Atherosclerosis in intracranial or extracranial vessels in diabetic patients and the association with stroke subtype
Introduction
Diabetes mellitus (DM) is an accepted independent risk factor for ischemic stroke. There is limited and conflicting data on the impact of DM on large and small artery disease in the brain. Some reports claim that DM increases the risk of large artery disease (LAD) but not of small artery disease (SAD) in the brain (1,2), while others indicate that lacunar infarction is more frequent in patients with DM than in patients without DM (3). The different results might partly be because some studies used data from Doppler ultrasound, which cannot screen atherosclerosis in the intracranial and extracranial vessel comprehensively, and partly because of some patients with symptomatic intracranial and extracranial stenosis presented with lacunar stroke. It remains unclear which morphologic characteristics of atherosclerosis (i.e., severity, location, tandem lesion, or collateral circulation) are most closely associated with ischemic stroke subtype.
Metabolic abnormalities caused by DM induce vascular dysfunction that predisposes this patient population to atherosclerosis. We hypothesized that atherosclerosis in diabetes patients would cause more tandem lesions in both intracranial and extracranial vessels. With the Contrast-Enhanced Timing Robust Angiography (CENTRA) k-space sampling and sensitivity encoding (SENSE) techniques, time-of-flight MR angiography (TOF-MRA) accelerates image acquisition and reduces artery overlay (4), increasing the sensitivity and specificity of MRA for the detection of cerebral arterial stenosis. A meta-analysis has shown that contrast-enhanced MR angiography (CE-MRA) is the most accurate for 70–99% symptomatic stenosis (5). With multimodal MRI (including TOF-MRA for intracranial artery, CE-MRA for the extracranial artery, and brain MRI) at a one-stop examination, it is possible to assess both the location of artery disease and the ischemic stroke subtype. This study aimed to comprehensively assess atherosclerosis of intracranial and extracranial vessels in diabetic patients and its association with ischemic stroke subtype by using multimodal MRI. The findings of this study could be useful during imaging-based therapeutic decision making and for individualized secondary prevention.
Methods
Study population
This study complied with the principles of the Declaration of Helsinki [1964], and the approval was obtained from the local institutional review board. For this retrospective study, a consecutive of 1,428 patients with neurological symptoms clinically suspected to be due to stenosis of the carotid or intracranial arteries were identified from the database at our neurology clinic between 2012 and 2017. According to inclusion criteria, we selected 128 DM patients with neurological symptoms clinically suspected to be due to stenosis of the carotid or intracranial arteries, meanwhile another set of 195 nondiabetic patients with neurological symptoms were selected to form a control group (Figure 1). The “neurological symptoms” included transient symptoms (i.e., transient ischemic attack or amaurosis fugax dizziness) and prior stroke (i.e., any ischemic event with neurologic symptoms, including lacunar syndromes). Patients were excluded if they (I) had not undergone vascular imaging workup; (II) had presented with emboligenic cardiac diseases; (III) had intracranial hemorrhage or vascular malformations; (IV) had miscellaneous etiologies such as arterial dissection, vasculitis, or underlying active lupus disease; or (V) had recent history of conditions associated with hemodynamic disturbance, e.g., severe bleeding, severe anemia, dehydration, or exhaustion.
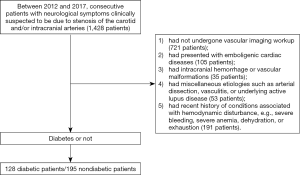
Data on baseline demographics (age, gender, body mass index), laboratory findings [HbA1c, low-density lipoprotein (LDL), high-density lipoprotein (HDL), triglycerides, serum creatinine], and medical history (diabetes duration, history of hypertension) were collected from the hospital records.
Imaging protocol
All patients underwent CT within 3 h of stroke onset; MRI was also done if necessary. Subsequently, MRA was performed to identify the location and severity of intracranial and extracranial artery stenosis. If significant stenosis was identified, digital subtraction angiography (DSA) was performed (within 7 days of MRA) to evaluate the severity of stenosis and the collateral flow.
