Personalized 3D printed coronary models in coronary stenting
Introduction
Coronary computed tomography angiography (CCTA) is a widely used less-invasive modality in the diagnostic assessment of coronary artery disease. Due to technological improvements in CT scanning techniques, CCTA has demonstrated high diagnostic accuracy in determining coronary stenosis and serves as a reliable gatekeeper for excluding obstructive coronary stenosis, even in patients with high heart rates (1-7). Coronary stenting is a commonly performed less invasive procedure following percutaneous coronary intervention for the treatment of patients with significant coronary artery stenosis, while stent patency and presence of in-stent restenosis is increasingly evaluated with CCTA, according to several systematic reviews and meta-analyses (8-13). Despite reported high sensitivity and specificity of CCTA, the clinical application of CCTA for evaluation of coronary in-stent restenosis remains questionable because of beam-hardening artifacts which result in high false positive rates, leading to low positive predictive value, especially when assessing stents with diameter less than 3.0 mm (14,15).
The recent introduction of third generation dual-source (DSCT) with improved spatial and temporal resolution has enhanced the visualization of coronary stent lumen and increased the diagnostic value of CCTA in detecting in-stent restenosis based on in vitro phantom and patient studies (16-19). Furthermore, use of different convolution kernels or postprocessing image reconstructions have been shown to reduce beam hardening or blooming artifacts associated with metal stent components, thus improving the diagnostic image quality of CCTA in coronary stenting (20,21). The purpose of this study was to simulate coronary stenting by placing coronary stents with different diameters into the three-dimensional (3D) printed coronary artery models and acquire images on the third generation DSCT scanner. The innovative aspect of this study lies in utilizing personalized 3D printed models to simulate the coronary stenting procedure and scanning the models with latest CT scanner with images reconstructed with different imaging parameters. 3D printing is increasingly used in medical applications with promising reports in the domain of congenital heart disease and aortic disease (22-30). To the best of our knowledge, this is the first study using 3D printed coronary models with stents inserted inside the coronary arteries for determining the feasibility of coronary stenting procedure.
Methods
3D printed coronary artery models
Patient-specific 3D printed coronary models were created using de-identified CCTA images with models printed by soft and elastic material, TangoPlus. Details about these 3D printed models were reported in our previous publication (31). In brief, each model has right coronary artery (RCA) and left coronary arteries (either left anterior descending-LAD, or left circumflex-LCx or both of these branches). Figure 1 shows these 3D printed coronary models that were used in the experiments.
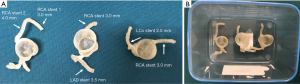
Coronary stent placement
Six balloon expandable expired coronary stents with different diameters and lengths were used to simulate coronary stenting. Stent details are summarized in Table 1.
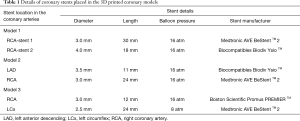
Full table
Each stent was placed into the 3D printed coronary artery to simulate the interventional procedure for treatment of coronary artery disease. Although the stents were not visible from the outside of the model, all were deployed according to recommended balloon inflation pressures on the instructions for use (IFU). Optimal deployment was therefore assumed as it could not be directly visualized. Of the 6 coronary stents, 2 were placed at the RCA (proximal and distal segments) in the model 1, and 1 in RCA and 1 in distal LAD in the model 2, 1 in RCA and 1 in proximal LCx in the model 3. The models were placed in a plastic container which was positioned on the CT scanner table parallel to the z-axis. Figure 2 shows 3D volume rendering images of stent locations in the 3D printed coronary artery branches.
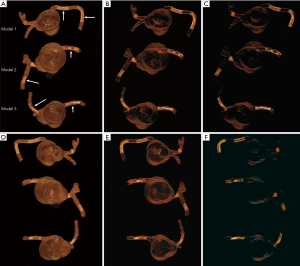
Coronary CT scanning protocols and image reconstruction
CT scan was performed on a 192-slice 3rd generation DSCT scanner (Siemens Force, Siemens Healthcare, Forchheim, Germany) without using electrocardiographic gating due to the static nature of the models. The following parameters were used for scans: beam collimation: 2×192× 0.6 mm with gantry rotation of 250 s, tube potential of 70 kV and tube current of 20 mA with resultant CTDIvol of 0.06 mGy and dose length product (DLP) of 2 mGy·cm−1.
