Impact of ocular magnification on retinal and choriocapillaris blood flow quantification in myopia with swept-source optical coherence tomography angiography
Introduction
Myopia is a common cause of vision impairment, projecting to affect approximately 50% of the world’s population by 2050 (1). Although the etiology of myopia has not been fully elucidated, lower ocular blood flow has been consistently reported in myopic eyes regardless of the imaging modalities (2). Optical coherence tomography angiography (OCTA) is a novel noninvasive modality that provides images with capillary-level resolution of the retinal vasculature (3,4). Moreover, OCTA has proven clinically useful for the assessment and diagnosis of myopic choroidal neovascularization (5,6).
OCTA is becoming increasingly popular in the investigation of the retinal and choroidal blood flow in myopia (7-12). OCTA parameters such as retinal vessel density (RVD) and choriocapillaris (CC) flow deficits provide information about the perfusion status of the retinal vasculature and CC. In OCTA studies, decreased, unchanged, or increased RVD has been reported in myopic eyes in comparison to emmetropic eyes (7-12). However, different from emmetropia, eyes with myopia usually have longer axial length, which may induce significant ocular magnification on OCTA images. According to the Littman (t = p × q × s) and the modified Bennett [q = 0.01306 × (x – 1.82)] formulae, the true size t of a retinal feature increases with axial length when imaging with the same fundus camera optical system (13,14). Currently, commercial optical coherence tomography (OCT) devices do not implement the function to correct image magnification. Therefore, it is essential to manually correct image magnification individually in myopic subjects for accurate measurements of OCT and OCTA parameters. It has been demonstrated that ocular magnification (attributable to axial length) significantly affects the OCT measurements including optic disc size and retinal nerve fiber layer thickness in myopia (15,16). However, there is limited literature has focused on the magnification error of quantitative OCTA measurements in myopic eyes. The purpose of this study is to investigate the potential impact of ocular magnification on retinal and CC blood flow quantification using OCTA in myopic eyes.
Methods
This study was approved by the Capital Medical University (Beijing, China) and the University of Washington (Seattle, USA). The study design followed the tenets of the Declaration of Helsinki. Signed informed consent was obtained from each subject before inclusion in the study.
Study population
Subjects with myopia who manifested spherical equivalent (SE) refractive error of –0.50 diopters (D) or less were prospectively enrolled in this study. Study eligibility criteria included a normal anterior segment with sufficiently clear media and a best-corrected visual acuity (BCVA) of 20/200 or better to allow imaging. Exclusion criteria included any coexisting or previous ocular disease that may confound measurements of the retinal and choroidal blood flow, such as glaucoma, optic nerve disease, chorioretinal pathology other than myopic chorioretinal atrophy. Each subject underwent comprehensive ophthalmic examination including assessment of BCVA, slit-lamp examination, refractive error correction, intraocular pressure measurements with Goldmann applanation tonometry, dilated funduscopy, and ocular biometry (IOLMaster® 500; Carl Zeiss Meditec, Jena, Germany). All biometric measurements and imaging scans were performed by trained operators at the Beijing Tongren Eye Center, Capital Medical University, China.
Swept-source OCTA (SS-OCTA) imaging and processing
SS-OCTA data were acquired using the PLEX® Elite 9000 SS-OCT system (Carl Zeiss Meditec, Dublin, CA, USA) with a central wavelength of 1,060 nm and a speed of 100-kHz A-scan rate. The 6×6 mm2 scanning protocol was used for imaging, with 500 A-lines per B-scan and 500 B-scans with two repeated scans in each fixed location. OCTA data were generated using complex optical microangiography (OMAGc) algorithm (17). Per recommendation from the manufacturer, scans with signal strength less than seven or obvious artifacts were excluded from the analyses.
