Imaging of solitary pulmonary nodule—a clinical review
Introduction
Increasingly widespread use of imaging, in particular computed tomography (CT), in clinical practice today has led to a steep rise in incidental findings, or ‘incidentalomas’. Indeterminate incidental findings often generate follow-up recommendations by radiologists, either in the form of interval scans, or additional imaging using different techniques or modalities.
Pulmonary nodules are one of the commonest incidental findings, found in 14.8% of asymptomatic subjects in a study on whole-body CT screening (1). A Mayo Clinic study in 2003 on lung cancer screening with low-dose CT found pulmonary nodules in 69% of patients, with high rate of benign nodule detection, raising concerns of overdiagnosis (2).
Pulmonary nodules are, as defined by Fleischner Society, “rounded opacities, well or poorly defined, measuring up to 3 cm in diameter” on chest radiographs and CT scans (3). In cases of solitary pulmonary nodule (SPN), the single, isolated, relatively spherical opacity less than 3 cm, completely surrounded by normal lung parenchyma, should not be associated with other abnormality, including atelectasis, hilar adenopathy or pleural effusion (4).
The significance of pulmonary nodules is variable, dependent first and foremost on the clinical context. In a patient with known primary malignancy, lung nodules, regardless of being solitary or multiple, would be deemed suspicious for metastases; whereas in a patient with no reported respiratory symptom or risk factor such as smoking history, a solitary nodule may be incidental and benign. The United States National Lung Cancer Screening Trial reported high false positive rates with low-dose CT screening, due to benign intrapulmonary nodes and non-calcified granulomas (5). Nevertheless, an SPN is almost always regarded as indeterminate on imaging, unless it demonstrates overt radiological feature favoring benignity (for example, popcorn calcification in benign hamartoma).
The reported incidence of lung cancer in patients with SPN varies widely, from 2-13% in screening studies, to 46-82% in positron emission tomography (PET) studies (6-8). Lung cancer is the most common cancer worldwide, with highest incidence rates in Europe (9). Although only a small proportion of patients with lung cancer presents with SPN on imaging, this is an important group of patients because SPN represents small tumor size and early disease, with high 5-year survival rates of 65-80% after surgical resection. Therefore, accurate diagnosis of SPNs is a challenge to both clinicians and radiologists.
In this review, we aim to discuss the diagnostic dilemma of SPNs, differential diagnoses, investigations and imaging of SPNs, functional imaging mainly PET-CT and its common pitfalls, and emerging new techniques for imaging evaluation of SPNs.
Differential diagnoses of SPNs and conventional radiological evaluation
There is a long list of differential diagnoses for SPNs, of which some are included in Table 1.
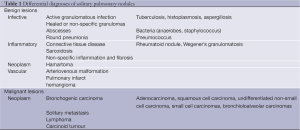
Full table
Traditionally, chest radiography provides basic information about SPN, with regards to lesion size, margin characteristics, calcification, and growth rate on serial X-rays. In current practice, patients with SPN detected on radiographs are much more likely to undergo early CT scan, instead of interval radiograph after trial of empirical antibiotics, particularly in absence of symptoms, because infective bronchopneumonia is a very uncommon cause of SPN, and interval imaging may cause unnecessary delay in diagnosis and treatment of malignant nodules (6). As many as 50% of patients with SPNs detected on radiographs prove to have multiple nodules on CT scanning, which alter the diagnostic evaluation, as multiplicity is suggestive of metastatic or granulomatous disease (10). SPN features are more accurately depicted on CT. Morphologic characteristics of SPN, which are used to correlate with likelihood of malignancy, include size, border, calcification (11). The limit of detectable size changes on plain radiography is estimated to be 3-5 mm, whereas high-resolution CT has a resolution of 0.3 mm (4). Lesion size and growth rate are useful predictors of malignant nodules. Seven studies found proportionately higher risk of malignancy with increasing diameter of pulmonary nodule. Nodules with diameter less than 5 mm, 5 to 10 mm, and greater than 2 cm, are associated with malignancy rates of less than 1%, 6-28%, and 64-82%, respectively (7).
Malignant nodules typically have a doubling time between one month and one year (Figure 1); thus stability in nodule size during a 2-year period is more likely benign (11-14). For spherical masses, a 25% increase in diameter corresponds to a doubling of overall volume. Computer-aided volumetric analysis is widely used, calculating nodule volume to within a 3% accuracy, enabling growth and doubling times to be determined (15).
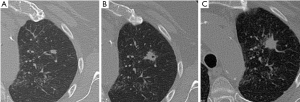
SPNs with irregular, spiculated margins (often described as ‘sunburst’ or ‘corona radiata’ appearance), or lobulated contours, are typically associated with malignancy. Although most SPNs with smooth, well-defined margins are benign, these features are not diagnostic of benignity: 21% of malignant nodules had well-defined margins (16).
