New York, 1970:56-76.
- Bonilha L, Rorden C, Appenzeller S, Coan AC, Cendes F, Li LM. Gray matter atrophy associated with duration of temporal lobe epilepsy. Neuroimage 2006;32:1070-9. [PubMed]
- Lin JJ, Riley JD, Juranek J, Cramer SC. Vulnerability of the frontal-temporal connections in temporal lobe epilepsy. Epilepsy Res 2008;82:162-70. [PubMed]
- Gross DW, Concha L, Beaulieu C. Extratemporal White Matter Abnormalities in Mesial Temporal Lobe Epilepsy Demonstrated with Diffusion Tensor Imaging. Epilepsia 2006;47:1360-3. [PubMed]
- Thivard L, Lehéricy S, Krainik A, Adam C, Dormont D, Chiras J, Baulac M, Dupont S. Diffusion tensor imaging in medial temporal lobe epilepsy with hippocampal sclerosis. Neuroimage 2005;28:682-90. [PubMed]
- Jeurissen B, Leemans A, Tournier JD, Jones DK, Sijbers J. Investigating the prevalence of complex fiber configurations in white matter tissue with diffusion magnetic resonance imaging. Hum Brain Mapp 2013;34:2747-66. [PubMed]
- Tournier JD, Mori S, Leemans A. Diffusion tensor imaging and beyond. Magn Reson Med 2011;65:1532-56. [PubMed]