Feasibility and reproducibility of a cardiovascular magnetic resonance free-breathing, multi-shot, navigated image acquisition technique for ventricular volume quantification during continuous exercise
Introduction
Exercise testing can be used to detect underlying cardiovascular abnormalities which are not apparent at rest. Whilst exercise-stress echocardiography and nuclear scintigraphy are widely available, their limitations include poor acoustic windows (1), motion artefacts (2) and radiation exposure (3). Cardiovascular magnetic resonance (CMR) imaging at rest is highly accurate and reproducible (4,5), however exercise stress testing with CMR presents significant challenges. The early evolution of exercise CMR (exCMR) focused on improving the MRI-compatibility of exercise treadmill equipment from being placed external to the MRI room (6), to being in close proximity to the MRI scanner (7-9), to a fully MRI compatible treadmill placed adjacent to the MRI system (8,10-12). These protocols are however limited by the time delay needed to transfer the patient from the treadmill onto the scanner. Any time delay between the cessation of exercise and MRI image acquisition is critical, since exercise-induced functional abnormalities may begin to disappear almost immediately after exercise cessation (13-15).
The development of a MRI-compatible cycle ergometer allows patients to exercise in the supine position whilst inside the bore of the magnet (16). Imaging during continuous exercise eliminates the time lapse between exercise and imaging and may allow a more accurate assessment of changes in cardiac physiology during exertion. Excessive motion during exercise however poses a challenge in image acquisition. As a result, investigators have resorted to acquire images following transient cessation of exercise (17), during breath-holds (6,17,18) or using ungated real-time cine imaging (19). Reconstruction of a short axis stack for volumetric analysis from ungated real-time imaging, however, involves complex post-processing analysis in addition to a requirement for bespoke in-house software (19).
The objectives of this study were: (I) to assess the feasibility and reproducibility of a navigated cine image acquisition method for the assessment of the ventricular volumes during continuous exercise; (II) to examine its clinical feasibility in patients with significant valvular heart disease.
Methods
Study design and population
This study was performed in 3 stages: (I) a pilot phase in which the feasibility of a navigated image acquisition sequence was tested in healthy volunteers; (II) an assessment of inter-scan reproducibility in which each healthy volunteer underwent a repeat exCMR after a median of 16 weeks; (III) clinical application of this technique in patients with severe mitral regurgitation (MRegur). The study was approved by a local ethics committee (Yorkshire & The Humber-Leeds West 12/YH/0551) and complied with the Declaration of Helsinki. All participants provided written informed consent.
Pilot phase & reproducibility
Ten healthy volunteers with no history or symptoms of cardiovascular disease and no contraindications to CMR were recruited. Absolute and relative contraindications to exercise testing were adhered to according to American Heart Association (AHA) guidelines (20). All participants had a height of <190 cm. All healthy volunteers underwent a supine cardiopulmonary exercise test (CPET) prior to undertaking exCMR on a supine cycle ergometer. CMR was performed on a 1.5 Tesla MRI system with 70 cm bore (Ingenia, Philips Healthcare, Best, Netherlands) equipped with a 28-channel coil and free-breathing images were acquired during continuous exercise. Exercise intensity was individualized to the heart rate (HR) corresponding to 55% and 75% of the maximal HR (HRmax) attained on their pre-CMR supine CPET. After a median time of 16 weeks, exCMR was repeated using an identical scanner and protocol.
Clinical feasibility
The potential for translation of this technique into clinical practice was examined in a separate cohort of 6 patients with significant MRegur, all prospectively recruited from the valvular heart disease clinic at Leeds Teaching Hospitals NHS Trust. Inclusion criteria included: moderate-severe or severe MRegur on echocardiography, and New York Heart Association functional Class I. Exclusion criteria included: contraindications to exercise stress testing according to AHA guidelines (20), presence of atrial fibrillation, height >190 cm, inability to exercise and contraindications to CMR. In our institution treadmill CPET is used clinically in patients with significant MRegur and we utilized these data to prescribe the individualized HR during exCMR. To allow for the lower HR response in supine cycling compared to upright treadmill exercise and the reduced exercise tolerance seen in patients with severe MRegur, the prescribed HR had to be altered from healthy volunteers. Patients were thus exercised to 30–39% and 40–59% of their heart rate reserve (HRR), corresponding to ‘light’ and ‘moderate’-intensity exercise according to the American College of Sports Medicine guidelines (21). HRR was calculated based on this formula: resting HR on CPET + [x% of (max HR achieved on treadmill CPET − resting HR)]; where x is the target % of HRR.
