Prediction of suspicious ankle instability using the calcaneofibular ligament cross-sectional area
Introduction
Ankle sprains are one of the most common disorders among the general population, with long-term sequelae extending beyond the initial injury, including repeated injury and perceived instability with recurrent sensations of rolling of the ankle, which contribute to chronic ankle instability (AI) (1). For athletes, AI is one of the most prevalent debilitating diseases (2). A high percentage (20–60%) of athletes are identified as having AI originating from team sports, such as soccer and basketball. Characterization of AI includes sensations of ankle pain, frequent episodes of ankle unsteadiness with prolonged symptoms, and recurrent ankle injuries (3-8). Previous studies have highlighted that AI is a public health-care cost affecting not only athletic populations but also the general population (7). Thus, early diagnosis and management are important in the prevention of AI. During a lateral ankle sprain, the major ligaments in the ankle’s lateral ligament complex are the calcaneofibular ligament (CFL) and anterior talofibular ligament (ATFL) (9). In the neutral state, the CFL’s responsibility is resistance to inversion during dorsiflexion. The CFL stabilizes the subtalar joint and resists inversion alongside the ATFL during plantarflexion (10,11). Ankle magnetic resonance imaging (A-MRI) is beneficial for analyzing the pathologic abnormalities of the CFL and other related pathologic conditions in the ankle anatomy (12). Orthopedic surgeons consider the A-MRI findings when investigating morphologic changes in the CFL to assist with treatment choice decisions (1). Previous research has assessed the CFL using a simple measurement at the “halfway” point of the CFL (13); however, asymmetrical and partial inflammatory hypertrophies of the CFL can occur at any point.
Consequently, a measurement error bias is possible. In contrast to the CFLT, the cross-sectional area of the CFL will not suffer from measurement error bias because it measures a much broader area of the CFL. Therefore, to analyze the hypertrophy of the CFL, we created the CFL cross-sectional area (CFLCSA) as a new image analysis tool to diagnose AI. We believe that the CFLCSA will become a vital image analysis parameter in the diagnosis of AI.
Methods
Participants
This study protocol was approved by the Catholic Kwandong Medical University Hospital Institutional Review Board. We reviewed the charts of patients who had visited the orthopedic ankle clinic with lateral ankle and foot pain from November 2016 to January 2019 and had undergone A-MRI within 6 months of the visit.
In order to meet the inclusion criteria for the AI group, patients must have: (I) had a history of lateral ankle sprain with persistent symptoms of AI, a positive anterior drawer test, and received non-operative treatment for at least 4 months; (II) experienced AI, pain, and buckling in those 4 months; and (III) no evidence of acute lower-limb or brain injury. Patients were excluded from this study if they met any of the following criteria: (I) peroneal pathology; (II) history of ankle surgery; (III) plantar fasciitis; (IV) neuromuscular atrophic disease; (V) hindfoot varus; or (VI) functional instability.
There were a total of 26 participants in the AI group; 17 (65.38%) were male, and 9 (34.62%) were female, with a mean age of 36.00±11.39 years (20–57 years) (Table 1). To contrast the CFLT and CFLCSA between subjects with and without AI, we recruited a normal control group comprised of participants with ankle and foot pain who wished to undergo A-MRI for an accurate diagnosis. There were no abnormal findings on A-MRI in the normal group.
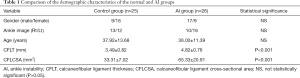
Full table
In the normal group, 25 participants (13 men and 12 women) were enrolled, with an average age of 37.92±13.68 years (20–67 years).
Imaging parameters
Using a 3.0T MRI system (MAGNETOM Skyra; Siemens Medical Solutions, Erlangen, Germany) and 3T Ingenia (Philips Medical, Eindhoven, Netherlands) scanners, we obtained coronal T1-weighted turbo spin echo images from all participants. The A-MR imaging parameters were as follows: slice plane axial, pulse sequence RF-spoiled steady-state gradient echo, the field of view 170 cm × 170 cm, repetition time 827.0 ms, echo time 11.0 ms, flip angle 35 degrees, slice thickness 3.50 mm, matrix size 384×250, several signals averaged =2, scan time 4 min 32 s, and 3> echo train length.