MRI and DSA examination
MRI protocol consists of T1-and T2-weighted imaging, diffusion-weighted imaging (DWI) (with b values of 0 and 1,000 s/mm2), fluid-attenuated inversion recovery (FLAIR) imaging, TOF-MRA, and CE-MRA. MRI examinations were performed using a 3T MR imaging unit (Achieva; Philips Medical Systems, Best, The Netherlands) equipped with either a 16-channel phased-array head coil or 8-channel phased-array carotid coil. Three-dimensional TOF-MRA (3D-TOF-MRA) of the brain was obtained using a 3D-T1-Fast Field Echo (FFE) sequence with repetition time (TR)/echo time (TE), 30 ms/3.2 ms; flip angle, 20°; field-of-view (FOV), 250×190×108; four slabs (180 slices); slice thickness, 0.8 mm; matrix, 732×1,024; and reconstructed pixel, 0.24×0.24×1.2 mm3. We used CENTRA k-space SENSE to accelerate image acquisition. SENSE factor was 2, and the acquisition time was 8 min 56 s.
CE-MRA was performed immediately after 3D-TOF-MRA and covered the region from the aortic arch to the level of the central skull base in the coronal plane. After a preliminary mask image was acquired, 2 mL of gadolinium-DTPA (Gd-DTPA; Magnevist; Schering AG, Berlin, Germany) was administered to measure transit time from the arm vein to the carotid bifurcation. The mean delay time was 13 s (range, 11–19 s). A standard 20 mL volume of Gd-DTPA was then injected at a rate of 2.5 mL/s by using an MR-compatible power injector (Spectris; Medrad, Indianola, PA, USA). This was followed by a 20 mL flush of 0.9% saline injected at the same rate. A SURVEY-PCA (TR/TE 4.3 ms/1.27 ms; FOV 450 mm × 450 mm) was used for image acquisition, with a slice thickness of 150 nm and a voxel size of 1.76 mm × 1.8 mm. The dynamic scan time was 0.54 s, and overall imaging time was 1 min and 22.4 s.
After the acquisition, both the 3D-TOF-MRA and CE-MRA image data sets were transferred to a work station (EWS; Philips Medical), where 3D image reconstruction was performed with volume rendering (VR) and maximum intensity projection (MIP) using a specialized software package (Volume Inspection; Philips Medical). To reduce artery overlay and effectively identify extracranial and intracranial artery disease, the single-artery-highlighting method was applied as previously described (4).
Conventional DSA was performed on a DSA unit (Artiz, Siemens Healthcare, Germany) with a 1,024×1,024 matrix and a 170–200 mm FOV. The extracranial and intracranial arteries were displayed in at least two projections (anteroposterior and lateral) by automated injection of 8–10 mL of iodixanol (320 mg of iodine/mL) for carotid and intracranial arteries (flow rate, 4–5 mL/s) and 7 mL for the vertebral artery (flow rate, 2–3 mL/s), respectively; rotational angiography was performed in some cases. Three-dimensional images were reconstructed by VR and MIP on a workstation (SyngoXWP VA70B; Siemens Healthcare, Germany).
Ischemic stroke subtype and vascular etiologies
Using the MRI, angiography, and clinical data, stroke patterns were categorized according to the underlying cause (large artery and lacunar) as previously reported (6,7). Briefly, lacunar stroke can present clinically with one of six typical lacunar syndromes. It occurs in association with either a striatocapsular infarct or a subcortical infarct ≤15 mm or even in the absence of MRI evidence of an infarct. Large artery strokes were subdivided according to the underlying mechanism into four groups: (I) artery-to-artery embolism (where the infarct is distal to the stenotic vessel in the territory of the relevant artery); (II) local branch occlusion (where the infarct is localized to an area adjacent to the stenosed vessel); (III) in situ thrombo-occlusion (where the infarct extensively involves most or all of the territory supplied by the stenosed artery); (IV) hemodynamic impairment (where the infarct is located in border zone areas).
Definite association between the ischemic stroke lesion and the stenosis was diagnosed when the neurological signs were consistent with an acute or subacute infarct in an area of the brain supplied by the stenotic artery. Concomitant asymptomatic atherosclerosis, i.e., an extracranial or intracranial artery atherosclerotic disease unrelated to the current infarction, was also assessed.
Image analysis
Two radiologists (LM Wei and JG Zhao), blinded to all clinical information, evaluated the MR images of all patients in a consensus reading. MRI of the brain was assessed for the presence of acute (hyperintense on DWI and hypointense on apparent diffusion coefficient map) or subacute (hyperintense on T2-weighted and FLAIR images) large artery infarcts or lacunar infarcts.
