Design and numerical evaluation of a volume coil array for parallel MR imaging at ultrahigh fields
Introduction
Radiofrequency (RF) coil arrays with a set of surface coils (1-7) become more popular in MR imaging as they can provide improved signal-to-noise ratio and the capability of performing parallel imaging (8-30). Compared with surface coils, RF volume coils, such as birdcage coils (31), are able to generate homogenous magnetic fields (B1) and potentially uniform MR images (17,32-36,37-53). In recent years it has been demonstrated that multiple tuned volume coils for multiple nuclear MR applications (54) can be designed by integrating different type of birdcage coils which are intrinsically decoupled due to the cancellation of their magnetic flux. In this work, we propose and investigate a single tuned proton volume coil array based on that design concept using combination of different typed birdcage coils for improving SNR (31) and also for implementing parallel imaging (55-59) and parallel transmission (60-65) to accelerate imaging. To demonstrate the design concept, a 3-channel volume coil array was designed, which consists of a conventional birdcage coil, a transverse birdcage coil, and a helix birdcage coil. All the three coils were intrinsically decoupled and tuned to 298 MHz which is corresponding to proton frequency at 7T. By taking advantage of the intrinsic decoupling feature, this volume coil array can be designed without using any decoupling circuitry between array elements. The performance of the volume coil array is evaluated using FDTD numerical simulation (39,66-69) in terms of the RF field distribution and mutual coupling. The parallel imaging performance of the 3-element volume coil array was also evaluated by calculating the g-factor map (56) at different acceleration rates for SENSE reconstruction.
Methods
The structure of the proposed multichannel volume coil array is shown in Figure 1. The inner coil is a 16-strut low-pass helix birdcage coil with 3 cm ID, the middle coil is a 16-strut birdcage coil with 3.8 cm ID, while the outer coil is a conventional birdcage coil with 4.5 cm ID. The coils were all built using copper tape and the coil length was 4 cm. Capacitors were place on each strut and used to tune the resonant frequency to 298 MHz. The coaxial feed port was modeled as a voltage source with 50 Ohm impedance. The software XFDTD6.4 (Remcom Inc., State College, PA, USA) was used to model this 3-channel birdcage coil array and evaluate the magnetic field distribution. The Yee cell size was 0.5 mm on both the transverse plane and longitudinal direction which was small enough for satisfying the accuracy requirement of the FDTD calculation. The boundary condition was set as Perfectly Matching Layers (PML). To ensure the calculation stability and accurate performance of the PML absorbing boundaries, in each direction 20 Yee cells of free space padding were placed between the PML boundary and the conducting materials. The Gauss waveform was used to sweep frequency to determine the proper capacitance value for each volume coil working at 298.2 MHz, and the stop criteria were that the calculation converged to –30 dB. Then, after obtaining the capacitance, the sinusoid waveform was used to calculate the RF field distributions and the stop criteria were set as the calculation converged to –30 dB.
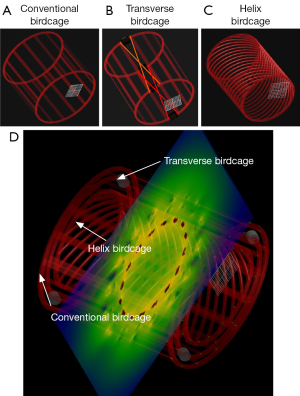
To demonstrate the parallel imaging performance of the proposed 3-element volume coil array, the g-factor maps at different acceleration rates were calculated and plotted based on the RF field distribution calculated from the FDTD simulation by using the equation below (56):
[1] |
where ρ is the index of voxel in the FOV, S is the reduced Fourier encoding, ψ is the noise correlation matrix between channels. The g-factor is strongly depends on the voxel position and is related to the signal to noise ratio (SNR):
[2] |
where R is the acceleration rate, showing that the SNR of the accelerated image is inversely proportional to the g-factor.