Retinal and CC slabs were segmented using a validated semi-automated segmentation method (18). The retinal vasculature slab was defined as a slab extending from the inner limiting membrane to the outer plexiform layer. Following the guidelines in the previous study (19), the CC slab in myopic eyes was defined as a slab extending from approximately 4 to 14 µm below the outer boundary of Bruch’s membrane (BM). The CC en face flow images were generated using the sum projection, followed by removal of the retinal vessel projection artifacts (20) and compensation for the CC signal strength due to heterogeneous optical properties of the retinal pigment epithelium (21) before the identification of CC flow deficits (FDs). All images were analyzed with MATLAB (R2017b; MathWorks, Inc., Natick, MA, USA).
The retinal vessel area density (RVAD) and retinal vessel skeleton density (RVSD) were calculated from the en face retinal flow images using our previously published algorithm (22). The percentage of CC FDs (CC FD%) was calculated using a previously described global thresholding method, followed by removal of the CC FDs with an equivalent diameter smaller than the normal intercapillary distance of 24 µm (21,23). All the parameters were quantified within a 5-mm-diameter circle centered on the fovea in the en face OCTA images (Figure 1). Examples of the en face retinal flow image, the en face CC flow image, and the quantitative maps are shown in Figure 2.
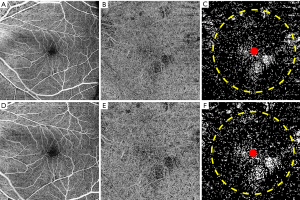
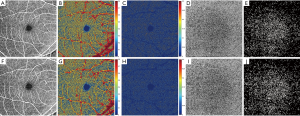
Adjustment for image magnification
For the estimation of corrected or “true” measurements, original OCTA images were adjusted by a scaling factor defined by the Littman and the modified Bennett formulae (13,14). According to the Littman formula, the relationship between the true size t of a retinal feature and its measured size s on OCTA image can be expressed as t = p × q × s, where p is the magnification factor related to the OCT system, and q is the magnification factor related to the eye (13). The factor q can be determined by the Bennett formula: q = 0.01306 × (x – 1.82), where x is the axial length (14). By using the default axial length 24.46 mm adopted by the PLEX® Elite 9000 system for a magnification of 1 (i.e., t = s), p can be calculated as 1/[0.01306×(24.46–1.82)]=3.382. Therefore, the scaling factor of the OCTA for adjustment of the ocular magnification can be calculated as: t/s = 3.382 × 0.01306 × (x – 1.82). The en face OCTA image of the myopic eye was then adjusted by this scaling factor for further quantification (Figure 2F,G,H,I,J).
Statistical analysis
Statistical analysis was conducted using the commercial statistical software (IBM SPSS Statistics version 25.0; IBM Corporation, Armonk, NY, USA). Variable normality was inspected using histograms and the Shapiro-Wilk test. The OCTA measurements before and after magnification correction were compared with paired t-test or Wilcoxon matched-pairs signed-rank test as appropriate. For all comparisons, a P value of less than 0.05 was considered statistically significant.
Results
Demographics and clinical characteristics
Twenty-eight myopic eyes were analyzed in this study. The myopic eyes were further classified as non-high myopia (axial length <26.5 mm) in 12 eyes (43%) and high myopia (axial length ≥26.5 mm) in 16 eyes (57%). Baseline characteristics are listed in Table 1.

Full table
Comparison of OCTA measurements before and after image magnification correction
Based on the Shapiro-Wilk test, the corrected and uncorrected RVSD and CC FD% measurements were compared using the Wilcoxon matched-pairs signed-rank test. The corrected and uncorrected RVAD measurements were compared using the paired t-test. Box plots of the RVAD, RVSD, and CC FD% measurements are shown in Figure 3, with brackets indicating statistically significant differences between the corrected and uncorrected measurements. The mean and standard deviation values of all OCTA measurements are shown in Table 2. The mean corrected RVAD and RVSD were significantly lower than the uncorrected measurements in all groups (all P<0.01; Figure 3A,B). In eyes with high myopia, the mean corrected CC FD% was significantly higher than the uncorrected CC FD% (14.9%±4.9% vs. 14.2%±4.5%, P=0.009; Figure 3C). However, no statistically significant difference was observed between the corrected and uncorrected CC FD% measurements in eyes with non-high myopia (11.7%±5.8% vs. 11.5%±5.8%, P=0.133; Figure 3C).