Image analysis
A board-certified pain specialist took the measurements of CFLT and CFLCSA with 15 years of experience, who was blinded to the ankle state. At the thickest visualization of the CFL, we obtained T1-weighted coronal A-MR images. Using the INFINITT picture archiving and communications system (PACS), we measured the CFLT and CFLCSA on A-MRI (INFINITT, Incheon, South Korea). The INFINITT PACS system is an award-winning, web-based, image analysis system offering streamlined workflow task viewing. The INFINITT PACS modules all run on a single-database platform and require a single sign-on, providing a single desktop utility. It also offers a diagnostic viewer with an enterprise imaging solution. Coronal T1-weighted A-MR image showed the tortuous shape of the CFL, which arises from the peroneus longus and brevis tendons to the tip of the lateral malleolus, and connects to a lateral calcaneal tubercle. The coronal planes were obtained to detect lateral ankle ligament pathology. We measured the CFLCSA as the cross-sectional area of the ligament margin of the CFL that was the most hypertrophied area in the A-MR images. We also measured the CFLT at the midline between the insertion and origin (Figure 1).
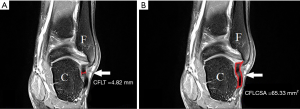
Statistical analysis
We compared the CFLT and CFLCSA between the AI and control group participants using unpaired t-tests. The cut-off points estimated the prediction value of the CFLT and CFLCSA in the diagnosis of AI, the area under the curve (AUC), specificity, and sensitivity. Values of P<0.05 were considered to be statistically significant. All statistical analyses were carried out using SPSS version 22.0 (SPSS, Inc., IBM, Chicago, IL, USA).
Results
Significant differences were not found in the demographic characteristics. The average CFLT was 3.49±0.82 mm in the normal subjects, and 4.82±0.76 mm in the AI group. The average CFLCSA was 33.31±7.02 mm2 in the normal subjects, and 65.33±20.91 mm2 in the AI group. The AI group had significantly greater CFLT (P<0.001) and CFLCSA (P<0.001) than did the normal group (Table 1). Table 2 shows the receiver operating characteristic (ROC) curves of CFLT used to predict AI in the normal subjects and AI group. The AUC of CFLT was 0.89 (95% CI, 0.79–0.99); the best cut-off score of the CFLT was 4.06 mm, with 76.9% sensitivity and 76.0% specificity (Figure 2). Table 3 shows the ROC curves of CFLCSA to predict AI in the normal subjects and AI groups. The AUC of CFLCSA was 0.94 (95% CI, 0.86–1.00). The best cut-off score of the CFLCSA was 43.85 mm2, with 92.3% sensitivity and 92.0% specificity (Figure 2).
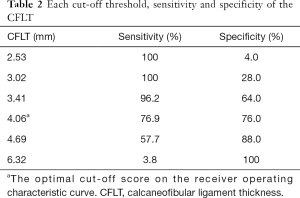
Full table
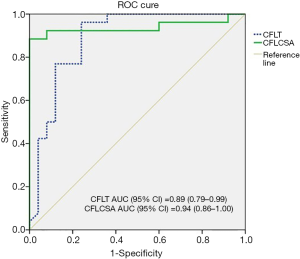
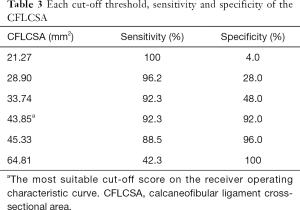
Full table
Discussion
This study aimed to discover the role of the CFLCSA in AI. We demonstrated that the CFLCSA had 92.3% sensitivity and 92.0% specificity for predicting AI. In contrast, the CFLT had 76.9% sensitivity, 76.0% specificity, and an AUC of 0.89. These results showed CFLCSA to be a better predictor of AI compared to CFLT.
Ankle sprains are one of the most common problems in physically active individuals, for whom the prevalence of ankle sprains is well established (1,14,15). Many patients who suffer from ankle sprain end up developing chronic AI, which is caused by a multitude of changes in the structural integrity of the ankle. Hypermobility or hypomobility of the ankle may lead to restriction of movement, and patients with chronic AI have been found to have less capacity for adjustment at the transfer of kinetic energy throughout the lower extremity (4,16). The precise causes of these malfunctions are still debated and are multifactorial. Thus, identifying lateral ankle ligament sprain is very important for establishing causation and deciding on treatment (17). Although most lateral ankle ligament sprains can be treated conservatively, the development of AI, characterized by the occurrence of repeated ankle injuries and persistence of pain, is frequent. AI limits daily physical activity, and it also leads to an increased risk of osteoarthritis; patients with severe discomfort occasionally require surgery to restore mechanical stability (18-21). Thus, the early diagnosis of AI is critical. Although multiple imaging techniques are available, including A-MRI, computed tomography (CT), ultrasonography, and stress view ankle radiography (12,22), the early diagnosis of AI is still not easy due to the lack of a reliable objective imaging parameters.