Benign patterns of calcification in lung nodules include central, diffuse solid, laminated, and ‘popcorn’; the first three typically found in previous granulomatous infections, and the lattermost characteristic of chondroid calcification in benign hamartoma (16). Intra-nodular fat [–40 to –120 Hounsfield units (HU)], best seen on thin-section high-resolution CT, is also a reliable indicator of benign hamartoma (present in up to 50% of hamartomas) (Figure 2) (16). In the absence of definite benign morphologic features, SPNs are indeterminate, possibly malignant. There is considerable overlap between benign and malignant nodules in terms of morphology in many cases. 25-39% of malignant nodules are inaccurately classified as benign after radiologic assessment of size, margins and internal characteristics (17,18).
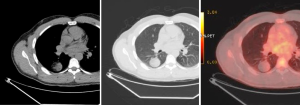
Contrast-enhanced computed tomography
Contrast-enhanced CT improves accuracy of benign versus malignant differentiation of SPNs (sensitivity 98%, specificity 58%, accuracy 77%). After administration of iodinated contrast material intravenously with power injection (300 mg/mL at 2 mL/sec), nodular enhancement of less than 15 HU is strongly predictive of benignity; whereas enhancement of more than 20 HU, reflecting presence of tumour neo-vascularisation, is indicative of malignancy (19).
Higher accuracy is reported for dynamic enhancement evaluation on helical CT, by analyzing combined wash-in and washout characteristics. Malignant nodules show greater washout of contrast enhancement (20). A recent meta-analysis of ten dynamic CT studies reported pooled sensitivity of 93%, specificity of 76%, positive predictive value (PPV) of 80% and negative predictive value (NPV) of 95% for SPN characterization (21). Currently, dynamic contrast-enhanced CT is not widely used in the UK. This technique may be particularly valuable in evaluation of non-calcified SPN in patients with low clinical probability of malignancy, due to its relatively low cost, high sensitivity and NPV.
Positron emission tomography (PET) and combined PET-CT
PET, using 18-fluorine fluoro-deoxyglucose (18F-FDG), a D-glucose analogue labeled with radio-isotope, is a physiologic imaging technique, which can quantify the rate of glucose metabolism by cells, thereby detecting presence of metabolically active tissue. Malignant nodules consist of metabolically active cells that have higher uptake of glucose due to over-expression of glucose transporter protein (Figure 3). FDG is trapped and accumulates within these cells, as the radio-labeled glucose analogue is phosphorylated once but not metabolized further.
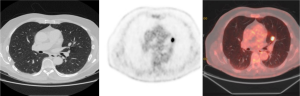
18F-FDG PET alone is reported to be an accurate non-invasive imaging test, with a meta-analysis reporting pooled sensitivity of 96.8% and specificity of 77.8% for malignant nodules (22).
In the 1990s, combined PET and CT scanner with a common gantry and rapid sequencing of CT and PET image acquisitions was developed, which permitted closer imaging registration of the two modalities (23). Integration of anatomic (CT component) and metabolic (PET component) imaging is synergistic by maintaining sensitivity of CT and specificity of PET, resulting in an overall significantly improved accuracy, with 97% sensitivity and 85% specificity, for differentiating malignant from benign SPNs (24).
The current “gold standard” for diagnosing pulmonary nodules is pathology, with tissue obtained either surgically or by percutaneous biopsy, but these techniques are invasive and involve significant risks for the patients (6). Using PET-CT as a diagnostic tool could reduce the number of unnecessary biopsies or thoracotomies on benign SPNs.
PET scans can be interpreted qualitatively (i.e., visual analysis) or semi-quantitatively by using a standardized uptake value (SUV), which is a reflection of the degree of 18F-FDG uptake (25). SUV measurement performed in selected region of interest is reproducible and observer-independent (26).
Bryant et al. found that the maximum SUV (SUVmax) is a predictor of pathology. The higher the SUVmax, the higher chance a nodule would be malignant (27). There is variation in threshold SUVmax used among different institutions for discriminating benign from malignant lesions. Early PET-CT publications frequently adopted 2.5 as a threshold SUVmax (28-31). Yi et al. considered nodules with FDG uptake greater than mediastinal blood-pool or with SUVmax >3.5 as malignant (32). Nguyen et al. compared lesion SUVmax in four anatomical location sites for common indications (pulmonary nodules, head and neck malignancies) and concluded that a common threshold SUVmax did not exist for the different lesion sites. They reported sensitivity and specificity of 81% and 94% respectively using SUVmax >3.6 for SPNs (33).