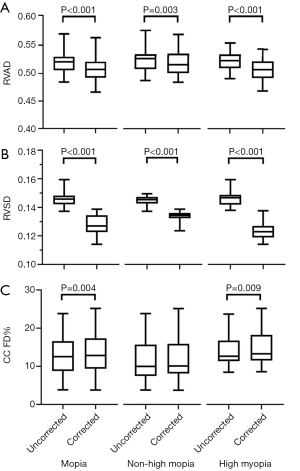
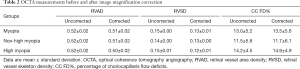
Full table
Discussion
The present study demonstrated that both the retinal vascular measurements and the CC FD measurements evaluated from corrected OCTA images were significantly different from those from uncorrected images in myopic eyes. The corrected RVAD and RVSD measurements were significantly lower than the uncorrected measurements in myopic eyes. Meanwhile, the corrected CC FD% measurements were significantly higher than the uncorrected measurements in highly myopic eyes.
The impact of image magnification on RVAD measurements with OCTA in healthy subjects has been reported by Sampson et al. (24). In their study, 51% of the eyes exhibited more than 5% correction of the foveal RVAD measurements, suggesting that ocular magnification may affect foveal RVAD quantification. However, the RVAD was quantified within a 1-mm circle and a 1-mm rim centered on the fovea in their study, which limited a further interpretation of the magnification effect on RVAD measurements in larger regions. Moreover, as a more sensitive parameter for assessing retinal capillary perfusion (22), the magnification effect on RVSD measurement was not assessed.
In the current study, we evaluated both RVAD and RVSD measurements from the 6×6 mm2 OCTA scans in myopic eyes. Our results showed that both the corrected RVAD and the corrected RVSD measurements significantly decreased after correcting ocular magnification. In OCTA, the scan area of 6×6 mm2 was calculated from the linear distance on the surface of the retina subtended by an angular size of approximately 20°×20° using the default axial length adopted by the OCT system. In myopic eyes, a longer axial length would result in a larger retinal area being scanned than the nominal 6×6 mm2 area, which makes the retinal vascular network appear artificially denser.
For accurate comparison of the interocular OCTA parameters, RVAD and RVSD were quantified within a 5-mm-diameter circle centered on the fovea in the present study. After correcting magnification in myopic eyes with longer axial length, the foveal avascular zone occupied a relatively larger portion in the 5-mm-diameter circular region, resulting in decreased RVSD measurements from the corrected images compared with the uncorrected ones. Meanwhile, parts of the large-caliber vessels were also excluded from the measuring circle after magnification correction. As a result, the corrected RVAD was lower than the uncorrected RVAD in myopic eyes. Therefore, for more accurate quantification of RVAD and RVSD in myopic eyes, the ocular magnification effect should be taken into consideration.
We also evaluated the magnification effect on the CC blood flow quantification since abnormal CC flow has been reported in myopic eyes (12,25). In the current study, CC en face flow images were generated from a 10-µm-thickness slab below BM based on CC thickness measurements in myopic eyes using our previously described algorithm (unpublished data) (26). No statistically significant difference was observed between the corrected and uncorrected CC FD% measurements in eyes with non-high myopia. In our preliminary observation, CC FDs in non-highly myopic eyes were basically uniformly distributed in the central 5-mm-diameter circular region. As a relative value, CC FD% measurements did not change before and after ocular magnification correction in eyes with non-high myopia. However, the current study showed that the mean corrected CC FD% was significantly higher than the uncorrected CC FD% in eyes with high myopia. In highly myopic eyes, CC FDs might merge into a large area where CC was completely lost. When these lesions were located in or around the measuring circular region, significant differences were observed between the corrected and uncorrected CC FD% measurements (Figure 1). Consequently, when calculating CC FD% in highly myopic eyes, ocular magnification correction is recommended.