Results
The simulated B1 field distribution is shown in Figure 2. The 1st row is the results of the conventional birdcage; the 2nd row is the results of the transverse birdcage coil, while the 3rd row is the helix birdcage coil. The left column results are the B1 field distribution of each coil when combined together as a birdcage coil array, while the right column results are the B1 distribution of each individual coil. It is clearly shown that, the B1 field distribution of each birdcage coil within the volume coil is almost the same as that of the individual coil, demonstrating excellent isolation between the three channels. The Blue arrows in the figures denote the direction of the magnetic field flux density. In a conventional birdcage coil, the B1 direction are along one direction within the whole coil; in a transverse birdcage coil, the B1 direction of the two half is opposed to each other; while in the helix birdcage coil, the B1 direction in one slice is the same, but along longitudinal direction the B1 direction varies from 0° to 360°, therefore its net magnetic flux is zero. Thus the flux from one coil to the others is theoretically zero, leading to excellent decoupling between each pair of birdcage coils.
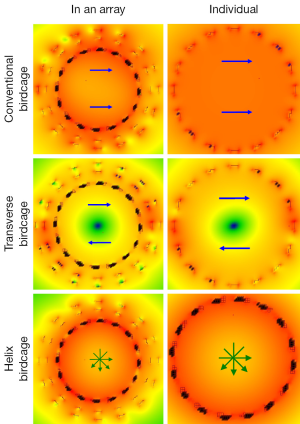
Figure 3 shows the sensitivity maps and phase maps on transverse plane of the three volume coil elements when fed individually. This transverse plane is the central slice. Each element shows different B1 distribution, leading to different sensitivity maps which can be used for parallel imaging. Figure 4 shows the g-factor maps for 1D SENSE reconstruction at different acceleration rates: R =1.2, 1.5, 1.7, 2.0, 2.3 and 2.5. The acceleration rate and the corresponding average g-factor are shown on the top of each g-map. It is demonstrated that with the increase of the acceleration the average g-factor increases (Figure 5). At acceleration rate of 2, the g-factor is around 1.5, which is practically good for performing parallel imaging for this 3-element coil array.
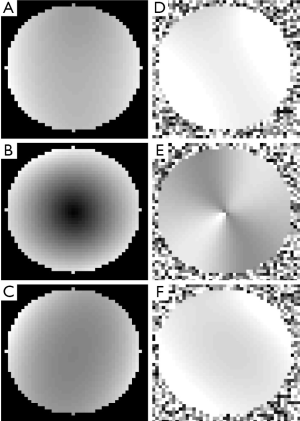
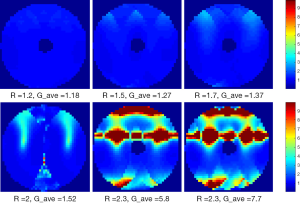
Discussion and conclusions
In this work, a novel volume coil array design using different types of birdcage coils is proposed for MR imaging. The resonant elements of this volume coil array are a conventional birdcage coil, a transverse birdcage coil and a helix birdcage coil. The field behavior and the parallel imaging performance of the volume coil array have been investigated using numerical simulations and SENSE parallel imaging algorithm. The studies verify the theoretical expectation on electromagnetic decoupling performance of these birdcage coil elements, demonstrating the feasibility of this volume coil array design technique. From the results it can be seen that the three birdcage coils are deeply decoupled from each other due to their unique magnetic flux directions resulting in the net magnetic flux cancellation in both the transverse birdcage coil and the helix birdcage coil. In this design method with intrinsic decoupling feature, there is no dedicated decoupling circuits needed, making the volume coil array design simple and practical. Each element of a volume coil array has bigger imaging coverage than that of conventional surface coil arrays, which may potentially lead to a better g-factor and ultimately improved parallel imaging performance, particularly in imaging with large field-of-view (FOV). Compared with conventional non-array volume coils, the volume coil array is expected to provide increased SNR in MR imaging. Typical magnetic field distribution of surface coil arrays is not uniform over the FOV, showing strong gradient behavior with much higher signal intensity in the peripheral area and weak signal in the center region. With the volume coil array, more uniform SNR distribution can be expected across a large volume.
Acknowledgements
This work was partially supported by NIH grants EB004453, EB008699, K99EB015487 and P41EB013598, a QB3 Research Award, a Springer Med Fund Award, and a National Natural Science Foundation of China Grant (51228702).
Disclosure: The authors declare no conflict of interest.