Kim et al. found that visual interpretation by experienced radiologist or nuclear medicine specialist is sufficient, if not superior, for characterizing SPN, and quantitative analysis (using 2.0 as cut-off SUVmax) did not improve accuracy (24).
There is growing evidence that using threshold SUVmax to differentiate malignant from benign lesions is unrealistic, and SUVmax of 2.5 should not be embraced as a magic threshold (34,35). SUV is a semi-quantitative measurement of glucose uptake by the lesion and is affected by a large number of parameters, which are difficult to control and specify. These include the equipment used, the physics, and biological factors. Partial volume effect, attenuation correction, image noise, image reconstruction method, radiotracer distribution time (time between injection and image acquisition), body size (amount of body fat and brown fat uptake), level of fasting blood glucose, and tracer extravasations, all affect SUV measurements considerably (36). Initiatives, such as the “EANM procedure guidelines for tumour PET imaging”, are useful for standardization to reduce variability in SUV across different centres (37).
Limitations and pitfalls of 18F-FDG PET-CT in characterization of solitary pulmonary nodules
In most studies, the sensitivity of 18F-FDG PET-CT tends to be higher than its specificity for assessment of SPN. This is because FDG, as a marker of glucose metabolism, is not a specific tracer for malignancy. Many benign conditions, such as granulomatous, infective, and inflammatory processes, can mimic malignant nodules and produce false-positive results on PET-CT. In these conditions, FDG uptake is attributed to granulocyte and/or macrophage increased glucose metabolism (38).
In geographical regions where there are high endemic rates of infectious or granulomatous lung disease (for example histoplasmosis or tuberculosis), FDG PET-CT may have significant limitations. A study of 279 patients in south-central United States with high prevalence of histoplasmosis reported specificity of 40% using FDG-PET for diagnosis of lung cancer in pulmonary nodules (39).
On the other hand, false negative results for SPN characterization on PET-CT can occur in three main settings: small lesion size, low tumor metabolic activity, and hyperglycemia (40).
Small lesions (<1 cm) are challenging due to limited spatial resolution of PET, which is approximately 7 mm for modern scanners (8). The partial volume effect leads to considerable underestimation of true intensity or activity within the lesion. In general, negative PET-CT results for nodules smaller than 1 cm, particularly <7 mm, do not confidently exclude malignancy (22).
Tumor histology plays an important part. Some highly differentiated malignant tumors have relatively little metabolic activity and low rate of proliferation, resulting in false-negative PET-CT (Figure 4). It has been suggested that FDG PET is falsely negative in around 50% of patients with bronchioloalveolar carcinoma (BAC) (41), or adenocarcinoma in situ (AIS), as it is currently known after the recent revised classification of lung adenocarcinomas (42). In addition, metastasis from certain primary malignancy, such as renal cell carcinoma, testicular or prostate cancer, may show little FDG tracer accumulation and may even be undetectable on PET-CT (43).
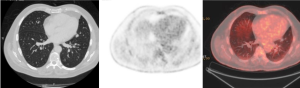
Table 2 lists some of the common false-positive and false-negative nodules on PET-CT.
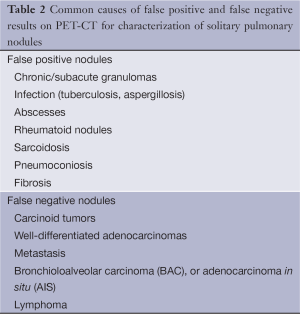
Full table
False negative FDG PET-CT scans may also occur in patients with hyperglycemia. FDG competes with circulating glucose, leading to diminished FDG uptake and accumulation within the malignant lesion. Accurate measurement and documentation of patients’ blood glucose levels for all scans is crucial to allow radiologists and nuclear medicine specialists to recognize this factor limiting scan interpretation.
Dual time point FDG-PET imaging
Increasing number of studies suggests added benefit and improved accuracy of evaluation of SPN by adopting dual time point FDG-PET imaging (DTPI) (44-49). In order to achieve accurate assessment of glucose metabolic activity, SUVmax should be measured once tissue concentration of FDG reaches a plateau. In some malignant tumours, FDG uptake continues to rise beyond 60 minutes and does not reach plateau for several hours (50). The usual image acquisition time is approximately 60 minutes after tracer injection in most centres. If, at this time point, FDG uptake is still occurring in many tissues and a plateau has not been reached, the true FDG accumulation and tumor SUVmax may be significantly underestimated, leading to false-negative results. Therefore, some authors have proposed dual time point FDG-PET imaging, using the change in SUVmax between early and delayed scans to help differentiate benign and malignant SPNs (44-49).
