Anterior segment optical coherence tomography evaluation of ocular graft-versus-host disease: a case study
Introduction
Graft-versus-host disease (GVHD) is a major complication following allogeneic hematopoietic stem cell transplantation (aHSCT), occurring in 25-70% of patients, due to the reaction of transplanted immune cells against host’s body cells (1-3). GVHD severely limits the full potential of aHSCT and is still one of the leading causes of morbidity and mortality in aHSCT.
Usually, GVHD involves several organ systems, such as skin, liver, gastrointestinal tract, mouth and eye (4,5). Among these target organs, ocular involvement occurs in ~60% of GVHD patients (1,3,4), and affects almost all parts of the eye, particularly cornea, conjunctiva, lids and lacrimal gland, resulting in a wide spectrum of ocular complications (1,2,5-8). Although ocular involvement is generally not a fatal problem, it has been well recognized that ocular GVHD causes considerable suffering and significantly impairs the quality of life (1-4,6-9).
The frequent and potentially severe ocular involvement in GVHD patients necessitates a full ocular evaluation (pre- and post-transplantation) of these patients for early recognition and timely treatment prior to the onset of other complications. Currently, the diagnosis of ocular GVHD is mainly based on the presence of ocular manifestations such as new-onset dry, gritty, or painful eyes, keratoconjunctivitis sicca, photophobia and punctate keratopathy, and is commonly confirmed by invasive biopsy or other relevant tests (5). Whereas, such clinical manifestations usually occur in a late phase of ocular GVHD and the recognition is more or less a subjective procedure. On the other hand, the invasive nature of biopsy precludes its use in the longitudinal assessment of disease progression and treatment response. Thus, a non-invasive examining method capable of detecting early changes is particularly attractive in the ocular GVHD.
In addition, a practical treatment guideline is necessary for diagnosing and treating ocular GVHD. Due to the frequent involvement of conjunctiva in the ocular GVHD, Jabs and his colleagues proposed a conjunctival GVHD staging system (8) and then refined with additional examination experience (4). This staging system mainly outlines the development of conjunctival GVHD. However, the involvement of other ocular GVHD manifestations is not considered. This is largely due to the current lack of appropriate assessment tools.
Optical coherence tomography (OCT) is an imaging tool capable of providing micrometer-resolution (10-12). Based on these attributes, OCT is considered particularly suited for ocular imaging and is currently part of the clinical standard of patient care in ophthalmology.
The aim of this pilot case study is to explore the feasibility of using OCT to better evaluate ocular GVHD. Based on the common manifestations of ocular GVHD, the OCT imaging is mainly focused on four regions of interests (ROIs), including conjunctiva, cornea, eyelids and tear meniscus.
Materials and methods
The study was approved by the Institutional Review Board at the Fred Hutchinson Cancer Research Center and the University of Washington. The patient was recruited with prior informed consent. Information from the GVHD patient with ocular involvement is summarized in Table 1. OCT imaging was performed prior the bandage contact lens trial (GVHD0), and then two follow-up examinations at 2-week interval were performed for assessing treatment response (GVHD2 & GVHD4).
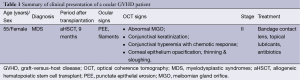
Full table
We used an anterior segment OCT (AS-OCT) system in this study. The system was reported in detail in reference (13), except the light source employed. The light source was a broadband superluminescent diode (SLD) with a central wavelength of 1,340 nm and a spectral bandwidth of 110 nm, providing an axial resolution of ~7.2 µm in air. An objective lens with a 50 mm focal length was used in the sample arm to deliver the probing light to the ROIs at the cornea, yielding a measured lateral resolution of ~16 µm. A brief schematic representation of the OCT system is presented in Figure 1.