References
- Ackerman JJ, Grove TH, Wong GG, et al. Mapping of metabolites in whole animals by 31P NMR using surface coils. Nature 1980;283:167-70. [PubMed]
- Roemer PB, Edelstein WA, Hayes CE, et al. The NMR phased array. Magn Reson Med 1990;16:192-225. [PubMed]
- Hayes CE, Tsuruda JS, Mathis CM. Temporal lobes: surface MR coil phased-array imaging. Radiology 1993;189:918-20. [PubMed]
- Porter JR, Wright SM, Reykowski A. A 16-element phased-array head coil. Magn Reson Med 1998;40:272-9. [PubMed]
- Zhang X, Ugurbil K, Chen W. Microstrip RF surface coil design for extremely high-field MRI and spectroscopy. Magn Reson Med 2001;46:443-50. [PubMed]
- Weiger M, Pruessmann KP, Leussler C, et al. Specific coil design for SENSE: a six-element cardiac array. Magn Reson Med 2001;45:495-504. [PubMed]
- Wiggins GC, Triantafyllou C, Potthast A, et al. 32-channel 3 Tesla receive-only phased-array head coil with soccer-ball element geometry. Magn Reson Med 2006;56:216-23. [PubMed]
- Balu N, Yarnykh VL, Scholnick J, et al. Improvements in carotid plaque imaging using a new eight-element phased array coil at 3T. J Magn Reson Imaging 2009;30:1209-14. [PubMed]
- Wright SM, McDougall MP, Brown DG. Single Echo Acquisition (SEA) MR Imaging. Proceedings of International Society of Magnetic Resonance in Medicine, Toronto, Canada, 2003:23.
- Buehrer M, Pruessmann KP, Boesiger P, et al. Array compression for MRI with large coil arrays. Magn Reson Med 2007;57:1131-9. [PubMed]
- Chang G, Friedrich KM, Wang L, et al. MRI of the wrist at 7 tesla using an eight-channel array coil combined with parallel imaging: preliminary results. J Magn Reson Imaging 2010;31:740-6. [PubMed]
- Hardy CJ, Cline HE, Giaquinto RO, et al. 32-element receiver-coil array for cardiac imaging. Magn Reson Med 2006;55:1142-9. [PubMed]
- Hardy CJ, Giaquinto RO, Piel JE, et al. 128-channel body MRI with a flexible high-density receiver-coil array. J Magn Reson Imaging 2008;28:1219-25. [PubMed]
- Henry RG, Fischbein NJ, Dillon WP, et al. High-sensitivity coil array for head and neck imaging: technical note. AJNR Am J Neuroradiol 2001;22:1881-6. [PubMed]
- Lee RF, Hardy CJ, Sodickson DK, et al. Lumped-element planar strip array (LPSA) for parallel MRI. Magn Reson Med 2004;51:172-83. [PubMed]
- Adriany G, Van de Moortele PF, Wiesinger F, et al. Transmit and receive transmission line arrays for 7 Tesla parallel imaging. Magn Reson Med 2005;53:434-45. [PubMed]
- Wiggins GC, Potthast A, Triantafyllou C, et al. Eight-channel phased array coil and detunable TEM volume coil for 7 T brain imaging. Magn Reson Med 2005;54:235-40. [PubMed]
- Zhu Y, Hardy CJ, Sodickson DK, et al. Highly parallel volumetric imaging with a 32-element RF coil array. Magn Reson Med 2004;52:869-77. [PubMed]
- Li Y, Xie Z, Pang Y, et al. ICE decoupling technique for RF coil array designs. Med Phys 2011;38:4086-93. [PubMed]
- Boskamp EB, Lee RF. Whole body LPSA transceive array with optimized transmit homogeneity. Proceedings of International Society of Magnetic Resonance in Medicine, Honolulu, HI, 2002:903.