All images were acquired with a slice thickness of 0.5 mm and 0.25 mm reconstruction interval, resulting in the voxel size of 0.25×0.25×0.25 mm3 for volumetric data. Images were reconstructed with advanced modelled iterative reconstruction (ADMIRE) algorithm using the level 3 strength.
For visualization of both coronary artery and stent lumen, two sets of axial images were reconstructed with smooth kernel (Bv36) and sharp kernel (Bv59). Images (2D and 3D) reconstructed with smooth kernel were used for assessment of coronary artery lumen with stents placed in the coronary arteries, while images reconstructed with sharp kernel (only 2D axial images) were used for evaluation of stent details, especially in the coronary stenting regions where multiple stent wires overlapped resulting in significant beam hardening artifacts.
Further to the use of different reconstruction kernels, images were reconstructed with thick slab maximum-intensity projection (MIP) and thin slab MIP images with corresponding parameters of 3.0 mm slice thickness and 1.5 mm reconstruction interval, 1.5 mm slice thickness and 0.75 mm reconstruction interval, respectively. This aims to determine the effect of different MIP reconstructions on the visualization and assessment of coronary stent lumen.
2D and 3D image visualization and assessment
CCTA images in digital imaging and communications in medicine (DICOM) format were transferred to a separate workstation for image postprocessing and analysis. 2D and 3D volume rendering reconstructions were performed using an open source RadiAnt DICOM viewer (64-bit) with measurement of stent lumen and stent diameter on 2D axial and 3D volume rendering images (Figure 3). All measurements were performed by an observer with more than 15 years of experience in interpreting cardiac CT images. Three measurements were repeated at each stent location with the mean values used to minimize intra-observer variability.
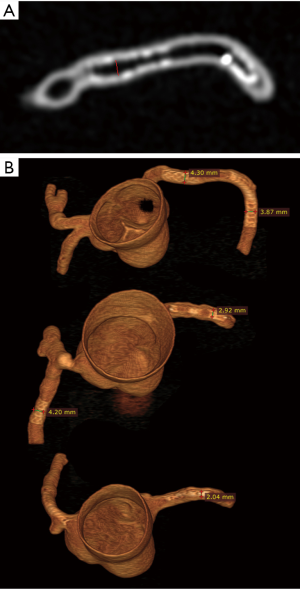
3D virtual intravascular endoscopy (VIE) views were also generated using a commercially available software Analyze 12.0 (AnalyzeDirect, Inc., Lexana, KS, USA) in the images reconstructed with soft kernel Bv36 algorithm to provide intraluminal views of the coronary stents in relation to the coronary wall for determining the position of stent placement, stent patency and configuration. Details of producing VIE images have been well described in our previous studies (32-35).
Statistical analysis
Quantitative variables were expressed as mean ± standard deviation. A two-tailed T test with P value <0.05 was used to determine statistical significance. All statistical analyses were performed using SPSS 24.0 (IBM Corporation, Armonk, NY, USA).
Results
The mean stent lumen diameters measured with different image reconstructions are presented in Table 2. The stent lumen visibility ranged from 54% to 97%, depending on the location of the stent in the coronary arteries. Three stents gave high visibility between 72% and 97%, with more than 86% visibility assessed by these reconstruction images in the LCx stent in the model 3. Relatively low visibility was seen in the other 3 stents ranging from 54% to 77%, in particular in the two stents which failed to achieve wall apposition consistently along their length resulting in the lowest visibility.
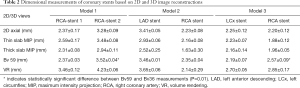
Full table
The lumen diameters were found to be significantly smaller than the actual sizes when measurements were performed on 2D axial, thin and thick slab MIP images (P<0.01). In contrast, volume rendering images allowed for more accurate measurements with measured stent lumen close to the actual dimensions of these coronary stents, except for the stent placed at the RCA in model 2, with similar measurements among these 2D and 3D reconstructions (P=0.65–0.94). This is mainly due to the reason that the stent was not fully expanded in the model during deployment.
Thick slab MIP images resulted in measured stent lumen diameters smaller than those from thin slab MIP images, with significant differences noticed in the measurements of the 4 stents in the models 1 and 2 (P<0.05), with no significant differences in the remaining 2 stents in the model 3 (P=0.19–0.38). Figure 4 shows visualization of coronary stents with images reconstructed with thin and thick slab MIP. Significant improvements of assessing stent lumen are observed in most of the images with use of thin slab MIP as opposed to the thick slab images.