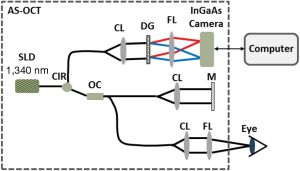
We focused OCT imaging on four selected ROIs including eyelid, tear meniscus, cornea and conjunctiva. Specifically, for eyelids and tear menisci, the OCT imaging was performed at the inferior portion of the eye, while for cornea it was at the central cornea. The scanning protocol was optimized for real-time, high-definition microstructural imaging, in which the data volume consists of 512 (depth pixels) × 512 (A lines) × 512 (B-frames) covering 3×4.5×4.5 mm3 that required an acquisition time of ~3.6 seconds. For conjunctival region, the OCT imaging was performed at the nasal portion of eye. This later scanning protocol was optimized for imaging microstructure, blood and lymphatic vasculature in parallel as detailed in reference (14). The data volume consists of 512 (depth pixels) × 360 (A lines) × 1,000 (B-frames) covering 3×4.5×4.5 mm3 that required an acquisition time of ~5 seconds. The 1,000 B-frames was captured 200 sampling positions with five repeated scans at each position in order to achieve the imaging of blood perfusion within conjunctival tissue beds by the use of optical microangiography (OMAG) (15-18). In addition, because the lymphatic vessel lumen appears as low-signal region in the OCT image, a semi-automatic segmentation software (19) was use to segment the three dimensional (3D) lymphatic vasculatures out from the surrounding structures (20,21).
Results
Normal subject (Figures 2-5)
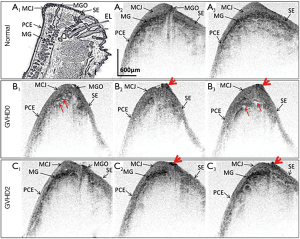
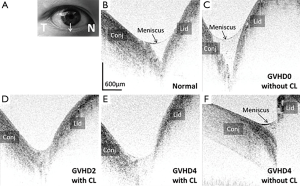
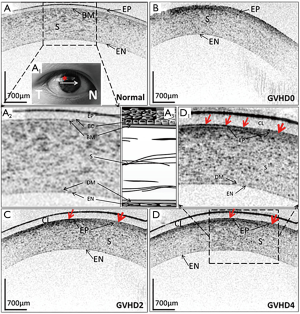
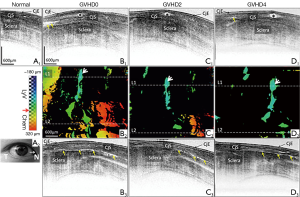
A normal subject was scanned by AS-OCT as well. The protocol of the normal subject was similar to the study patient. The representative results were respectively reported in Figure 2A2,A3, Figure 3B, Figure 4A and Figure 5A1 for comparing with GVHD data.
GVHD case
A 55-year-old woman with myelodysplastic syndrome (MDS) who underwent aHSCT in December 2011 suffered from severe photosensitivity and gritty sensation since May 2012. Bilateral diffuse punctate epithelial erosions (PEEs) and a few corneal filaments were disclosed at her first visit to ophthalmic clinic. A series of AS-OCT imaging were taken after ocular examination. The AS-OCT images acquired from the normal subjects indicate that OCT is able to delineate meibomian gland orifice (MGO) (Figure 2A2), and the meibomian gland (MG) appears as a high-scattering region beneath palpebral conjunctival epithelium (PCE) and mucocutaneous junction (MCJ) (Figure 2A2,A3). However, the OCT images acquired from the ocular GVHD patient showed less visible MGO than normal subjects, and there appeared some scarring-like tissue around MGO in her first two visits (before treatment, GVHD0, Figure 2B and 2-week follow-up visit, GVHD2, Figure 2C). In addition, the MG was presented more inflamed and appeared as low-scattering regions in the OCT images acquired at her first visit (Figure 2B); however, this inflammation was somewhat relieved at her 2-week follow-up visit (Figure 2C). The image appearance of eyelid at her 4-week follow-up visit was almost the same as her 2-week follow-up visit (GVHD2).
In contrast to its clear and transparent appearance in normal subjects (Figure 3B), the tear meniscus in the GVHD patient was visiblely turbid at the first visit prior to treatment (Figure 3C). During the 2-week and 4-week follow-up visits, tear meniscus significantly reduced while wearing contact lens (Figure 3D,F). After taking off contact lens at the 4-week follow-up visit, the turbid tear meniscus appeared again in OCT cross-sectional image (Figure 3F).