The location of atherosclerosis was classified as (I) intracranial artery, i.e., including the distal internal carotid artery (dICA) (the cavernous and petrous segments), middle cerebral artery (MCA), anterior cerebral artery (ACA), posterior cerebral artery (PCA), basilar artery (BA), and distal vertebral artery (dVA) (the intradural V4 segment); or (II) extracranial artery, i.e., including the subclavian artery, common carotid artery (CCA) (from the bifurcation down to the origin from the aortic arch or innominate artery), the proximal internal carotid artery (pICA), and the proximal vertebral artery (pVA) (including the ostium and V2–3 segments).
Extracranial and intracranial steno-occlusive disease was graded as grade 1 (low-grade stenosis; <50%); grade 2 (high-grade stenosis; 50–99%); or grade 3 (complete occlusion; 100%). Any stenosis >50% was regarded as clinically significant stenosis (grade 2 & 3). Concurrent stenosis was defined as the presence of any degree of stenosis in both extracranial and intracranial vessels; this included lesions in the same vascular territories (tandem lesions) and lesions in different vascular territories (non-tandem lesions).
A battery of angiographic scales was used to evaluate severe stenosis/occlusive lesion, antegrade flow, downstream territorial perfusion, and collateral site and grade. The collateral site could be communication between the extracranial and intracranial circulation (type 1), blood flow redistribution through the circle of Willis (type 2), leptomeningeal anastomoses (type 3), or mixed (type 4). Collateral circulation grade assessment was as described in a previous study (8).
Statistical analysis
Differences between categorical variables were evaluated using the Pearson chi-square test, and differences between continuous variables with one-way ANOVA. Multivariate logistic regression analysis was performed to determine the risk factors associated with stroke subtype. Statistical analysis was performed using SPSS for Windows, version 13.0 (SPSS Inc., Chicago, IL, USA). P≤0.05 was considered statistically significant.
Results
General characteristics and location of atherosclerosis
Table 1 summarizes the characteristics of the patients in the two groups. The prevalence of accepted risk factors of atherosclerosis such as age, hypertension, and dyslipidemia were comparable in the two groups. Atherosclerosis was present in 93.8% diabetic patients vs. 78.5% nondiabetic patients (P=0.002). While atherosclerosis mostly affected extracranial arteries in diabetic patients, it showed no predilection for any particular site in nondiabetic patients (Table 1). Clinically significant stenosis was present in 35.9% diabetic patients vs. 48.7% in nondiabetic patients (Table 2).
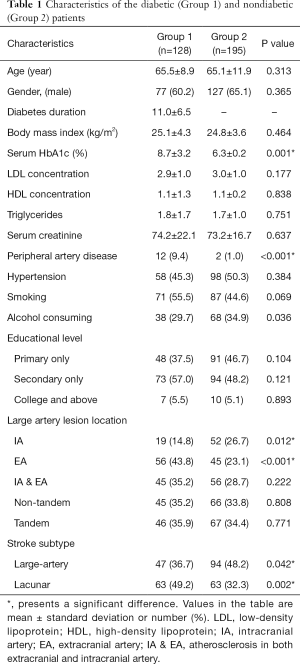
Full table
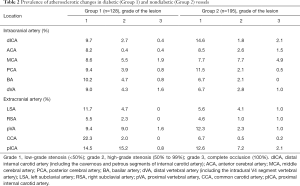
Full table
With DSA as the control, the sensitivity, specificity, and accuracy of TOF-MRA for the detection of clinically significant stenosis were 100%, 99.4%, and 99.5%, respectively. Sensitivity, specificity, and accuracy of CE-MRA were 100%, 99.5%, and 99.6%, respectively.
Differences between diabetic and nondiabetic patients
Atherosclerosis in extracranial vessels was significantly higher in diabetic patients than in nondiabetic patients (43.8% vs. 23.1%; P<0.001), whereas atherosclerosis in intracranial vessels was significantly lower in diabetic patients (14.8% vs. 26.7%; P=0.012; Table 1). Extracranial atherosclerosis tended to be more severe in diabetic patients, and high-grade stenoses in pICA and pVA were significantly more common in this group (P<0.05; Table 2).