Cardiopulmonary exercise testing
All healthy volunteers underwent CPET on a supine cycle ergometer (Lode BV, Groningen, The Netherlands). The crank length on the pre-CMR cycle ergometer was adjusted to replicate the setup of the in-scanner MRI ergometer. CPET was conducted as a ramp incremental test (15 W/min) to volitional intolerance. Breath-by-breath analysis of the volume and concentration of expired gases was achieved using an automated system (Medgraphics Ultima, Minnesota, USA). HR was continuously monitored via an attached 12-lead electrocardiogram (ECG). The main outcome measures were maximal HR and maximal power output in Watts. ExCMR was performed after a median of 8 days [interquartile range (IQR) 2–13].
Exercise CMR protocol and image acquisition
Exercise whilst in the bore of the magnet was conducted on a supine MRI-compatible cycle ergometer (Lode BV, Groningen, The Netherlands). Optimal participant preparation included instructions on consistent thoracic breathing, use of handrail to ensure trunk stability, skin preparation to maximize interface between electrode and skin, and securing vector ECG connections onto anterior chest wall with tape to ensure quality recording of ECG. A blood pressure (BP) cuff was placed on the left arm. Both the surface coil and torso pad were then firmly secured onto the participants with elastic Velcro® straps. The MRI table was advanced whilst participants performed a short bout of unloaded exercise to ensure that their knees did not contact the scanner casing during pedalling.
Free-breathing images were acquired at 3-stages, at rest and then during steady-state exercise at 55% HRmax and 75% HRmax. Exercise began with a 2 min warm-up at a power output of 0 W (unloaded). Work rate was incrementally increased by 10–20 W until the target 55% HRmax was achieved, and then adjusted to maintain the HR at the required target throughout the exercise. Verbal feedback was constantly given to participants and cycling cadence was maintained between 60–70 rpm. Following a rest period of 2 minutes, a second bout of exercise was undertaken until the target 75% HRmax was achieved. Heart rate and rhythm were continuously monitored, and BP was recorded at each stage. Each stage of exercise was maintained for 5–7 minutes (2 minutes to achieve steady-state in HR and approximately 3–5 minutes of image acquisition). Imaging was only performed during steady-state conditions, when HR was maintained at near constant levels. Criteria for termination prior to achieving target HR included participant’s request and a drop in systolic BP >10 mmHg.
The scan protocol included standard long axis views (vertical, horizontal long axis) and a short axis ventricular volume stack. Cine imaging was performed using a free-breathing, multi-shot, respiratory-navigated, balanced steady-state free precession pulse sequence. A respiratory echo-based navigator was placed on the right hemi-diaphragm with a 5 mm gating window and continuous gating level drift activated. A cylindrical MR radiofrequency excitation pulse from which a 1-dimensional projection of the lung-liver interface was generated and was used to infer the breathing phase. The navigator was played at the start of the R-R interval, at end-diastole of the cardiac cycle. The steady-state of ongoing balanced steady-state free precession (bSSFP) readout was stopped in the standard controlled manner by using half-alpha radiofrequency pulses to temporarily store the steady state magnetization in the z-direction. This allowed the respiratory navigator to last for a total duration of 24 ms (played out for 17 ms before resuming readout after 7 ms), equivalent to approximately 9 repetition time (TR). Retrospective cardiac triggering was used in this study (continuous data sampling). The bSSFP readout was continuous, wherein data from the ECG and k-space profile acquisition timings were matched to produce images for all cardiac phases. Cartesian sampling was used, and the acquired k-space lines were only accepted for image reconstruction if the right hemi-diaphragm position was within the gating window during end-expiratory phase. K-space profiles which were rejected outside of the respiratory navigator gate were reacquired. Other scan parameters were as follows: typical field of view (FOV) 320 mm × 320 mm, repetition time (TR) 2.8 ms, echo time (TE) 1.4 msec, flip angle 60°, temporal resolution 33 ms, SENSE factor 2, multi-shot turbo field echo (TFE) factor 11, TFE acquisition duration 30.4 ms, phase percentage 50%, slice thickness 10 mm, 0 mm gap, in-plane spatial resolution 2.4 mm × 2.4 mm and matrix 132×106. A total of 16 cardiac phases were acquired and this was reconstructed to 30 cardiac phases.