One of the earliest groups comparing single static and dual time point FDG-PET imaging in evaluating lung nodules, Matthies et al., reported achieving a sensitivity of 100%, with specificity of 89%, when they adopted a SUV increase of >10% between first and second scans as a criterion for malignancy (44). The single or first scan was started at a mean time point of 69 minutes, and second delayed scan at mean time point of 122 minutes, after tracer injection.
However, the role of DTPI has been disputed by some authors, most recently Laffon et al. who concluded neither an increase nor decrease in SUV allowed differentiation between malignant and benign nodules, and that DTPI was not suitable for lesions larger than 10 mm and with initial SUVmax >2.5 (51). A meta-analysis on diagnostic performance of DTPI in assessing lung nodules reported summary sensitivity and specificity of 85% and 77% respectively (52), which is similar to single time point FDG-PET, questioning the additive value of DTPI, especially as the technique involves increased time on the PET-CT scanner, higher costs and increased, probably unnecessary, radiation exposure to patients, mainly as the second time point’s CT for attenuation correction.
Other non-conventional techniques for imaging SPNs
Several other imaging techniques have been described for evaluation of SPNs, although these are not widely used in practice.
Dual-energy CT scan can simultaneously provide a virtual non-enhanced and an iodine-enhanced image from a single scan performed after iodine contrast administration, allowing both measurement of nodule enhancement and detection of calcifications. The advantages of this technique include reducing radiation exposure to patients by obviating baseline unenhanced scans, reducing measurement error due to variation in region of interests during subtraction of an unenhanced image from its enhanced counterpart (53).
CT perfusion imaging has been suggested as a promising and feasible method for differentiation of SPNs (54). Shu et al. described first-pass perfusion volume CT technique (perfusion scan in cine pattern, acquisition time of 45 seconds, and delay time of 0 second) and found significantly higher blood volume, permeability surface, and blood flow values in malignant SPN, compared to benign and inflammatory SPN. These quantitative perfusion parameters closely correlate with tumour angiogenesis and expression of vascular endothelial growth factor (VEGF).
Magnetic resonance (MR) imaging of pulmonary nodules is not a routine examination, due to known artefacts that result from tissue-air interfaces and relatively low spatial resolution. Nevertheless, the availability of high-performance gradient systems and parallel imaging techniques has enabled new interesting approaches to MR pulmonary imaging (55). A meta-analysis of six dynamic contrast-enhanced MR studies reported pooled sensitivity of 94%, which is comparable to dynamic contrast-enhanced CT, but with higher pooled specificity of 79% (21,56,57).
Another meta-analysis of 10 studies (with total of 545 patients) on diffusion-weighted MR imaging (DWI) found the technique useful for differentiating malignant and benign lung nodules, with pooled sensitivity and specificity of 84% for both (55), although a cut-off apparent diffusion coefficient (ADC) value for malignant versus benign lesion classification could not be made. This was due to different b values, bias in patient selection, and ADC measurement adopted by the studies. Satoh et al. used a 5-point rank scale (signal intensity of lesion on T2-weighted images compared to that of reference anatomical structures) to score pulmonary nodules, rather than ADC maps, because susceptibility artefacts made it difficult to measure ADC values of lesions located adjacent to air-containing organs (58). Mori et al. found DWI more specific than FDG PET, due to fewer false positives for active inflammation, which does not affect diffusion of water molecules (59). Large-scale randomized controlled trials are still necessary to assess the clinical value of DWI in SPN characterization.
Practical and clinical perspectives of SPN evaluation
Of the various imaging modalities and techniques described in this review, 18F-FDG PET-CT is one of the current mainstays of SPN evaluation. The major obstacles to widespread use of PET-CT are limited availability and high costs.
In the United States, with increasing availability of mobile PET scanners and the reimbursement for SPN evaluation and lung cancer staging with PET scans being supported by Medicare, whole-body PET-CT has become much more commonplace. An observational study found the average US Medicare expenditure for clinical management of a patient with malignant SPN was $50,233 (£30,353) and $22,461 (£13,577) when benign (60). Among the individuals with benign nodule, those with a positive PET scan incurred higher costs, as they were more likely to have surgery and other procedures, and had a higher risk of death.
In the United Kingdom, the National Institute for Clinical Excellence (NICE) currently recommends 18F-FDG PET for investigation of SPNs in cases where a biopsy is not possible or has failed, depending on nodule size, position and CT characterization (61). In a recent audit of local PET-CT centre serving a population of one million with an annual lung cancer incidence of 695 patients, 44 FDG PET-CT scans were requested per year to characterize SPNs. Extrapolated to the UK population, the present demand for FDG PET-CT to characterize SPNs is approximately 2,700 scans per year, equaling to almost 15% of NHS-funded PET-CT scans performed annually in the UK.