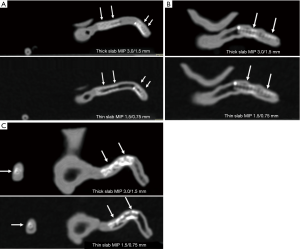
Stent lumen visualization was significantly improved in images reconstructed with sharp kernel algorithm, and this is especially apparent in the evaluation of stents at the RCA in the models 1 and 3 (P=0.01). Figure 5 shows 2D axial images reconstructed with smooth kernel Bv36, while Figure 6A are 2D axial images reconstructed with sharp kernel Bv59, with clear visualization of the right coronary stent in the model 3. Figure 6B shows the 3D volume rendering view of metal stent components with use of sharp kernel algorithm with improved stent lumen visibility. Use of sharp kernel algorithm resulted in stent lumen visibility ranging from 68% to 98%.
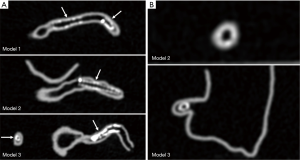
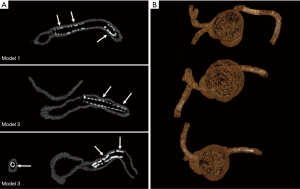
3D VIE views were successfully generated in all images with clear demonstration of the intravascular appearances of coronary wall, stent lumen and stent position inside the coronary arteries. Figure 7 demonstrates the VIE images of the stents at the RCA in the model 1 with stent patent and smooth coronary wall, while Figure 8A,B shows the lack of wall apposition between the coronary wall and the RCA stent in the model 2 because of insufficient expansion of the stent. The LCx stent was shown patent with circular appearance (Figure 8C). The LAD stent in the model 3 was revealed as circular and smooth appearance, while the RCA stent was shown to be irregularly placed inside the coronary artery with lack of wall apposition between the stent and the coronary wall due to insufficient expansion (Figure 9).
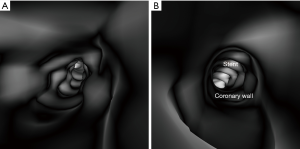
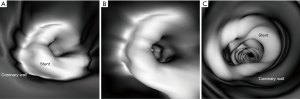
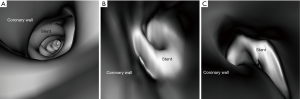
Discussion
In this preliminary study, we have demonstrated the feasibility of simulating coronary stenting in 3D printed coronary artery models with good stent lumen visibility based on images acquired with the latest third generation DSCT. With use of different image postprocessing kernels, visualization of coronary stents has been significantly improved when sharp kernel is applied, especially in the stenting areas with high degree of stent wire overlapping or presence of multiple stent wires. Findings of this study can be used to develop optimal CCTA protocols in coronary stenting.
Invasive coronary angiography still remains the gold standard for assessment of coronary lumen and detection of in-stent restenosis, however, given its invasive nature and potential risk of procedure-related morbidity and mortality, CCTA is a promising less-invasive modality in the follow-up of coronary stenting with improved scanning techniques available with latest scanners. Recent studies using advanced CT scanners such as high definition CT or third generation DSCT have reported the improved diagnostic value of coronary restenosis following coronary stenting (16-18). Results of our study are consistent with these findings, as we have shown the improved stent lumen visibility using the third generation DSCT. The stent lumen visibility in this study was higher than a recent report with in vitro evaluation of 24 stents (45–55% visibility for more than 50% of the stents evaluated), and this is most likely because of their study being conducted on a 128-slice CT (14). Given only a small number of stents placed in these coronary arteries in the 3D printed models, further research with assessment of more stents is needed to confirm our findings.
Another approach to reduce the effect of beam hardening or blooming artifacts resulting from stents is to apply reconstruction techniques or postprocessing algorithms which has been shown to greatly enhance the diagnostic performance of CCTA in coronary stent assessment (21). When compared to the smooth kernel Bv36, the sharp kernel Bv59 showed significant improvements in stent lumen visibility, which is clearly demonstrated by assessing the RCA stent in the model 3 (Figure 6). The mean stent lumen measured with Bv59 was superior to the other image reconstructions (Table 2), with measured lumen diameter close to the actual size of 3.0 mm. Despite improved lumen visibility, the sharp kernel algorithm affects the visualization of soft tissue details such as coronary wall as shown in Figure 6B, thus, it is recommended for only assessing the stent lumen visibility.