Lateral ankle ligaments include the ATFL, posterior talofibular ligament, and CFL (16). The CFL is cylindrical, lies deep to the peroneal tendons, and crosses 2 joints, where it acts as a subtalar joint stabilizer. The strain on the CFL is highest in dorsiflexion with an inverted ankle; thus, when the ankle is dorsiflexed, the CFL is the most frequently injured ligament in inversion ankle sprains. Even though isolated CFL tears are not common, CFL injuries in combination with ATFL tears are the second most common ligament damage pattern (7,23). The CFL runs inferiorly from the posterolateral aspect of the calcaneus to the lateral malleolus. For scanning of the CFL, the transducer is placed between the posterolateral aspect of the calcaneus and the lateral malleolus, and the CFL exhibits a curved probe course with hypoechogenicity. The thickening of the CFL has been considered to be a significant morphologic parameter of an inflammatory hypertrophic CFL. However, Abdeen et al. reported that ultrasound (US)-guided CFLT diagnostic sensitivity and specificity were 47% and 83%, respectively, with an accuracy of 72% (24).
Moreover, we found several difficulties associated with measuring CFLT. Previous research evaluated the CFLT using a single line at the middle point of the CFL. However, Kim et al. have insisted that A-MRI morphology of lateral ankle ligament injuries include a wavy or curved contour, ligament discontinuity, contour elongation, variable signal intensity, and ligament irregularities within the injury site (18). Therefore, 1 single measurement can result in erroneous measurements. We thought that the cross-sectional whole ligament area of the CFL might predict AI because CFLCSA measures the entire cross-sectional area of the CF and is unaffected by this erroneous measurement, in contrast to the CFLT. Eventually, we demonstrated that the CFLCSA was superior to the CLFT as a morphological measurement tool of AI. In the current original research, we demonstrated that the CFLCSA had 92.3% sensitivity and 92.0% specificity for predicting AI. In contrast, the CFLT had 76.9% sensitivity, 76.0% specificity, and an AUC of 0.89. These current results demonstrated that the CFLCSA is a better predictor of AI than is the CFLT (21).
This research has many limitations. First, the lateral ligament complex of the ankle comprises the ATFL, CFL, and the posterior talofibular ligament. However, we focused only on the CFL. Second, erroneous measurements of the CFLCSA and CFLT may happen on A-MRI. Even though we were trying to analyze these morphologic measurement methods and view the CFL at the coronal image section clearly, inhomogeneity of the coronal section images was still possible due to discrepancies in the cutting level or angle in the A-MRI resulting from individual anatomic differences and technical problems (25). Third, despite there being several alternative imaging diagnostic tools for evaluating AI, such as US examination (26,27), arthroscopy, manual anterior drawer test, or stress radiography, our research protocol analyzed only the measurement of the CFLCSA and CFLT using A-MRI. Fourth, CFLs were evaluated and classified using 2 categories; “absent or thin” and “thickened”. Multiple presentations, such as wavy, thin, signal changes, and attenuated, can be seen depending on the stage of the ligament injuries. However, we solely focused on thickened CFLs because the ligament undergoes an inflammatory and proliferative phase for several weeks in the post-injury recovery process, but may show thickening in an acute or subacute sprain. Fifth, we did not consider functional instability because of the subjective nature of functional instability findings that may vary between interpretations. We aimed to create objective morphological indicators. Despite these limitations, ours was the first research to record the association between CFLCSA and AI.
Conclusions
The goal of this research was to identify the role of the CFLCSA in AI. We demonstrated that the CFLCSA had high sensitivity (92.3%), high specificity (92.0%), and an AUC of 0.94 (95% CI, 0.86–1.00) for predicting AI. Current research data will support the results that the CFLCSA plays a significant role in identifying AI.
Acknowledgments
All authors express thanks to the International St. Mary’s Hospital.