- Pang Y, Zhang X, Xie Z, et al. Common-mode differential-mode (CMDM) method for double-nuclear MR signal excitation and reception at ultrahigh fields. IEEE Trans Med Imaging 2011;30:1965-73. [PubMed]
- Wu B, Wang C, Krug R, et al. 7T human spine imaging arrays with adjustable inductive decoupling. IEEE Trans Biomed Eng 2010;57:397-403. [PubMed]
- Wu B, Wang C, Kelley DA, et al. Shielded microstrip array for 7T human MR imaging. IEEE Trans Med Imaging 2010;29:179-84. [PubMed]
- Wu B, Li Y, Wang C, et al. Multi-reception strategy with improved SNR for multichannel MR imaging. PLoS One 2012;7:e42237. [PubMed]
- Wu B, Wang C, Lu J, et al. Multi-channel microstrip transceiver arrays using harmonics for high field MR imaging in humans. IEEE Trans Med Imaging 2012;31:183-91. [PubMed]
- Wu B, Zhang X, Wang C, et al. Flexible transceiver array for ultrahigh field human MR imaging. Magn Reson Med 2012;68:1332-8. [PubMed]
- Li Y, Yu B, Pang Y, et al. Planar quadrature RF transceiver design using common-mode differential-mode (CMDM) transmission line method for 7T MR imaging. PLoS One 2013;8:e80428. [PubMed]
- Li Y, Pang Y, Vigneron D, et al. Investigation of multichannel phased array performance for fetal MR imaging on 1.5T clinical MR system. Quant Imaging Med Surg 2011;1:24-30. [PubMed]
- Pang Y, Zhang X. Precompensation for mutual coupling between array elements in parallel excitation. Quant Imaging Med Surg 2011;1:4-10. [PubMed]
- Gilbert KM, Belliveau JG, Curtis AT, et al. A conformal transceive array for 7 T neuroimaging. Magn Reson Med 2012;67:1487-96. [PubMed]
- Hayes C, Edelstein W, Schenck J, et al. An efficient, highly homogeneous radiofrequency coil for whole-body NMR imaging at 1.5T. J Magn Reson 1985;63:622-8.
- Alecci M, Collins CM, Smith MB, et al. Radio frequency magnetic field mapping of a 3 Tesla birdcage coil: experimental and theoretical dependence on sample properties. Magn Reson Med 2001;46:379-85. [PubMed]
- Avdievich NI, Hetherington HP. 4 T Actively detuneable double-tuned 1H/31P head volume coil and four-channel 31P phased array for human brain spectroscopy. J Magn Reson 2007;186:341-6. [PubMed]
- Vaughan JT, Adriany G, Garwood M, et al. Detunable transverse electromagnetic (TEM) volume coil for high-field NMR. Magn Reson Med 2002;47:990-1000. [PubMed]
- Vaughan JT, Hetherington HP, Otu JO, et al. High frequency volume coils for clinical NMR imaging and spectroscopy. Magn Reson Med 1994;32:206-18. [PubMed]
- Wen H, Chesnick AS, Balaban RS. The design and test of a new volume coil for high field imaging. Magn Reson Med 1994;32:492-8. [PubMed]
- Zhang X, Ugurbil K, Chen W. A microstrip transmission line volume coil for human head MR imaging at 4T. J Magn Reson 2003;161:242-51. [PubMed]
- Fujita H, Braum WO, Morich MA. Novel quadrature birdcage coil for a vertical B(0) field open MRI system. Magn Reson Med 2000;44:633-40. [PubMed]
- Ibrahim TS, Lee R, Baertlein BA, et al. B1 field homogeneity and SAR calculations for the birdcage coil. Phys Med Biol 2001;46:609-19. [PubMed]
- Jin J, Shen G, Perkins T. On the field inhomogeneity of a birdcage coil. Magn Reson Med 1994;32:418-22. [PubMed]
- Lin FH, Kwong KK, Huang IJ, et al. Degenerate mode birdcage volume coil for sensitivity-encoded imaging. Magn Reson Med 2003;50:1107-11. [PubMed]
- Murphy-Boesch J, Srinivasan R, Carvajal L, et al. Two configurations of the four-ring birdcage coil for 1H imaging and 1H-decoupled 31P spectroscopy of the human head. J Magn Reson B 1994;103:103-14. [PubMed]
- Shen GX, Boada FE, Thulborn KR. Dual-frequency, dual-quadrature, birdcage RF coil design with identical B1 pattern for sodium and proton imaging of the human brain at 1.5 T. Magn Reson Med 1997;38:717-25. [PubMed]
- Tropp J. The theory of the birdcage resonator. J Magn Reson 1989;82:51-62.