3D printing has shown great promise in medical applications with increasing reports in cardiovascular disease, primarily in assisting pre-operative planning and simulation of surgical procedures, improving medical education and enhancing doctor-patient communication (23-30). Another emerging area of utilizing personalized 3D printed models in cardiovascular imaging is to develop optimal CT scanning protocols. Only a few studies are available in the literature with regard to testing different imaging parameters on 3D printed heart and pulmonary artery models (36-38). To our knowledge, this is the first study reporting the use of 3D printed coronary models for investigation of CCTA in coronary stenting. Although this study represents a preliminary report with simulation of only a few stents in the 3D printed coronary arteries, results are promising as it is feasible to place these stents in the 3D printed realistic models, therefore, encouraging further experiments to identify optimal protocols in coronary CT stenting.
There are some limitations in this study. First, only 6 stents were tested in this study, and more stents with different diameters such as inclusion of 2.25 and 2.75 mm stents and various stent materials are needed. Second, despite using a patient-specific 3D printed coronary model, no simulation of contrast enhancement or electrocardiographic gating was performed. This could contribute to the high stent lumen visibility achieved with this study. Hence, results need to be interpreted with caution. Further, for simulation of the realistic anatomical environment, the coronary models should be placed in the thorax with inclusion of other anatomical structures such as lungs, ribs and heart. This could be addressed in future experimental design. Finally, in-stent restenosis was not simulated in this study. The main application of CCTA in coronary stenting is to detect in-stent restenosis so that early treatment can be offered to patients to reduce the risk of cardiac events. This is currently being investigated in our ongoing study by simulation of in-stent restenosis in 3D printed coronary models.
In conclusion, we have presented our preliminary experience of utilizing personalized 3D coronary artery models for placement of a number of stents in the coronary arteries by demonstrating the feasibility of visualizing the stent lumen using images reconstructed with different parameters. Visibility of the stent lumen overall is good, regardless of the stent diameter. Application of a sharp kernel algorithm improves stent lumen visibility, especially in areas with multiple stent wires. This study provides guidance for future experimental design with the aim of developing optimal coronary CT angiography protocols after coronary stenting.
Acknowledgments
We would like to thank Mr. Werner Ducke from Cook Medical Australia for providing these coronary stents that were used in the experiments. We also thank Mr. Tom Tiang from Perth Children’s Hospital for his assistance in CT scanning.
Funding: This work was supported by National Heart Foundation of Australia (No. 101843).
Footnote
Conflicts of Interest: The authors have no conflicts of interest to declare.
References
- Barth S, Marwan M, Hausleiter J, Moshage W, Korosoglou G, Leber A, Schmermund A, Gohlke H, Bruder O, Dill T, Schröder S, Kerber S, Hamm K, Gietzen F, Schneider S, Senges J, Achenbach S. Coronary computed tomography angiography (CCTA) in patients with suspected stable coronary artery disease (CAD): diagnostic impact and clinical consequences in the German Cardiac CT Registry depending on stress test results. Int J Cardiovasc Imaging 2019;35:741-8. [Crossref] [PubMed]
- Abidov A, Gallagher MJ, Chinnaiyan KM, Mehta LS, Wegner JH, Raff GL. Clinical effectiveness of coronary computed tomographic angiography in the triage of patients to cardiac catheterization and revascularization after inconclusive stress testing: results of a 2-year prospective trial. J Nucl Cardiol 2009;16:701-13. [Crossref] [PubMed]
- Sun Z, Al Moudi M, Cao Y. CT angiography in the diagnosis of cardiovascular disease: a transformation in cardiovascular CT practice. Quant Imaging Med Surg 2014;4:376-96. [PubMed]