CMR analysis
CMR analysis was performed by two independent operators (PC, LB; both observers with 3 years CMR experience) using commercially available computer software (cmr42, Circle Cardiovascular Imaging Inc, Calgary, Alberta, Canada). Left and right ventricular volumes, and ejection fraction (EF) were calculated in the conventional method, by manually tracing endocardial contours in end-diastole and end-systole on the short axis stack (Figure S1). Biventricular end-diastolic and end-systolic volumes were calculated using a summation of discs technique (22). Stroke volume (SV) was measured as the difference between end-diastolic and end-systolic volume, whereas cardiac output was calculated as: SV × HR. All measured volumes and cardiac output parameters were indexed to body surface area (Mostellar formula). Longitudinal LV function in the form of mitral annular plane systolic excursion (MAPSE) was assessed by using mitral annular excursion. In the 4-chamber cine image, atrioventricular motion was measured at the lateral junction points between the left atrium and ventricle at end diastole and end systole. The perpendicular distance between these two points was measured. Figure 1 outlines the methodology used to assess LV longitudinal contraction.
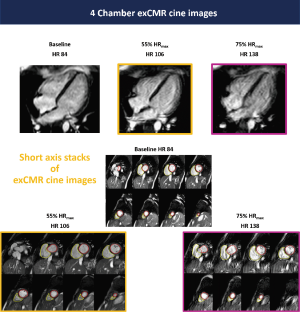
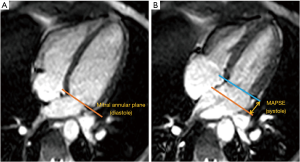
Statistical analysis
All statistical analysis was performed using the SPSS V.21.0 (IBM Corp., New York, USA). All continuous data were tested for normality using the Shapiro-Wilk test; variables are expressed as mean ± SD or median (IQR) in cases of skewed distributions. Categorical variables are expressed as frequencies and percentages. Repeated measures analysis of variance (ANOVA) with Bonferroni post-test analysis was used to compare data between rest and different stages of exercise. Intra- and inter-observer reproducibility was assessed by the coefficient of variation (CV) test, the standard deviation of differences between observations divided by the mean. P<0.05 was considered statistically significant.
Results
Healthy volunteers and baseline CMR data
All 10 healthy volunteers [7 men, age 25±2 years, body mass index (BMI) 23.1±2.2 kg/m2] completed the full study protocol. HR increased during exercise (68±12 vs. 94±13 vs. 131±11 bpm, baseline vs. 55% HRmaxvs. 75% HRmax; all P<0.001). Systolic BP was significantly higher during exercise at 75% HRmax than at baseline (130±12 vs. 120±10 mmHg; P=0.03), whilst diastolic BP remained unchanged (70±14 vs. 70±8 mmHg; P=1.00). Mean supine work rate for exercise at 55% HRmax and 75% HRmax was 25±19 W and 87±23 W, respectively. CMR data for all subjects are shown in Table 1. Figure 2 demonstrated the exCMR images at baseline and during exercise.
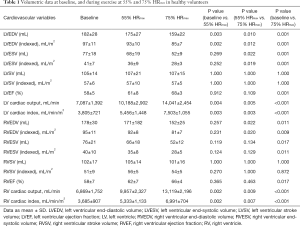
Full table
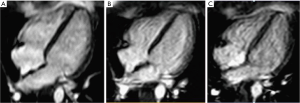
Left ventricular (LV) and right ventricular (RV) parameters during exercise
The changes in ventricular volumes during exercise are plotted in Figure 3. End-diastolic volume (EDV) of the LV decreased significantly during exercise at 55% and 75% HRmax. In contrast, RVEDV remained unchanged from baseline at 55% HRmax and significantly decreased at 75% HRmax (P=0.02). LV end-systolic volume (LVESV) decreased when exercised from 55% HRmax to 75% HRmax (P=0.02). During exercise at 55% HRmax, LVESV was however not significantly different from baseline. RV end-systolic volume (RVESV) significantly decreased during exercise at 75% HRmax compared to baseline. Both LV and RV stroke volumes remained unchanged. Ejection fractions (EF) for both ventricles were significantly higher during exercise at 75% HRmax when compared to their respective baseline values (LVEF 68%±3% vs. 58%±5%; P=0.001 and RVEF 66%±4% vs. 58%±7%; P=0.02). During exercise, LV and RV cardiac indexes also increased significantly (Figure 4).
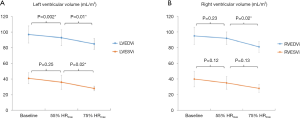
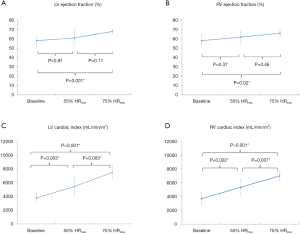
LV longitudinal contraction
Baseline MAPSE appears to be higher in healthy volunteers when compared to patients with severe mitral regurgitation (14±4 vs. 12±3 mm) (Figure 5). In the healthy volunteers, MAPSE increased from 14±4 to 19±5 mm (P=0.05) during exercise at 55% HRmax. At 75% HRmax, MAPSE appears to decrease to 17±4mm although this change was not statistically significant (P=1.00). In patients with severe mitral regurgitation, there was no significant change of MAPSE between baseline and both stages of exercise. There is a trend however, indicating that MAPSE increased with exercise and appear to decline slightly when higher intensity exercise was achieved.