While we have demonstrated that magnification correction should be considered for more accurate quantification of OCTA images in myopic eyes, this consideration would be equally applicable to hyperopic eyes. In eyes with hyperopia, a shorter axial length will result in a smaller retinal area being scanned than the nominal defined area in OCT. As a result, the true values of RVAD and RVSD should have been higher than the uncorrected values in hyperopic eyes. Furthermore, magnification correction is also important for accurate assessment of longitudinal changes in retinal and CC blood flow in eyes undergoing axial growth. The decrease of RVAD or RVSD may not be detected if OCTA measurements were not corrected with elongated axial lengths in myopic eyes. When it comes to myopic eyes or hyperopic eyes with retinal or CC pathologies, changes in RVAD, RVSD, and CC FD measurements before and after magnification correction are even more complicated. Therefore, it is recommended to correct all quantification values derived from OCTA for accurate and reliable assessments of the retinal and CC blood flow in all ametropic eyes.
We acknowledged several limitations to our study. First, due to the poor fixation and low vision status of some myopic subjects, not all OCTA images are optimal for quantitative analysis. Therefore, our study is limited by a relatively small sample size. However, this study still included myopic eyes with a broad axial length ranging from 24.50 to 33.22 mm, which demonstrated the magnification effect on retinal and CC blood flow quantification in low myopia, moderate myopia, high myopia, and extremely high myopia. Second, in the current study, image magnification error was corrected based on Bennett’s formula with an abbreviated axial length. For more accurate correction, detailed calculations utilizing the axial length, keratometry, ametropia, anterior chamber depth, as well as the lens thickness might be considered (14). However, measurements of multiple variables might introduce multiple measurement errors in the calculation. Since Bennett’s formula has been demonstrated differs little from more detailed calculations (27), we adopted Bennett’s method as our magnification correction algorithm.
In conclusion, the current study shows that ocular magnification significantly affects the results of retinal and CC blood flow quantification with OCTA in myopic eyes. For accurate determination of the OCTA derived parameters in myopia as well as other ocular disease investigations, magnification correction should be taken into consideration.
Acknowledgments
Funding: This work was supported by Carl Zeiss Meditec; and an unrestricted grant from the Research to Prevent Blindness. The funding organizations had no role in the design or conduct of this research.
Footnote
Provenance and Peer Review: With the arrangement by the Guest Editors and the editorial office, this article has been reviewed by external peers.
Conflicts of Interest: All authors have completed the ICMJE uniform disclosure form (available at http://dx.doi.org/10.21037/qims-20-1011). The special issue “Advanced Optical Imaging in Biomedicine” was commissioned by the editorial office without any funding or sponsorship. RKW served as the unpaid Guest Editor of the specail issue and serves as an unpaid Deputy Editor of Quantitative Imaging in Medicine and Surgery. RKW received research support from Carl Zeiss Meditec and an innovative research award from Research to Prevent Blindness. RKW is a consultant to Carl Zeiss Meditec. The authors have no other conflicts of interest to declare.
Ethical Statement: This study was approved by the Capital Medical University (Beijing, China) and the University of Washington (Seattle, USA). The study design followed the tenets of the Declaration of Helsinki. Signed informed consent was obtained from each subject before inclusion in the study.
Open Access Statement: This is an Open Access article distributed in accordance with the Creative Commons Attribution-NonCommercial-NoDerivs 4.0 International License (CC BY-NC-ND 4.0), which permits the non-commercial replication and distribution of the article with the strict proviso that no changes or edits are made and the original work is properly cited (including links to both the formal publication through the relevant DOI and the license). See: https://creativecommons.org/licenses/by-nc-nd/4.0/.