- Rubin GD, Leipsic J, Joseph Schoepf U, Fleischmann D, Napel S. CT angiography after 20 years: a transformation in cardiovascular disease characterization continues to advance. Radiology 2014;271:633-52. [Crossref] [PubMed]
- Sun Z, Ng KH. Diagnostic value of coronary CT angiography with prospective ECG-gating in the diagnosis of coronary artery disease: A systematic review and meta-analysis. Int J Cardiovasc Imaging 2012;28:2109-19. [Crossref] [PubMed]
- Liang J, Wang H, Xu L, Yang L, Dong L, Fan Z, Wang R, Sun Z. Diagnostic performance of 256-row detector coronary CT angiography in patients with high heart rates within a single cardiac cycle: a preliminary study. Clin Radiol 2017;72:694.e7-14. [Crossref] [PubMed]
- Liang J, Wang H, Xu L, Dong L, Fan Z, Wang R, Sun Z. Impact of SSF on diagnostic performance of coronary computed tomography angiography within 1 heart beat in patients with high heart rate using a 256-row detector computed tomography. J Comput Assist Tomogr 2018;42:54-61. [Crossref] [PubMed]
- Sun Z, Almutairi AM. Diagnostic accuracy of 64 multislice CT angiography in the assessment of coronary in-stent restenosis: A meta-analysis. Eur J Radiol 2010;73:266-73. [Crossref] [PubMed]
- Sun Z, Davidson R, Lin CH. Multi-detector row CT angiography in the assessment of coronary in-stent restenosis: A systematic review. Eur J Radiol 2009;69:489-95. [Crossref] [PubMed]
- Kumbhani DJ, Ingelmo CP, Schoenhagen P, Curtin RJ, Flamm SD, Desai MY. Meta-analysis of diagnostic efficacy of 64-slice computed tomography in the evaluation of coronary in-stent restenosis Am J Cardiol 2009;103:1675-81. [Crossref] [PubMed]
- Andreini D, Pontone G, Mushtaq S, Pepi M, Bartorelli A. Multidetector computed tomography coronary angiography for the assessment of coronary in-stent restenosis Am J Cardiol 2010;105:645-55. [Crossref] [PubMed]
- Liu WJ, Li GZ, Liu HF, Lei JQ. Diagnostic accuracy of dual-source computed tomography angiography for the detection of coronary in-stent restenosis: a systematic review and meta-analysis. Echocardiography 2018;35:541-50. [Crossref] [PubMed]
- Liu HF, Wang M, Xu YS, Shrestha MK, Lei JQ. Diagnostic accuracy of dual-source and 320-row computed tomography angiography in detecting coronary in-stent restenosis: a systematic review and meta-analysis. Acta Radiol 2019;60:149-59. [Crossref] [PubMed]
- Hickethier T, Wenning J, Doerner J, Maintz D, Michels G, Bunck AC. Fourth update on CT angiography of coronary stents: in vitro evaluation of 24 novel stent types. Acta Radiol 2018;59:1060-5. [Crossref] [PubMed]
- Andreini D, Pontone G, Mushtaq S, Conte E, Guglielmo M, Mancini ME, Annoni A, Baggiano A, Formenti A, Montorsi P, Magatelli M, Di Odoardo L, Melotti E, Resta M, Muscogiuri G, Fiorentini C, Bartorelli AL, Pepi M. Diagnostic accuracy of coronary CT angiography performed in 100 consecutive patients with coronary stents using a whole-organ high-definition CT scanner. Int J Cardiol 2019;274:382-7. [Crossref] [PubMed]
- Eckert J, Renczes-Janetzko P, Schmidt M, Magedanz A, Voigtlander T, Schmermund A. Coronary CT angiography (CCTA) using third-generation dual-source CT for ruing out in-stent restenosis. Clin Res Cardiol 2019;108:402-10. [Crossref] [PubMed]
- Li Y, Yu M, Li W, Lu Z, Wei M, Zhang J. Third generation dual-source CT enables accurate diagnosis of coronary restenosis in all size stents with low radiation dose and preserved image quality. Eur Radiol 2018;28:2647-54. [Crossref] [PubMed]
- Mangold S, Cannaó PM, Schoepf UJ, Wichmann JL, Canstein C, Fuller SR, Muscogiuri G, Varga-Szemes A, Nikolaou K, de Cecco CN. Impact of an advanced image-based monoenergetic reconstruction algorithm on coronary stent visualization using third generation dual-source dual-energy CT: a phantom study. Eur Radiol 2016;26:1871-8. [Crossref] [PubMed]
- Gassenmaier T, Petri N, Allmendinger T, Flohr T, Maintz D, Voelker W, Bley TA. Next generation coronary CT angiography: in vitro evaluation of 27 coronary stents. Eur Radiol 2014;24:2953-61. [Crossref] [PubMed]