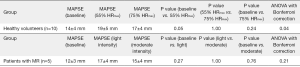
Intra- and inter-observer reproducibility
Intra-observer reproducibility of LV volumes, LV ejection fraction and LV cardiac index was excellent at all three stages, evidenced by CV ≤10% (Table 2). During exercise, the measurements of RVESV were more variable (CV 11–20%). The reproducibility of RV EDV, RV ejection fraction, and RV cardiac index was however excellent (CV <10%).
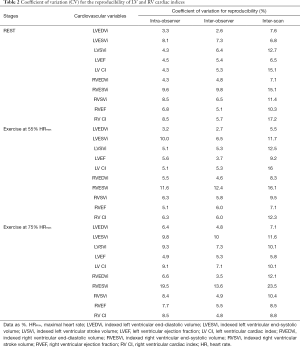
Full table
Inter-observer reproducibility of LV volumes, LV ejection fraction and LV cardiac index was also excellent at all three stages (CV for LVEDV ≤5%; LVESV ≤10%; LVEF <6%; LV cardiac index <8%). With incremental exercise, inter-observer reproducibility was better in the assessment of RVEDV (CV <5%), when compared to RVESV measurements (CV 12–14%). Although measurements of RVESV were more variable during exercise, the reproducibility of RV ejection fraction, RV stroke volume and RV cardiac index was however excellent. During exercise at 75% HRmax, inter-observer LVESV was more reproducible than RVESV (CV 10% vs. 14%).
Inter-scan reproducibility
We observed good inter-scan reproducibility for LV end-diastolic and end-systolic volumes during exercise; although only modest reproducibility was seen in the readings of LV cardiac index (CV 10–16%). The RVESV measurements were the least reproducible (CV 11–24%). Inter-scan LV and RV ejection fraction were however highly reproducible (CV <10%) at all 3 stages.
Ventricular volumes in clinical patients
Of 6 patients with severe MRegur, 5 patients (60% men, age 60±14 years, BMI 24±2.2 kg/m2) completed the full study protocol. exCMR had to be abandoned in 1 patient due to a significant hypotensive response. HR increased throughout exercise (73±6 vs. 111±11 vs. 118±18 bpm, baseline vs. light vs. moderate; all P<0.01). Systolic BP was significantly higher during moderate intensity exercise than at baseline (114±6 vs. 148±15 mmHg; P=0.02), whilst diastolic BP remained constant (74±9 vs. 80±8 vs. 66±14 mmHg; P=1.00). Mean supine work rate for light and moderate-intensity exercise was 44±19 and 53±32 W, respectively. CMR data for all clinical patients are described in Table 3. There was no significant change in the LVEDV during exercise in this small patient sample, and despite a downward trend of LVESV, this was not significant. LVEF was significantly higher when moderate-intensity exercise was achieved. The augmentation of cardiac output and cardiac index was apparent with incremental exercise. When considering the RV parameters, there was no significant change in its EDV. During moderate-intensity exercise, RVESV was significantly smaller than at baseline. Despite a numerical increase in RVEF with exercise, this was not significant. RV cardiac output and RV cardiac index were unchanged during light-intensity exercise but were significantly increased during moderate-intensity exercise.
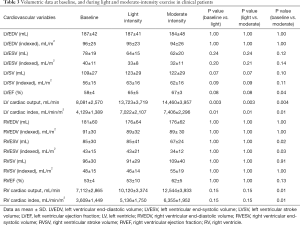
Full table
Discussion
This study demonstrated the (I) feasibility of the free-breathing, multi-shot, navigated image acquisition method in the serial assessment of ventricular volumes during continuous exercise; (II) excellent intra- and inter-observer reproducibility, in particular the LV indices; (III) clinical feasibility of this imaging method in a challenging group of patients with significant mitral regurgitation, the first exCMR study performed in this patient group.