- Vullo T, Zipagan RT, Pascone R, et al. Experimental design and fabrication of birdcage resonators for magnetic resonance imaging. Magn Reson Med 1992;24:243-52. [PubMed]
- Wang C, Qu P, Shen GX. Potential advantage of higher-order modes of birdcage coil for parallel imaging. J Magn Reson 2006;182:160-7. [PubMed]
- Zhang X, Ugurbil K, Sainati R, et al. An inverted-microstrip resonator for human head proton MR imaging at 7 tesla. IEEE Trans Biomed Eng 2005;52:495-504. [PubMed]
- Pang Y, Xie Z, Li Y, et al. Resonant Mode Reduction in Radiofrequency Volume Coils for Ultrahigh Field Magnetic Resonance Imaging. Materials (Basel) 2011;4:1333-44. [PubMed]
- Wang C, Li Y, Wu B, et al. A practical multinuclear transceiver volume coil for in vivo MRI/MRS at 7 T. Magn Reson Imaging 2012;30:78-84. [PubMed]
- Pang Y, Xie Z, Xu D, et al. A dual-tuned quadrature volume coil with mixed λ/2 and λ/4 microstrip resonators for multinuclear MRSI at 7 T. Magn Reson Imaging 2012;30:290-8. [PubMed]
- Zhang X, Zhu XH, Chen W. Higher-order harmonic transmission-line RF coil design for MR applications. Magn Reson Med 2005;53:1234-9. [PubMed]
- Alsop DC, Connick TJ, Mizsei G. A spiral volume coil for improved RF field homogeneity at high static magnetic field strength. Magn Reson Med 1998;40:49-54. [PubMed]
- Li Y, Wang C, Yu B, et al. Image homogenization using pre-emphasis method for high field MRI. Quant Imaging Med Surg 2013;3:217-23. [PubMed]
- Wong WH, Unno S, Anderson WA. Multiple tuned birdcage coils. United States of America, 2002, Patent No. US6420871.
- Sodickson DK, Manning WJ. Simultaneous acquisition of spatial harmonics (SMASH): fast imaging with radiofrequency coil arrays. Magn Reson Med 1997;38:591-603. [PubMed]
- Pruessmann KP, Weiger M, Scheidegger MB, et al. SENSE: sensitivity encoding for fast MRI. Magn Reson Med 1999;42:952-62. [PubMed]
- Griswold MA, Jakob PM, Heidemann RM, et al. Generalized autocalibrating partially parallel acquisitions (GRAPPA). Magn Reson Med 2002;47:1202-10. [PubMed]
- Ji JX, Son JB, Rane SD. PULSAR: a matlab toolbox for parallel magnetic resonance imaging using array coils and multiple channel receivers. Concepts Magn Reson B: Magn Reson Eng 2007;31B:24-36.
- Lin FH, Kwong KK, Belliveau JW, et al. Parallel imaging reconstruction using automatic regularization. Magn Reson Med 2004;51:559-67. [PubMed]
- Katscher U, Börnert P, Leussler C, et al. Transmit SENSE. Magn Reson Med 2003;49:144-50. [PubMed]
- Zhu Y. Parallel excitation with an array of transmit coils. Magn Reson Med 2004;51:775-84. [PubMed]
- Grissom W, Yip CY, Zhang Z, et al. Spatial domain method for the design of RF pulses in multicoil parallel excitation. Magn Reson Med 2006;56:620-9. [PubMed]
- Ma C, Xu D, King KF, et al. Joint design of spoke trajectories and RF pulses for parallel excitation. Magn Reson Med 2011;65:973-85. [PubMed]
- Xu D, King KF, Liang ZP. Improving k-t SENSE by adaptive regularization. Magn Reson Med 2007;57:918-30. [PubMed]
- Zhang X, Pang Y. Parallel Excitation in Ultrahigh Field Human MR Imaging and Multi-Channel Transmit System. OMICS J Radiol 2012;1:e110. [PubMed]
- Collins CM, Yang QX, Wang JH, et al. Different excitation and reception distributions with a single-loop transmit-receive surface coil near a head-sized spherical phantom at 300 MHz. Magn Reson Med 2002;47:1026-8. [PubMed]
- Yang QX, Wang J, Zhang X, et al. Analysis of wave behavior in lossy dielectric samples at high field. Magn Reson Med 2002;47:982-9. [PubMed]
- Wang J, Yang QX, Zhang X, et al. Polarization of the RF field in a human head at high field: a study with a quadrature surface coil at 7.0 T. Magn Reson Med 2002;48:362-9. [PubMed]
- Pang Y, Wu B, Wang C, et al. Numerical Analysis of Human Sample Effect on RF Penetration and Liver MR Imaging at Ultrahigh Field. Concepts Magn Reson Part B Magn Reson Eng 2011;39B:206-16. [PubMed]