References
- Resnikoff S, Jonas JB, Friedman D, He M, Jong M, Nichols JJ, Ohno-Matsui K, Smith EL III, Wildsoet CF, Taylor HR, Wolffsohn JS, Wong TY. Myopia - a 21st century public health issue. Invest Ophthalmol Vis Sci 2019.60. Mi-Mii. [PubMed]
- Grudzińska E, Modrzejewska M. Modern diagnostic techniques for the assessment of ocular blood flow in myopia: current state of knowledge. J Ophthalmol 2018;2018:4694789. [Crossref] [PubMed]
- Kashani AH, Chen CL, Gahm JK, Zheng F, Richter GM, Rosenfeld PJ, Shi YG, Wang RK. Optical coherence tomography angiography: a comprehensive review of current methods and clinical applications. Prog Retin Eye Res 2017;60:66-100. [Crossref] [PubMed]
- Chen CL, Wang RK. Optical coherence tomography based angiography. Biomed Opt Express 2017;8:1056-82. [Crossref] [PubMed]
- Ohno-Matsui K, Ikuno Y, Lai TYY, Gemmy Cheung CM. Diagnosis and treatment guideline for myopic choroidal neovascularization due to pathologic myopia. Prog Retin Eye Res 2018;63:92-106. [Crossref] [PubMed]
- Ishida T, Watanabe T, Yokoi T, Shinohara K, Ohno-Matsui K. Possible connection of short posterior ciliary arteries to choroidal neovascularisations in eyes with pathologic myopia. Br J Ophthalmol 2019;103:457-62. [Crossref] [PubMed]
- Li M, Yang Y, Jiang H, Gregori G, Roisman L, Zheng F, Ke B, Qu D, Wang J. Retinal microvascular network and microcirculation assessments in high myopia. Am J Ophthalmol 2017;174:56-67. [Crossref] [PubMed]
- Yang Y, Wang J, Jiang H, Yang X, Feng L, Hu L, Wang L, Lu F, Shen M. Retinal microvasculature alteration in high myopia. Invest Ophthalmol Vis Sci 2016;57:6020-30. [Crossref] [PubMed]
- Sung MS, Lee TH, Heo H, Park SW. Association between optic nerve head deformation and retinal microvasculature in high myopia. Am J Ophthalmol 2018;188:81-90. [Crossref] [PubMed]
- Milani P, Montesano G, Rossetti L, Bergamini F, Pece A. Vessel density, retinal thickness, and choriocapillaris vascular flow in myopic eyes on OCT angiography. Graefes Arch Clin Exp Ophthalmol 2018;256:1419-27. [Crossref] [PubMed]
- Al-Sheikh M, Phasukkijwatana N, Dolz-Marco R, Rahimi M, Iafe NA, Freund KB, Sadda SR, Sarraf D. Quantitative OCT angiography of the retinal microvasculature and the choriocapillaris in myopic eyes. Invest Ophthalmol Vis Sci 2017;58:2063-9. [Crossref] [PubMed]
- Su L, Ji YS, Tong N, Sarraf D, He X, Sun X, Xu X, Sadda SR. Quantitative assessment of the retinal microvasculature and choriocapillaris in myopic patients using swept-source optical coherence tomography angiography. Graefes Arch Clin Exp Ophthalmol 2020;258:1173-80. [Crossref] [PubMed]
- Littmann H. Determination of the real size of an object on the fundus of the living eye. Klin Monbl Augenheilkd 1982;180:286-9. [Crossref] [PubMed]
- Bennett AG, Rudnicka AR, Edgar DF. Improvements on Littmann's method of determining the size of retinal features by fundus photography. Graefes Arch Clin Exp Ophthalmol 1994;232:361-7. [Crossref] [PubMed]