Funding: None.
Footnote
Conflicts of Interest: All authors have completed the ICMJE uniform disclosure form (available at http://dx.doi.org/10.21037/qims-20-764). The authors have no conflicts of interest to declare.
Ethical Statement: This study conforms to the tenets of the Declaration of Helsinki. The Ethics committee approved this study protocol of the Catholic Kwandong Medical University Hospital Institutional Review Board.
Open Access Statement: This is an Open Access article distributed in accordance with the Creative Commons Attribution-NonCommercial-NoDeri 4.0 International License (CC BY-NC-ND 4.0), which permits the non-commercial replication and distribution of the article with the strict proviso that no changes or edits are made and the original work is properly cited (including links to both the formal publication through the relevant DOI and the license). See: https://creativecommons.org/licenses/by-nc-nd/4.0/.
References
- Akatsuka Y, Teramoto A, Takashima H, Watanabe K, Yamashita T. Morphological evaluation of the calcaneofibular ligament in different ankle positions using a three-dimensional MRI sequence. Surg Radiol Anat 2019;41:307-11. [Crossref] [PubMed]
- Best R, Mauch F, Fischer KM, Rueth J, Brueggemann GP. Radiographic monitoring of the distal insertion of the calcaneofibular ligament in anatomical reconstructions of ankle instabilities: A preliminary cadaveric study. Foot Ankle Surg 2015;21:245-9. [Crossref] [PubMed]
- Boykin RE, Ogunseinde B, McFeely ED, Nasreddine A, Kocher MS. Preliminary results of calcaneofibular ligament transfer for recurrent peroneal subluxation in children and adolescents. J Pediatr Orthop 2010;30:899-903. [Crossref] [PubMed]
- Hunt KJ, Pereira H, Kelley J, Anderson N, Fuld R, Baldini T, Kumparatana P, D'Hooghe P. The Role of Calcaneofibular Ligament Injury in Ankle Instability: Implications for Surgical Management. Am J Sports Med 2019;47:431-7. [Crossref] [PubMed]
- Sakakibara Y, Teramoto A, Takagi T, Yamakawa S, Okada Y, Shoji H, Kobayashi T, Fujimiya M, Fujie H, Watanabe K, Yamashita T. Effect of Initial Graft Tension During Calcaneofibular Ligament Reconstruction on Ankle Kinematics and Laxity. Am J Sports Med 2018;46:2935-41. [Crossref] [PubMed]
- Samoto N, Sugimoto K, Takaoka T, Fujita T, Kitada C, Takakura Y. Comparative results of conservative treatments for isolated anterior talofibular ligament (ATFL) injury and injury to both the ATFL and calcaneofibular ligament of the ankle as assessed by subtalar arthrography. J Orthop Sci 2007;12:49-54. [Crossref] [PubMed]
- Stenquist DS, Gonzalez TA, Tepolt FA, Kramer DE, Kocher MS. Calcaneofibular Ligament Transfer for Recurrent Peroneal Tendon Subluxation in Pediatric and Young Adult Patients. J Pediatr Orthop 2018;38:44-8. [Crossref] [PubMed]
- Sugimoto K, Kasanami R, Iwai M, Takakura Y, Kawate K. Achilles tendon rupture associated with injury of the calcaneofibular ligament. J Orthop Trauma 2003;17:534-5. [Crossref] [PubMed]
- Guerra-Pinto F, Côrte-Real N, Mota Gomes T, Silva MD, Consciência JG, Monzo M, Oliva XM. Rotational Instability after Anterior Talofibular and Calcaneofibular Ligament Section: The Experimental Basis for the Ankle Pivot Test. J Foot Ankle Surg 2018;57:1087-91. [Crossref] [PubMed]
- Wang W, Xu GH. Allograft tendon reconstruction of the anterior talofibular ligament and calcaneofibular Ligament in the treatment of chronic ankle instability. BMC Musculoskelet Disord 2017;18:150. [Crossref] [PubMed]
- Yoshizuka H, Shibata K, Asami T, Kuraoka A. Anatomical variation in the form of inter- and intra-individual laterality of the calcaneofibular ligament. Anat Sci Int 2018;93:495-501. [Crossref] [PubMed]