Rational use of imaging is crucial. The Fleischner Society guidelines for management of pulmonary nodules are widely adopted, outlining appropriate imaging follow-up at various time intervals, according to patient risk stratification and nodule size (62). Where nodules increase in size, further investigation is indicated, with options such as dynamic contrast-enhanced CT, PET, and/or biopsy, to be considered, although the approach taken would largely depend on local expertise and equipment available. Another key consideration is the patient’s fitness level and co-morbidities, which may limit the options for work-up and subsequent treatment of SPNs.
Gould et al. recommended that tissue diagnosis be attained if CT scans show growth of nodule or patient has high risk of malignancy (6). If indeterminate nodule is larger than 8 mm in a patient with low to moderate risk of malignancy, FDG PET scan is recommended to characterize the nodule further. Moderate-risk group is defined as age >50 and >20 pack-year smoking history or secondhand exposure, and no additional risk factor (radon exposure, occupational exposure, cancer history, family history, or lung cancer). Low-risk individuals are <50 years old and have <20 pack-year smoking history.
Clinical risk stratification is particularly helpful, and the importance of multi-disciplinary approach for clinico-radiological correlation of imaging and PET-CT results must be emphasized. There remain many pitfalls in modern imaging including PET-CT, with false positive and negative nodules. There is risk of overdiagnosis and overtreatment, with patients undergoing unnecessary invasive biopsies for tissue diagnosis in benign pathology. Conversely, delayed or missed diagnoses of lung cancers can occur due to false reassurance offered by false-negative PET-CT in small malignant lesions with low metabolic activity.
Appropriate correlation with clinical risk will more accurately guide subsequent management, with regards to biopsy or intervention. PET-CT cannot replace “gold standard” pathology by resection or percutaneous biopsy. This is highlighted by two studies analyzing solitary pulmonary nodules in patients with history of malignancy, both advocating surgical resection of SPN for definitive diagnosis of metastasis versus primary lung cancer, or benign lesions, as this has significant prognostic value; although there is selection bias for patients with good general health and fit for surgery (63,64).
PET-CT can be considered an important part of the overall risk stratification of SPNs. Higher FDG uptake in lung cancer as measured by SUV analysis is associated with aggressive cancers and shorter survival (median survival 20 months), compared to longer survival of >75 months in lesions with low FDG uptake (65). In patients with high risk for aggressive intervention or surgery, low FDG uptake in an SPN may help clinicians decide to omit invasive diagnostic thoracotomy. Lower levels of FDG uptake often correspond to histologically and clinically less aggressive tumour behavior (66).
Integrated PET-CT is and will continue to be a valuable non-invasive imaging tool for evaluation of SPNs. PET-CT provides vital information on anatomy, nodule morphology, and metabolic activity in a single integrated scan. An added value of PET-CT is the detection of other unexpected metabolically active lesion and/or lymphadenopathy to support probable diagnosis of SPN being a primary or secondary malignancy (Figure 5).
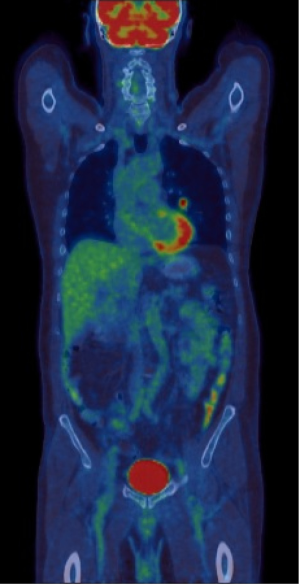
Summary
Increasing detection of SPNs is inevitable. Large number of these nodules will be indeterminate on imaging, become a diagnostic dilemma for clinicians and radiologists, and lead to patient anxiety. Contrast-enhanced CT, interval CT for ‘stability’ assessment, and 18F-FDG PET-CT are the most widely used modalities in radiological evaluation of SPNs. Various emerging novel imaging techniques are on the horizon, each with its own strengths and pitfalls, all aiming to improve the accuracy of non-invasive diagnosis of SPNs.
As aptly summarized in the most recent American College of Radiology (ACR) appropriateness criteria for solitary pulmonary nodule, there is no single algorithm or ‘one-size-fits-all’ pathway for work-up of SPNs (67).
Depending on the regional prevalence of lung disease and cancer, equipment and skill levels available, an ideal diagnostic algorithm should incorporates best judicious use of local clinical and radiological resources for risk stratification, maximizing non-surgical tissue diagnosis by percutaneous or transbronchial biopsy, in order to achieve accurate diagnosis of SPN.
Acknowledgements
Disclosure: The authors declare no conflict of interest.