As shown in Figure 4, compared to the normal subject (refer to Figure 4A,A2), the corneal epithelium of the GVHD patient showed less transparency, mild opacity and high scattering with less smooth corneal surface at her first visit prior to treatment (refer to Figure 4B). Bilateral bandage contact lenses were placed after the first ocular examination. The patient was also received topical antibiotics and lubricants at the time of imaging. During the 2-week and 4-week follow-up visits (refer to Figure 4C,D,D1), the OCT images indicate that the original less-transparent corneal epithelium becomes thinned and sloughing in the region marked by the star in Figure 4A1, which might be lightly scraped off by contact lens clinically.
As reported in Figure 5, compared to that of normal subject (Figure 5A1), the conjunctival image of GVHD shows much higher OCT signal on the surface which is most likely due to the conjunctival keratinization. In addition, the conjunctival lymphatic vessels are dilated in GVHD patient compared to those in normal subject (as marked by stars in Figure 5B1,C1,D1). And the chemotic response can be observed around the boundary between conjunctiva and sclera (as indicated by thin arrows in Figure 5B1,B3,C3,D1,D3). Based on the 3D conjunctival OCT images, the lymphatic vasculature and chemosis can be differentiated from the surrounding tissues, as shown by the depth-encoded projection views in Figure 5B2,C2,D2, where the yellow and orange colors are primarily corresponding to chemosis, and blue and green colors are mainly corresponding to lymphatic vessels. It is noticed that much more obvious conjunctival chemosis was present at the first visit prior to treatment, and it was reduced significantly during the 2-week and 4-week follow-up visits after adequate treatment. However, the conjunctival hyperemia still existed at her 4-week follow-up visit (Figure 6) as shown by the dense blood vasculature in Figure 6D.
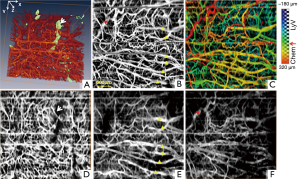
Discussion and conclusions
Non-invasive OCT imaging findings in our patient showed scar formation of the MGO (Figure 2), confirming the clinical observations on ocular GVHD patients. It is known that the tear film, which plays an important role in maintaining ocular surface and clear vision, is mainly composed of three physiological layers: lipid layer by MG, aqueous layer by lacrimal gland and mucin layer by conjunctival goblet cells. Our findings on the tear meniscus (Figure 3) demonstrated a different relationship between the tear production and severity of ocular surface pathology as currently characterized by Schirmer’s test (measuring tear production): we observed higher tear meniscus during the initial exam while the ocular surface irritation caused reflex tearing, in contrast to the conventional believe that GVHD patients lacks tears. This further confirmed that Schirmer’s test, the current standard for ocular GVHD staging, has not been accurate in correlating with clinical findings.
According to the results of this pilot study, we found MG dysfunction possibly appears at the early stage, affecting the lipid/oil secretion, while the tear/aqueous secretion by lacrimal gland is still functioning. The lack of the MG secretions would accelerate the evaporation of tear film and influence the functions of tear film, which together might be responsible for the above-mentioned disorders of cornea (refer to Figure 4) and conjunctiva (refer to Figures 5,6). Our non-invasive in vivo imaging in GVHD patient has demonstrated for the first time the inflammatory changes of the ocular surface vascularture and chemosis in response to treatment with bandage contact lenses. AS-OCT offers unique advantage of precise dynamic analysis of the ocular surface system without physical disturbance (compared to Schirmer’s). This information will be invaluable for the fundimental understanding of the pathophysiology of the GVHD.
Referring to the ocular GVHD conjunctival staging system proposed by Jabs et al. (8) and combining with the clinical manifestations, this ocular patient is most likely corresponding to stage II GVHD.
“The earlier the detection, the better the prognosis would be”. Ocular GVHD is also one of the ocular surface disorders that follow this rule. The promising results from this pilot case study demonstrates the feasibility of using OCT for ocular GVHD examination at early stages. The detailed information extracted from OCT, including MG, tear film, corneal epithelium, and conjunctival vasculature, can potentionally be used for enriching the current ocular GVHD staging system and the clinical management for GVHD treatment. In the future, more pre- and post-aHSCT with or without ocular GVHD cases will be recruited for OCT imaging and statistical analysis to investigate the significance between these reported OCT findings and ocular GVHD.
Acknowledgements
This work was supported in part by the Lions Research Fund, the Guiliani Family Foundation, Research to Prevent Blindness and the Coulter translational research grant.