- Park HJ, Lee SY, Choi YJ, Hong HP, Park SJ, Park JH, Kim E. 3D isotropic T2-weighted fast spin echo (VISTA) versus 2D T2-weighted fast spin echo in evaluation of the calcaneofibular ligament in the oblique coronal plane. Clin Radiol 2017;72:176.e1-7. [Crossref] [PubMed]
- Edama M, Kageyama I, Kikumoto T, Nakamura M, Ito W, Nakamura E, Hirabayashi R, Takabayashi T, Inai T, Onishi H. The effects on calcaneofibular ligament function of differences in the angle of the calcaneofibular ligament with respect to the long axis of the fibula: a simulation study. J Foot Ankle Res 2017;10:60. [Crossref] [PubMed]
- Dowling A, Downey B, Green R, Reddy P, Wickham J. Anatomical and possible clinical relationships between the calcaneofibular ligament and peroneus brevis--a pilot study. Man Ther 2003;8:170-5. [Crossref] [PubMed]
- Draghi F, Gregoli B, Bortolotto C. Absence of elevation of fibular tendons during dorsal hyperflexion of the foot: a sign of loss of the calcaneofibular ligament. J Ultrasound Med 2014;33:1307-8. [Crossref] [PubMed]
- Kamada K, Watanabe S, Yamamoto H. Chronic subtalar instability due to insufficiency of the calcaneofibular ligament: a case report. Foot Ankle Int 2002;23:1135-7. [Crossref] [PubMed]
- Lee KT, Park YU, Kim JS, Kim JB, Kim KC, Kang SK. Long-term results after modified Brostrom procedure without calcaneofibular ligament reconstruction. Foot Ankle Int 2011;32:153-7. [Crossref] [PubMed]
- Kim WJ, Lee HS, Moon SI, Kim HS, Yeo ED, Kim YH, Seok Park E, Lee YK. Presence of Subfibular Ossicle Does Not Affect the Outcome of Arthroscopic Modified Broström Procedure for Chronic Lateral Ankle Instability. Arthroscopy 2019;35:953-60. [Crossref] [PubMed]
- Nakasa T, Nakashiro Md Ph DJ, Adachi Md Ph DN. Arthroscopic Repair of Anterior Talofibular Ligament Using the Pull-Out Technique for Chronic Ankle Instability: Case Report. J Foot Ankle Surg 2019;58:156-60. [Crossref] [PubMed]
- Torp DM, Thomas AC, Donovan L. External feedback during walking improves measures of plantar pressure in individuals with chronic ankle instability. Gait Posture 2019;67:236-41. [Crossref] [PubMed]
- Wikstrom EA, Song K, Tennant JN, Dederer KM, Paranjape C, Pietrosimone B. T1rho MRI of the talar articular cartilage is increased in those with chronic ankle instability. Osteoarthritis Cartilage 2019;27:646-9. [Crossref] [PubMed]
- Tao H, Hu Y, Qiao Y, Ma K, Yan X, Hua Y, Chen S. T2-mapping evaluation of early cartilage alteration of talus for chronic lateral ankle instability with isolated anterior talofibular ligament tear or combined with calcaneofibular ligament tear. J Magn Reson Imaging 2018;47:69-77. [Crossref] [PubMed]
- Kitsoulis P, Marini A, Pseftinakou A, Iliou K, Galani V, Paraskevas G. Morphological study of the calcaneofibular ligament in cadavers. Folia Morphol (Warsz) 2011;70:180-4. [PubMed]
- Abdeen R, Comfort P, Starbuck C, Nester C. Ultrasound Characteristics of Foot and Ankle Structures in Healthy, Coper, and Chronically Unstable Ankles. J Ultrasound Med 2019;38:917-26. [Crossref] [PubMed]
- Lee S, Cho HR, Yoo JS, Kim YU. The prognostic value of median nerve thickness in diagnosing carpal tunnel syndrome using magnetic resonance imaging: a pilot study. Korean J Pain 2020;33:54-9. [Crossref] [PubMed]
- Ekinci M, Ciftci B, Alici HA, Ahiskalioglu A. Ultrasound-guided rhomboid intercostal block effectively manages myofascial pain. Korean J Anesthesiol 2020. Epub ahead of print. [Crossref] [PubMed]
- Kim TE, Tsui BCH. Simulation-based ultrasound-guided regional anesthesia curriculum for anesthesiology residents. Korean J Anesthesiol 2019;72:13-23. [Crossref] [PubMed]