- Tatsugami F, Higaki T, Sakane H, Nakamura Y, Iida M, Baba Y, Fujioka C, Senoo A, Kitagawa T, Yamamoto H, Kihara Y, Awai K. Diagnostic accuracy of in-stent restenosis using model-based iterative reconstruction at coronary CT angiography: initial experience. Br J Radiol 2018;91:20170598. [PubMed]
- Ulrich A, Burg MC, Raupach R, Bunck A, Schuelke C, Maintz D, Heindel W, Seifarth H. Coronary stent imaging with dual-source CT: assessment of lumen visibility using different convolution kernels and postprocessing filters. Acta Radiol 2015;56:42-50. [Crossref] [PubMed]
- Sun Z, Squelch A. Patient-specific 3D printed models of aortic aneurysm and aortic dissection. J Med Imaging Health Inf 2017;7:886-9. [Crossref]
- Sun Z, Lee S. A systematic review of 3D printing in cardiovascular and cerebrovascular diseases. Anatol J Cardiol 2017;17:423-35. [PubMed]
- Kiraly L. Three-dimensional modelling and three-dimensional printing in pediatric and congenital cardiac surgery. Transl Pediatr 2018;7:129-38. [Crossref] [PubMed]
- Lau IW, Liu D, Xu L, Fan Z, Sun Z. Clinical value of patient-specific three-dimensional printing of congenital heart disease: Quantitative and qualitative Assessments. PLoS One 2018;13:e0194333. [Crossref] [PubMed]
- Valverde I, Gomez-Ciriza G, Hussain T, Suarez-Mejias C, Velasco-Forte MN, Byrne N, Ordoñez A, Gonzalez-Calle A, Anderson D, Hazekamp MG, Roest AAW, Rivas-Gonzalez J, Uribe S, El-Rassi I, Simpson J, Miller O, Ruiz E, Zabala I, Mendez A, Manso B, Gallego P, Prada F, Cantinotti M, Ait-Ali L, Merino C, Parry A, Poirier N, Greil G, Razavi R, Gomez-Cia T, Hosseinpour AR. Three dimensional printed models for surgical planning of complex congenital heart defects: an international multicenter study. Eur J Cardiothorac Surg 2017;52:1139-48. [Crossref] [PubMed]
- Lau I, Sun Z. Three-dimensional printing in congenital heart disease: A systematic review. J Med Radiat Sci 2018;65:226-36. [Crossref] [PubMed]
- Lau I, Wong YH, Yeong CH, Abdul Aziz YF, Ms Sari NA, Hashim SA, Sun Z. Quantitative and qualitative comparison of low- and high-cost 3D-printed heart models. Quant Imaging Med Surg 2019;9:107-14. [Crossref] [PubMed]
- Sun Z. Personalized three-dimensional printed coronary artery models for accurate assessment of coronary stenosis using high resolution imaging. Australasian Med J 2019;12:105-9.
- Sun Z, Lau I, Wong YH, Yeong CH. Personalised three-dimensional printed models in congenital heart disease. J Clin Med 2019;8:522. [Crossref] [PubMed]
- Sun Z, Ng CK, Squelch A. Synchrotron radiation computed tomography assessment of calcified plaques and coronary stenosis with different slice thicknesses and beam energies on 3D printed coronary models. Quant Imaging Med Surg 2019;9:6-22. [Crossref] [PubMed]
- Sun Z, Dimpudus FJ, Nugroho J, Adipranoto JD. CT virtual intravascular endoscopy assessment of coronary artery plaques: a preliminary study. Eur J Radiol 2010;75:e112-9. [Crossref] [PubMed]
- Xu L, Sun Z. Virtual intravascular endoscopy visualization of calcified coronary plaques: a novel approach of identifying plaque features for more accurate assessment of coronary lumen stenosis. Medicine 2015;94:e805. [Crossref] [PubMed]
- Sun Z, Dosari SA, Ng C, al-Muntashari A, Almaliky S. Multislice CT virtual intravascular endoscopy for assessing pulmonary embolisms: a pictorial review. Korean J Radiol 2010;11:222-30. [Crossref] [PubMed]
- Sun Z, Xu L. CT virtual intravascular endoscopy in the visualization of coronary plaques: A pictorial essay. Curr Med Imaging Rev 2017;13:154-61. [Crossref]
- Abdullah KA, McEntee MF, Reed W, Kench PL. Development of an organ-specific insert phantom generated using a 3D printer for investigations of cardiac computed tomography protocols. J Med Radiat Sci 2018;65:175-83. [Crossref] [PubMed]
- Aldosari S, Jansen S, Sun Z. Patient-specific 3D printed pulmonary artery model with simulation of peripheral pulmonary embolism for developing optimal computed tomography pulmonary angiography protocols. Quant Imaging Med Surg 2019;9:75-85. [Crossref] [PubMed]
- Aldosari S, Jansen S, Sun Z. Optimization of computed tomography pulmonary angiography protocols using 3D printing model with simulation of pulmonary embolism. Quant Imaging Med Surg 2019;9:53-62. [Crossref] [PubMed]