Disclosure: The authors declare no conflict of interest.
References
- Franklin RM, Kenyon KR, Tutschka PJ, Saral R, Green WR, Santos GW. Ocular manifestations of graft-vs-host disease. Ophthalmology 1983;90:4-13. [PubMed]
- Jack MK, Jack GM, Sale GE, Shulman HM, Sullivan KM. Ocular manifestations of graft-v-host disease. Arch Ophthalmol 1983;101:1080-4. [PubMed]
- Anderson NG, Regillo C. Ocular manifestations of graft versus host disease. Curr Opin Ophthalmol 2004;15:503-7. [PubMed]
- Riemens A, te Boome L, Imhof S, Kuball J, Rothova A. Current insights into ocular graft-versus-host disease. Curr Opin Ophthalmol 2010;21:485-94. [PubMed]
- Filipovich AH. Diagnosis and manifestations of chronic graft-versus-host disease. Best Pract Res Clin Haematol 2008;21:251-7. [PubMed]
- Hirst LW, Jabs DA, Tutschka PJ, Green WR, Santos GW. The eye in bone marrow transplantation. I. Clinical study. Arch Ophthalmol 1983;101:580-4. [PubMed]
- Jabs DA, Hirst LW, Green WR, Tutschka PJ, Santos GW, Beschorner WE. The eye in bone marrow transplantation. II. Histopathology. Arch Ophthalmol 1983;101:585-90. [PubMed]
- Jabs DA, Wingard J, Green WR, Farmer ER, Vogelsang G, Saral R. The eye in bone marrow transplantation. III. Conjunctival graft-vs-host disease. Arch Ophthalmol 1989;107:1343-8. [PubMed]
- Kim SK. Update on ocular graft versus host disease. Curr Opin Ophthalmol 2006;17:344-8. [PubMed]
- Huang D, Swanson EA, Lin CP, Schuman JS, Stinson WG, Chang W, Hee MR, Flotte T, Gregory K, Puliafito CA, Fujimoto JG. Optical coherence tomography. Science 1991;254:1178-81. [PubMed]
- Tomlins PH, Wang RK. Theory, developments and applications of optical coherence tomography. J Phys D: Appl Phys 2005;38:2519.
- Fercher AF, Drexler W, Hitzenberger CK, Lasser T. Optical coherence tomography - principles and applications. Rep Prog Phys 2003;66:239.
- Li P, Johnstone M, Wang RK. Full anterior segment biometry with extended imaging range spectral domain optical coherence tomography at 1340 nm. J Biomed Opt 2014;19:046013. [PubMed]
- Li P, An L, Reif R, Shen TT, Johnstone M, Wang RK. In vivo microstructural and microvascular imaging of the human corneo-scleral limbus using optical coherence tomography. Biomed Opt Express 2011;2:3109-18. [PubMed]
- Wang RK, Jacques SL, Ma Z, Hurst S, Hanson SR, Gruber A. Three dimensional optical angiography. Opt Express 2007;15:4083-97. [PubMed]
- Wang RK, An L, Francis P, Wilson DJ. Depth-resolved imaging of capillary networks in retina and choroid using ultrahigh sensitive optical microangiography. Opt Lett 2010;35:1467-9. [PubMed]
- An L, Qin J, Wang RK. Ultrahigh sensitive optical microangiography for in vivo imaging of microcirculations within human skin tissue beds. Opt Express 2010;18:8220-8. [PubMed]
- Reif R, Wang RK. Label-free imaging of blood vessel morphology with capillary resolution using optical microangiography. Quant Imaging Med Surg 2012;2:207-12. [PubMed]
- Yin X, Chao JR, Wang RK. User-guided segmentation for volumetric retinal optical coherence tomography images. J Biomed Opt 2014;19:086020. [PubMed]
- Yousefi S, Qin J, Zhi Z, Wang RK. Label-free optical lymphangiography: development of an automatic segmentation method applied to optical coherence tomography to visualize lymphatic vessels using Hessian filters. J Biomed Opt 2013;18:86004. [PubMed]
- Yousefi S, Zhi Z, Wang RK. Label-free optical imaging of lymphatic vessels within tissue beds in vivo. IEEE J Sel Top Quantum Electron 2014;20:6800510.