Previously, image acquisition techniques using the MRI cycle ergometer have either involved a brief period of exercise cessation (17) or required a breath-hold protocol (6,18) in order to reduce excessive motion artefacts and avoid poor ECG signal. Ungated real-time CMR imaging (19,23,24) has been a method that enabled cine images to be acquired during continuous exercise. However, the post-processing analysis of these images requires retrospective synchronization of ECG and respiratory movements, in addition to the need for non-commercially available in-house software (19), therefore decreasing widespread attainability. The application of other image acquisition techniques such as motion correction (25,26) can be challenging in this setting due to the large amount of through plane motion during exercise. Navigator-echo-based gating techniques have been practical methods for effective reduction of respiration motion effects, and are well established for coronary MRI imaging (27,28). Our feasibility study demonstrated that the application of respiratory-navigated technique in exCMR has the potential to overcome respiratory motion which can be quite significant during vigorous exercise. This technique was feasible in both healthy volunteers and clinical patients, and the images acquired were analyzable and reproducible. Moreover, this imaging technique allowed serial assessment of cardiac function at incremental exercise with a further advantage that image analysis can be performed on widely used, commercially available software. This protocol therefore has the potential to increase the utility of exCMR as a clinical assessment tool.
La Gerche et al. (19) compared real-time ungated with gated CMR techniques and demonstrated that despite its complex post-processing analysis, ventricular volumes were analysable more frequently with real-time ungated compared with gated CMR (100% vs. 47%; P<0.001). In our gated CMR study, when combined with ‘respiratory-navigation’, sufficient image quality for analysis was achieved in 100% of the scans. La Gerche et al. also observed better interobserver variability for real-time ungated (CV =1.9% and 2.0% for LV and RV stroke volumes, respectively) than gated scans (CV =15.2% and 13.6%; P<0.01) (19). Comparing their gated study to ours, the incorporation of ‘respiratory-navigation’ in our gated study improved the CV for left and RV stroke volumes (CV of 7.3% and 4.9%, during exercise at 75% HRmax, respectively).
In 2017, Le et al. (24) combined real-time imaging with ECG-gated sequences to assess exercise cardiac volumetrics in healthy volunteers and athletes. Image acquisition, however, required suspension of exercise at the end of every stage for free-breathing imaging. The decline in HR following cessation of exercise (15,29) can potentially impair diagnostic accuracy and clinical utility. In contrast, our exCMR protocol permits imaging during continuous exercise, eliminating the time lapse between exercise and imaging altogether. In relation to scan parameters, our study had better temporal resolution (33 vs. 39 ms) and a smaller voxel size (2.4 mm × 2.4 mm vs. 3.3 mm × 2.3 mm) indicating improved spatial resolution.
The effects of left and RV volumes during physical exercise remains controversial. Some data are generally consistent with an enhanced contractile state during supine exercise, but the role of Frank Starling mechanism remains uncertain. In supine exCMR, there are several factors that affect the hemodynamic response. Firstly, exercise in the supine position results in a lower HR response but a greater rise in systolic BP, compared with upright exercise (30,31). This results in a similar double product (HR × systolic BP), which is an index of myocardial oxygenation (32), and a rational for similar detection rates of ischemia between upright and supine stress echocardiography despite a significant lower peak heart rates with supine exercise (30,33). As such, for a given exercise intensity, lower heart rates are expected in supine exercise, compared with upright exercise. Secondly, due to increased afterload in the supine position, it is postulated that end-systolic volumes are near maximal even at rest. As a result, a recent meta-analysis in exCMR studies by Beaudry et al. demonstrated no significant rise in LVEDV, with many studies demonstrating a non-significant decrease with exercise (34). This meta-analysis also demonstrated an exercise rise in LV stroke volume driven by a fall in end-systolic volume. The authors however did not account for one important factor, which is the effect of respiration on cardiac hemodynamics. Claessen et al. (35) elegantly demonstrated the significant effect respiration cycles have on cardiac hemodynamics; with end-expiration resulting in a significant rise in LVEDV and LV stroke volume and a fall in RVEDV and RV stroke volume, when compared with end-inspiration at numerous levels of exercise. The results of this meta-analysis should therefore be interpreted with caution, as although insightful, it analyses a heterogenous group of exCMR studies including both free-breathing and breath-held acquisitions, in a varied mix of healthy volunteers, endurance athletes and patients with cardiac disease, and who were exercised to different exercise intensities.