- Leung CK, Cheng AC, Chong KK, Leung KS, Mohamed S, Lau CS, Cheung CY, Chu GC, Lai RY, Pang CC, Lam DS. Optic disc measurements in myopia with optical coherence tomography and confocal scanning laser ophthalmoscopy. Invest Ophthalmol Vis Sci 2007;48:3178-83. [Crossref] [PubMed]
- Kang SH, Hong SW, Im SK, Lee SH, Ahn MD. Effect of myopia on the thickness of the retinal nerve fiber layer measured by Cirrus HD optical coherence tomography. Invest Ophthalmol Vis Sci 2010;51:4075-83. [Crossref] [PubMed]
- Wang RK, An L, Francis P, Wilson DJ. Depth-resolved imaging of capillary networks in retina and choroid using ultrahigh sensitive optical microangiography. Opt Lett 2010;35:1467-9. [Crossref] [PubMed]
- Yin X, Chao JR, Wang RK. User-guided segmentation for volumetric retinal optical coherence tomography images. J Biomed Opt 2014;19:086020. [Crossref] [PubMed]
- Chu Z, Gregori G, Rosenfeld PJ, Wang RK. Quantification of choriocapillaris with optical coherence tomography angiography: a comparison study. Am J Ophthalmol 2019;208:111-23. [Crossref] [PubMed]
- Zhang A, Zhang Q, Wang RK. Minimizing projection artifacts for accurate presentation of choroidal neovascularization in OCT micro-angiography. Biomed Opt Express 2015;6:4130-43. [Crossref] [PubMed]
- Zhang Q, Zheng F, Motulsky EH, Gregori G, Chu Z, Chen CL, Li C, de Sisternes L, Durbin M, Rosenfeld PJ, Wang RK. A novel strategy for quantifying choriocapillaris flow voids using swept-source OCT angiography. Invest Ophthalmol Vis Sci 2018;59:203-11. [Crossref] [PubMed]
- Chu Z, Lin J, Gao C, Xin C, Zhang Q, Chen CL, Roisman L, Gregori G, Rosenfeld PJ, Wang RK. Quantitative assessment of the retinal microvasculature using optical coherence tomography angiography. J Biomed Opt 2016;21:66008. [Crossref] [PubMed]
- Zhang Q, Shi Y, Zhou H, Gregori G, Chu Z, Zheng F, Motulsky EH, de Sisternes L, Durbin M, Rosenfeld PJ, Wang RK. Accurate estimation of choriocapillaris flow deficits beyond normal intercapillary spacing with swept source OCT angiography. Quant Imaging Med Surg 2018;8:658-66. [Crossref] [PubMed]
- Sampson DM, Gong P, An D, Menghini M, Hansen A, Mackey DA, Sampson DD, Chen FK. Axial length variation impacts on superficial retinal vessel density and foveal avascular zone area measurements using optical coherence tomography angiography. Invest Ophthalmol Vis Sci 2017;58:3065-72. [Crossref] [PubMed]
- Sayanagi K, Ikuno Y, Uematsu S, Nishida K. Features of the choriocapillaris in myopic maculopathy identified by optical coherence tomography angiography. Br J Ophthalmol 2017;101:1524-9. [Crossref] [PubMed]
- Zhou H, Dai Y, Gregori G, Rosenfeld PR, Duncan JL, Schwartz DM, Wang RK. Automated morphometric measurement of the retinal pigment epithelium complex and choriocapillaris using swept source OCT. Biomed Opt Express 2020;11:1834-50. [Crossref] [PubMed]
- Garway-Heath DF, Rudnicka AR, Lowe T, Foster PJ, Fitzke FW, Hitchings RA. Measurement of optic disc size: equivalence of methods to correct for ocular magnification. Br J Ophthalmol 1998;82:643-9. [Crossref] [PubMed]