References
- Furtado CD, Aguirre DA, Sirlin CB, et al. Whole-body CT screening: spectrum of findings and recommendations in 1192 patients. Radiology 2005;237:385-94. [PubMed]
- Swensen SJ, Jett JR, Hartman TE, et al. Lung Cancer screening with CT: Mayo Clinic experience. Radiology 2003;226:756-61. [PubMed]
- Hansell DM, Bankier AA, Macmahon H, et al. Fleischner society: glossary of terms for thoracic imaging. Radiology 2008;246:697-722. [PubMed]
- Ost D, Fein AM, Feinsilver SH. Clinical practice. The solitary pulmonary nodule. N Engl J Med 2003;348:2535-42. [PubMed]
- National Lung Screening Trial Research Team, Aberle DR, Adams AM, et al. Reduced lung-cancer mortality with low-dose computed tomographic screening. N Engl J Med 2011;365:395-409. [PubMed]
- Gould MK, Fletcher J, Iannettoni MD, et al. Evaluation of patients with pulmonary nodules: when is it lung cancer?: ACCP evidence-based clinical practice guidelines (2nd edition). Chest 2007;132:108S-130S.
- Wahidi MM, Govert JA, Goudar RK, et al. Evidence for the treatment of patients with pulmonary nodules: when is it lung cancer?: ACCP evidence-based clinical practice guidelines (2nd edition). Chest 2007;132:94S-107S.
- Tan BB, Flaherty KR, Kazerooni EA, et al. The solitary pulmonary nodule. Chest 2003;123:89S-96S. [PubMed]
- Ferlay J, Parkin DM, Steliarova-Foucher E. Estimates of Cancer incidence and mortality in Europe in 2008. Eur J Cancer 2010;46:765-81. [PubMed]
- Leef JL 3rd, Klein JS. The solitary pulmonary nodule. Radiol Clin North Am 2002;40:123-43. [PubMed]
- Albert RH, Russell JJ. Evaluation of the solitary pulmonary nodule. Am Fam Physician 2009;80:827-31. [PubMed]
- Yankelevitz DF, Henschke CI. Does 2-year stability imply that pulmonary nodules are benign? AJR Am J Roentgenol 1997;168:325-8. [PubMed]
- Takashima S, Sone S, Li F, et al. Small solitary pulmonary nodules (< or =1 cm) detected at population-based CT screening for lung Cancer: Reliable high-resolution CT features of benign lesions. AJR Am J Roentgenol 2003;180:955-64. [PubMed]
- Midthun DE, Swensen SJ, Jett JR. Approach to the solitary pulmonary nodule. Mayo Clin Proc 1993;68:378-85. [PubMed]
- Yankelevitz DF, Reeves AP, Kostis WJ, et al. Small pulmonary nodules: volumetrically determined growth rates based on CT evaluation. Radiology 2000;217:251-6. [PubMed]
- Erasmus JJ, Connolly JE, McAdams HP, et al. Solitary pulmonary nodules: Part I. Morphologic evaluation for differentiation of benign and malignant lesions. Radiographics 2000;20:43-58. [PubMed]
- Erasmus JJ, McAdams HP, Connolly JE. Solitary pulmonary nodules: Part II. Evaluation of the indeterminate nodule. Radiographics 2000;20:59-66. [PubMed]
- Gurney JW. Determining the likelihood of malignancy in solitary pulmonary nodules with Bayesian analysis. Part I. Theory. Radiology 1993;186:405-13. [PubMed]
- Swensen SJ, Viggiano RW, Midthun DE, et al. Lung nodule enhancement at CT: multicenter study. Radiology 2000;214:73-80. [PubMed]
- Jeong YJ, Lee KS, Jeong SY, et al. Solitary pulmonary nodule: characterization with combined wash-in and washout features at dynamic multi-detector row CT. Radiology 2005;237:675-83. [PubMed]
- Cronin P, Dwamena BA, Kelly AM, et al. Solitary pulmonary nodules: meta-analytic comparison of cross-sectional imaging modalities for diagnosis of malignancy. Radiology 2008;246:772-82. [PubMed]
- Gould MK, Maclean CC, Kuschner WG, et al. Accuracy of positron emission tomography for diagnosis of pulmonary nodules and mass lesions: a meta-analysis. JAMA 2001;285:914-24. [PubMed]