The results of this present study are in line with previous studies of supine exercise, showing a decrease in LV (24,36) and RV (18,23,37) EDVs, particularly during later stages of exercise. Similar to previous exCMR studies, we demonstrated no significant rise in stroke volume with exercise (38). Healthy volunteers have been shown to achieve their peak diastolic filling and contractility earlier (24). As a result, LVEDV in healthy volunteers peaked earlier and decreased subsequently. The increase in HR during exercise also reduced diastolic filling time, therefore leading to smaller LV and RV cavity during diastole. It is worth noting that as this study assessed 2 stages of exercise (55% and 75% HRmax) at moderate and high exercise intensities respectively, it is possible our data did not capture the initial LV dilatation described in the Frank Starling mechanism. Indeed, numerous prior investigators (23,24,35) have demonstrated an initial rise in LVEDV and LV stroke volume at early lower exercise stages followed by a fall at later higher intensity stages. However, due to the nature of exCMR studies being performed in small numbers, these changes often do not reach statistical significance. As such it is likely our study did not capture this early rise in LVEDV and LV stroke volume given the exercise stages were performed at moderate and high intensities. This is the first study to confirm clinical feasibility of this exCMR protocol in patients with severe MRegur. Ventricular volumes in patients were unchanged during light and moderate intensity exercise, which is likely a reflection of their relatively deconditioned state and poorer response in terms of myocardial contractility.
CMR MAPSE has been proposed as a simple and easy measure of longitudinal function in healthy volunteers and patients with hypertrophic cardiomyopathy (39). Longitudinal contraction assessed in the form of mitral annular plane systolic excursion (MAPSE) demonstrated that despite similar LV ejection fraction of >55%, healthy volunteers appeared to have a higher baseline MAPSE than those patients with severe mitral regurgitation; reflecting a better longitudinal contractility of the left ventricle. During exercise at 55% HRmax, healthy volunteers had an improved longitudinal contractility before the value plateaued at 75% HRmax. This initial change of improved contractility was not seen in patients with severe mitral regurgitation, potentially reflecting a deconditioned myocardium. These results should however be interpreted with caution in light of the relatively small sample population.
Intra-observer reproducibility of LV parameters was excellent at all three stages. Similarly, inter-observer reproducibility of LV parameters was also excellent. Although RVESV measurements were the least reproducible during exercise, the RV ejection fraction and cardiac index were however highly reproducible at all 3 stages. The inter-scan reproducibility was less optimal for LV parameters (CV 5–16%) and RVESV (CV 11–24%). The wide interscan variability can possibly be explained by the long 16 weeks scan interval between the 1st and 2nd exCMR scans. Although healthy volunteers had no specific exercise training during that period, other factors such as different loading conditions, diet and temperatures could influence cardiac physiology on a day-to-day basis.
This study has highlighted the potential of using ‘navigated’ image acquisition techniques for the assessment of cardiovascular response during continuous exercise. ExCMR has the potential of providing quantitative cardiac indices, whilst offering a direct link between physical activity, symptoms and stress imaging findings. Additionally, it can offer important information such as functional capacity and BP response. The use of exCMR can create new avenues for research and clinical practice, such as stress evaluation of ventricular dysfunction. This is particularly relevant to pathologies of the LV and RV, and pulmonary circulation that are challenging to assess by other imaging modalities. Further assessment of this ex-CMR protocol is now warranted for assessment of cardiac pathologies where current exercise imaging modalities have been shown to have limitations.
Limitations
As per all supine exCMR studies, there are general limitations with this approach. Cycling whilst lying in a flat, supine position is an unorthodox form of exercise, and skeletal muscle fatigue may lead to premature test termination (20). Knee-to-bore clearance whilst cycling is also limited by patient height and magnet bore diameter. This study had a maximum participant’s height of 188 cm. Furthermore, vigorous respiratory movement can also result in blurring or ghosting of images collated across cardiac cycles. When respiration is performed in the anterior-posterior direction, thus not captured by the navigator in the head-feet direction, the navigator could potentially fail to work. The use of respiratory navigator also causes interruption to steady-state imaging and these signal variations can potentially lead to artifacts, particularly in the systole phase during exercise. Optimal patient preparation, as detailed in the methodology, is therefore vital. Other limitations of exCMR include its inability to be performed in patients with certain implanted devices. Since most CMR acquisitions are acquired over multiple cardiac cycles, arrhythmias such as atrial fibrillation or premature ventricular contractions may pose additional challenges for standard CMR sequences. The study population was small, and the reproducibility should therefore be interpreted with caution. Although highly reproducible, the findings of this study were also not validated against an invasive reference standard. Further work could look into assessing the accuracy of this imaging method against invasive exercise standards (direct Fick method) in deriving cardiac output. This technique was not intended to achieve the 85% of ‘age-predicted maximal heart rate’ required for myocardial ischaemia testing purposes, as in-scanner 12 lead ECG monitoring is not feasible, and therefore accurate assessment of ST segment changes during exercise, which may prompt test termination, cannot be performed. The primary aim of this navigated exCMR technique was to assess the serial change in ventricular volumes with exercise as this can serve as an important tool in enabling understanding of physiology in patients with exertional symptoms and structural/congenital heart disease.