- Townsend DW, Beyer T. A combined PET/CT scanner: the path to true image fusion. Br J Radiol 2002;75:S24-30. [PubMed]
- Kim SK, Allen-Auerbach M, Goldin J, et al. Accuracy of PET/CT in characterization of solitary pulmonary lesions. J Nucl Med 2007;48:214-20. [PubMed]
- Lowe VJ, Hoffman JM, DeLong DM, et al. Semiquantitative and visual analysis of FDG-PET images in pulmonary abnormalities. J Nucl Med 1994;35:1771-6. [PubMed]
- Nahmias C, Wahl LM. Reproducibility of standardized uptake value measurements determined by 18F-FDG PET in malignant tumors. J Nucl Med 2008;49:1804-8. [PubMed]
- Bryant AS, Cerfolio RJ. The maximum standardized uptake values on integrated FDG-PET/CT is useful in differentiating benign from malignant pulmonary nodules. Ann Thorac Surg 2006;82:1016-20. [PubMed]
- Al-Sugair A, Coleman RE. Applications of PET in lung Cancer. Semin Nucl Med 1998;28:303-19. [PubMed]
- Lowe VJ, Fletcher JW, Gobar L, et al. Prospective investigation of positron emission tomography in lung nodules. J Clin Oncol 1998;16:1075-84. [PubMed]
- Hashimoto Y, Tsujikawa T, Kondo C, et al. Accuracy of PET for diagnosis of solid pulmonary lesions with 18F-FDG uptake below the standardized uptake value of 2.5. J Nucl Med 2006;47:426-31. [PubMed]
- Hain SF, Curran KM, Beggs AD, et al. FDG-PET as a “metabolic biopsy” tool in thoracic lesions with indeterminate biopsy. Eur J Nucl Med 2001;28:1336-40. [PubMed]
- Yi CA, Lee KS, Kim BT, et al. Tissue characterization of solitary pulmonary nodule: comparative study between helical dynamic CT and integrated PET/CT. J Nucl Med 2006;47:443-50. [PubMed]
- Nguyen NC, Kaushik A, Wolverson MK, et al. Is there a common SUV threshold in oncological FDG PET/CT, at least for some common indications? A retrospective study. Acta Oncol 2011;50:670-7. [PubMed]
- Kwee TC, Cheng G, Lam MG, et al. SUVmax of 2.5 should not be embraced as a magic threshold for separating benign from malignant lesions. Eur J Nucl Med Mol Imaging 2013;40:1475-7. [PubMed]
- Sim YT, Goh YG, Dempsey MF, et al. PET-CT evaluation of solitary pulmonary nodules: correlation with maximum standardized uptake value and pathology. Lung 2013;191:625-32. [PubMed]
- Thie JA. Understanding the standardized uptake value, its methods, and implications for usage. J Nucl Med 2004;45:1431-4. [PubMed]
- Boellaard R, O’doherty MJ, Weber WA, et al. FDG PET and PET/CT: EANM procedure guidelines for tumour PET imaging: version 1.0. Eur J Nucl Med Mol Imaging 2010;37:181-200. [PubMed]
- Ambrosini V, Nicolini S, Caroli P, et al. PET/CT imaging in different types of lung Cancer: an overview. Eur J Radiol 2012;81:988-1001. [PubMed]
- Deppen S, Putnam JB Jr, Andrade G, et al. Accuracy of FDG-PET to diagnose lung Cancer in a region of endemic granulomatous disease. Ann Thorac Surg 2011;92:428-32; discussion 433. [PubMed]
- Bar-Shalom R, Valdivia AY, Blaufox MD. PET imaging in oncology. Semin Nucl Med 2000;30:150-85. [PubMed]
- Orlacchio A, Schillaci O, Antonelli L, et al. Solitary pulmonary nodules: morphological and metabolic characterisation by FDG-PET-MDCT. Radiol Med 2007;112:157-73. [PubMed]
- Travis WD, Brambilla E, Noguchi M, et al. International association for the study of lung Cancer/American thoracic society/European respiratory society international multidisciplinary classification of lung adenocarcinoma. J Thorac Oncol 2011;6:244-85. [PubMed]
- Van Tassel D, Tassel LV, Gotway MB, et al. Imaging evaluation of the solitary pulmonary nodule. Clin Pulm Med 2011;18:274-99.