Conclusions
This exercise CMR protocol using a novel application of the free-breathing, multi-shot, navigated imaging method allows simultaneous assessment of the left and RV volumes during continuous exercise. This study demonstrates feasibility of exCMR in patients with mitral regurgitation for the first time. Intra and inter-observer readings were highly reproducible. Clinical feasibility of this protocol suggests a future role in the assessment of patients with exercise-related symptoms.
Acknowledgments
The authors are grateful for the support and assistance of the radiographers (Margaret Saysell, Gavin Bainbridge, Stephen Mhiribidi, Georgina Casey and Lisa Lewis) and research nurse (Fiona Richards) during this project. Only the abstract of this manuscript (<400 words) has been previously published in Heart 2018;104:A46-7.
Funding: The research was part-supported by the National Institute for Health Research (NIHR) infrastructure at Leeds which has no influence over the contents of this manuscript.
Footnote
Conflicts of Interest: All authors have completed the ICMJE uniform disclosure form (available at http://dx.doi.org/10.21037/qims-20-117). DMH reports other from Philips, outside the submitted work. GJF reports other from Novartis, during the conduct of the study. The other authors have no conflicts of interest to declare.
Ethical Statement: The study was approved by a local ethics committee (Yorkshire & The Humber-Leeds West 12/YH/0551) and complied with the Declaration of Helsinki (as revised in 2013). All participants provided written informed consent.
Open Access Statement: This is an Open Access article distributed in accordance with the Creative Commons Attribution-NonCommercial-NoDerivs 4.0 International License (CC BY-NC-ND 4.0), which permits the non-commercial replication and distribution of the article with the strict proviso that no changes or edits are made and the original work is properly cited (including links to both the formal publication through the relevant DOI and the license). See: https://creativecommons.org/licenses/by-nc-nd/4.0/.
References
- A Randomized Cross-Over Study for Evaluation of the Effect of Image Optimization With Contrast on the Diagnostic Accuracy of Dobutamine Echocardiography in Coronary Artery Disease. The OPTIMIZE Trial. JACC Cardiovasc Imaging 2008;1:145-52. [Crossref] [PubMed]
- Accuracy and limitations of exercise echocardiography in a routine clinical setting. J Am Coll Cardiol 1992;19:74-81. [Crossref] [PubMed]
- Issues regarding radiation dosage of cardiac nuclear and radiography procedures. J Nucl Cardiol 2006;13:19-23. [Crossref] [PubMed]
- Comparison of interstudy reproducibility of cardiovascular magnetic resonance with two-dimensional echocardiography in normal subjects and in patients with heart failure or left ventricular hypertrophy. Am J Cardiol 2002;90:29-34. [Crossref] [PubMed]
- Cardiovascular magnetic resonance imaging: what the general cardiologist should know. Heart 2016;102:1589-603. [Crossref] [PubMed]
- Feasibility to detect severe coronary artery stenoses with upright treadmill exercise magnetic resonance imaging. Am J Cardiol 2003;92:603-6. [Crossref] [PubMed]
- Cardiac function and myocardial perfusion immediately following maximal treadmill exercise inside the MRI room. J Cardiovasc Magn Reson 2008;10:3. [Crossref] [PubMed]
- Real-time cine and myocardial perfusion with treadmill exercise stress cardiovascular magnetic resonance in patients referred for stress SPECT. J Cardiovasc Magn Reson 2010;12:41. [Crossref] [PubMed]
- Treadmill Stress Cardiac Magnetic Resonance Imaging. First In Vivo Demonstration of Exercise-Induced Apical Ballooning. J Am Coll Cardiol 2008;52:1884. [Crossref] [PubMed]
- An MR-Compatible Treadmill for Exercise Stress Cardiac Magnetic Resonance Imaging. Magn Reson Med 2012;67:880-9. [Crossref] [PubMed]
- Comparison of treadmill exercise stress cardiac MRI to stress echocardiography in healthy volunteers for adequacy of left ventricular endocardial wall visualization: A pilot study. J Magn Reson Imaging 2014;39:1146-52. [Crossref] [PubMed]
- Diagnostic Performance of Treadmill Exercise Cardiac Magnetic Resonance: The Prospective, Multicenter Exercise CMR’s Accuracy for Cardiovascular Stress Testing (EXACT) Trial. J Am Heart Assoc 2016;5:e003811. [Crossref] [PubMed]
- Peak exercise and immediate postexercise imaging for the detection of left ventricular functional abnormalities in coronary artery disease. Am J Cardiol 1984;53:1532-7. [Crossref] [PubMed]
- Comparison of postexercise and transesophageal atrial pacing two-dimensional echocardiography for detection of coronary artery disease. Am J Cardiol 1986;57:547-53. [Crossref] [PubMed]
- Heart rate recovery following maximal arm and leg-ergometry. Clin Auton Res 2011;21:117-20. [Crossref] [PubMed]
- Design and testing of an MRI-compatible cycle ergometer for non-invasive cardiac assessments during exercise. Biomed Eng Online 2012;11:13. [Crossref] [PubMed]
- Biventricular response to supine physical exercise in young adults assessed with ultrafast magnetic resonance imaging. Am J Cardiol 2001;87:601-5. [Crossref] [PubMed]
- Moderate intensity supine exercise causes decreased cardiac volumes and increased outer volume variations: A cardiovascular magnetic resonance study. J Cardiovasc Magn Reson 2013;15:96. [Crossref] [PubMed]
- Cardiac MRI: A new gold standard for ventricular volume quantification during high-intensity exercise. Circ Cardiovasc Imaging 2013;6:329-38. [Crossref] [PubMed]
- Exercise standards for testing and training: A scientific statement from the American heart association. Circulation 2013;128:873-934. [Crossref] [PubMed]
- ACSM. ACSM’s Guidelines for Exercise Testing and Prescription (10th edition). Wolters Kluwer, 2017:472.