- Matthies A, Hickeson M, Cuchiara A, et al. Dual time point 18F-FDG PET for the evaluation of pulmonary nodules. J Nucl Med 2002;43:871-5. [PubMed]
- Xiu Y, Bhutani C, Dhurairaj T, et al. Dual-time point FDG PET imaging in the evaluation of pulmonary nodules with minimally increased metabolic activity. Clin Nucl Med 2007;32:101-5. [PubMed]
- Alkhawaldeh K, Bural G, Kumar R, et al. Impact of dual-time-point (18)F-FDG PET imaging and partial volume correction in the assessment of solitary pulmonary nodules. Eur J Nucl Med Mol Imaging 2008;35:246-52. [PubMed]
- Macdonald K, Searle J, Lyburn I. The role of dual time point FDG PET imaging in the evaluation of solitary pulmonary nodules with an initial standard uptake value less than 2.5. Clin Radiol 2011;66:244-50. [PubMed]
- Yang P, Xu XY, Liu XJ, et al. The value of delayed (18)F FDG-PET imaging in diagnosis of solitary pulmonary nodules: A preliminary study on 28 patients. Quant Imaging Med Surg 2011;1:31-4. [PubMed]
- Lan XL, Zhang YX, Wu ZJ, et al. The value of dual time point (18)F-FDG PET imaging for the differentiation between malignant and benign lesions. Clin Radiol 2008;63:756-64. [PubMed]
- Hamberg LM, Hunter GJ, Alpert NM, et al. The dose uptake ratio as an index of glucose metabolism: useful parameter or oversimplification? J Nucl Med 1994;35:1308-12. [PubMed]
- Laffon E, de Clermont H, Begueret H, et al. Assessment of dual-time-point 18F-FDG-PET imaging for pulmonary lesions. Nucl Med Commun 2009;30:455-61. [PubMed]
- Barger RL, Nandalur KR. Diagnostic performance of dual-time 18F-FDG PET in the diagnosis of pulmonary nodules: a meta-analysis. Acad Radiol 2012;19:153-8. [PubMed]
- Chae EJ, Song JW, Krauss B, et al. Dual-energy computed tomography characterization of solitary pulmonary nodules. J Thorac Imaging 2010;25:301-10. [PubMed]
- Shu SJ, Liu BL, Jiang HJ. Optimization of the scanning technique and diagnosis of pulmonary nodules with first-pass 64-detector-row perfusion VCT. Clin Imaging 2013;37:256-64. [PubMed]
- Wu LM, Xu JR, Hua J, et al. Can diffusion-weighted imaging be used as a reliable sequence in the detection of malignant pulmonary nodules and masses? Magn Reson Imaging 2013;31:235-46. [PubMed]
- Ohno Y, Hatabu H, Takenaka D, et al. Solitary pulmonary nodules: potential role of dynamic Mr imaging in management initial experience. Radiology 2002;224:503-11. [PubMed]
- Schaefer JF, Vollmar J, Schick F, et al. Solitary pulmonary nodules: dynamic contrast-enhanced Mr imaging--perfusion differences in malignant and benign lesions. Radiology 2004;232:544-53. [PubMed]
- Satoh S, Kitazume Y, Ohdama S, et al. Can malignant and benign pulmonary nodules be differentiated with diffusion-weighted MRI? AJR Am J Roentgenol 2008;191:464-70. [PubMed]
- Mori T, Nomori H, Ikeda K, et al. Diffusion-weighted magnetic resonance imaging for diagnosing malignant pulmonary nodules/masses: comparison with positron emission tomography. J Thorac Oncol 2008;3:358-64. [PubMed]
- Barnett PG, Ananth L, Gould MK, et al. Cost and outcomes of patients with solitary pulmonary nodules managed with PET scans. Chest 2010;137:53-9. [PubMed]
- National Institute for Health and Clinical Excellence. The diagnosis and treatment of lung cancer (update of NICE clinical guideline 24). Available online: www.nice.org.uk/guidance/index.jsp?action=byID&o=13456 (last accessed 15/07/2013).
- Macmahon H, Austin JH, Gamsu G, et al. Guidelines for management of small pulmonary nodules detected on CT scans: a statement from the Fleischner Society. Radiology 2005;237:395-400. [PubMed]
- Sakamoto M, Murakawa T, Kitano K, et al. Resection of solitary pulmonary lesion is beneficial to patients with a history of malignancy. Ann Thorac Surg 2010;90:1766-71. [PubMed]
- Rena O, Davoli F, Boldorini R, et al. The solitary pulmonary nodule in patients with previous Cancer history: results of surgical treatment. Eur J Surg Oncol 2013;39:1248-53. [PubMed]
- Grgic A, Yüksel Y, Gröschel A, et al. Risk stratification of solitary pulmonary nodules by means of PET using (18)F-fluorodeoxyglucose and SUV quantification. Eur J Nucl Med Mol Imaging 2010;37:1087-94. [PubMed]
- Kwee TC, Basu S, Saboury B, et al. A new dimension of FDG-PET interpretation: assessment of tumor biology. Eur J Nucl Med Mol Imaging 2011;38:1158-70. [PubMed]
- Kanne JP, Jensen LE, Mohammed TL, et al. ACR appropriateness Criteria® radiographically detected solitary pulmonary nodule. J Thorac Imaging 2013;28:W1-3. [PubMed]