- Relationship between central sympathetic drive and magnetic resonance imaging-determined left ventricular mass in essential hypertension. Circulation 2007;115:1999-2005. [Crossref] [PubMed]
- Feasibility and Reproducibility of Biventricular Volumetric Assessment of Cardiac Function During Exercise Using Real-Time Radial k-t SENSE Magnetic Resonance Imaging. J Magn Reson Imaging 2009;29:1062-70. [Crossref] [PubMed]
- Assessing exercise cardiac reserve using real-time cardiovascular magnetic resonance. J Cardiovasc Magn Reson 2017;19:7. [Crossref] [PubMed]
- Motion corrected compressed sensing for free-breathing dynamic cardiac MRI. Magn Reson Med 2013;70:504-16. [Crossref] [PubMed]
- Matrix description of general motion correction applied to multishot images. Magn Reson Med 2005;54:1273-80. [Crossref] [PubMed]
- Navigator-echo-based real-time respiratory gating and triggering for reduction of respiration effects in three-dimensional coronary MR angiography. Radiology 1996;198:55-60. [Crossref] [PubMed]
- Prospective navigator correction of image position for coronary MR angiography. Radiology 1997;203:733-6. [Crossref] [PubMed]
- Exercise Standards for Testing and Training: A Statement for Healthcare Professionals From the American Heart Association. Circulation 2001;104:1694-740. [Crossref] [PubMed]
- Supine bicycle versus post-treadmill exercise echocardiography in the detection of myocardial ischemia: A randomized single-blind crossover trial. J Am Coll Cardiol 1999;33:1485-90. [Crossref] [PubMed]
- Physiologic responses of cardiac patients to supine, recumbent, and upright cycle ergometry. Arch Phys Med Rehabil 1995;76:257-61. [Crossref] [PubMed]
- Hemodynamic predictors of myocardial oxygen consumption during static and dynamic exercise. Circulation 1974;50:1179-89. [Crossref] [PubMed]
- Comparison of supine bicycle exercise and treadmill exercise Doppler echocardiography in evaluation of patients with coronary artery disease. Am J Cardiol 2003;91:1245-8. [Crossref] [PubMed]
- Exercise cardiac magnetic resonance imaging: A feasibility study and meta-analysis. Am J Physiol Regul Integr Comp Physiol 2018;315:R638-45. [Crossref] [PubMed]
- Interaction between respiration and right versus left ventricular volumes at rest and during exercise: A real-time cardiac magnetic resonance study. Am J Physiol Heart Circ Physiol 2014;306:H816-24. [Crossref] [PubMed]
- Left ventricular size and performance during graded supine exercise in normal subjects. Jpn Heart J 1983;24:503-14. [Crossref] [PubMed]
- Volumetric response of right ventricle during progressive supine exercise in men. Am J Physiol 1991;261:H751-4. [PubMed]
- Assessing vascular response to exercise using a combination of real-time spiral phase contrast MR and noninvasive blood pressure measurements. J Magn Reson Imaging 2010;31:997-1003. [Crossref] [PubMed]
- Myocardial strain measurement with feature-tracking cardiovascular magnetic resonance: Normal values. Eur Heart J Cardiovasc Imaging 2015;16:871-81. [Crossref] [PubMed]