Diabetic patients were significantly more likely to have lacunar infarction than nondiabetic patients (49.2% vs. 32.3%; P=0.002) and significantly less likely to have large artery infarct (36.7% vs. 48.2%; P=0.042). In multivariate regression analysis, presence of DM (OR, 2.03; 95% CI, 1.96–4.30; P=0.006) and age >65 years (OR, 2.55; 95% CI, 1.24–5.22; P=0.011) were independent risk factors for lacunar infarct.
Association of symptomatic stenosis or occlusion with ischemic stroke subtype
Among the patients with symptomatic stenosis or occlusion, type and grade of collateral circulation were not significantly different between diabetic and nondiabetic patients (P>0.05; Table 3). In multivariate logistic regression analysis, after adjustment for gender, age, and group, the factors significantly associated with risk of large artery stroke were atherosclerosis in intracranial artery (OR, 1.763; 95% CI, 1.037–2.995; P=0.036), collateral type (OR, 0.350; 95% CI, 0.127–0.967; P=0.043), and collateral scale (OR, 2.254; 95% CI, 1.089–4.664; P=0.029) (Table 4). The risk of large artery stroke was significantly higher in nondiabetic patients (P=0.042). However, diabetic patients with symptomatic stenosis or occlusion, and good collateral circulation had a significantly higher risk of having lacunar infarction [47.8% (22/46) vs. 30.5% (29/95); P=0.045].
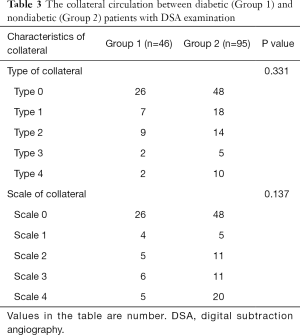
Full table
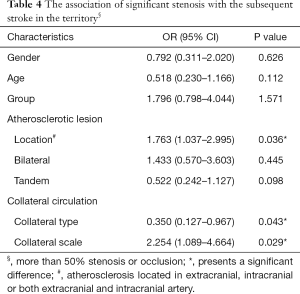
Full table
Discussion
This study was performed to determine the prevalence of atherosclerosis in intracranial and extracranial vessels of diabetic and nondiabetic patients with neurological symptoms and to examine the association of atherosclerosis with stroke subtype. The main findings were: (I) DM was associated with increased prevalence of atherosclerosis in extracranial vessels and also with increased severity of atherosclerosis in extracranial vessels, especially in pICA and pVA); (II) lacunar infarct was more frequent in diabetic patients than in nondiabetic patients; and (III) some patients with symptomatic stenosis might present with lacunar stroke, depending upon the location of the stenosis and the collateral circulation status.
Intracranial atherosclerosis is common among Asians. Our study showed that DM might increase the risk of atherosclerosis in extracranial vessels. We found symptomatic stenosis in the pICA and pVA to be more common in diabetic patients, which is consistent with one earlier report (9) but not with several others (1,10,11). The differences between the studies might be due to the inclusion criteria used for patient selection. We enrolled diabetic patients with neurologic symptoms suspicious of stenosis of the carotid or intracranial arteries.
In contrast, Lei et al. included acute (<7 days after onset) ischemic stroke or transient ischemic attack patients, for intracranial atherosclerosis is one of the most common causes of ischemic stroke and transient ischaemic attack (TIA) and vice versa. In our study, the predilection for symptomatic stenosis in pICA and pVA may be attributable to impairment of endothelial function in diabetic patients. It must be noted that all the studies cited here were hospital-based studies. The exact prevalence can only be established with further population-based studies.
Previous studies have reported different stroke subtypes in diabetic patients. One study showed that lacunar infarcts were more frequent in diabetic patients (3). Another reported that both atherothrombotic stroke and lacunar infarction are more common in diabetic patients than in nondiabetic individuals (10). Kim et al. demonstrated that DM increased the risk of LAD in the brain but not of SAD (1). Our results were consistent with some of these previous findings. The heterogeneous results regarding the prevalence of lacunar infarct and LAD in diabetic patients might be due to differences between studies in sample sizes, the definition of “lacunar infarct”, or the imaging modalities used. “Lacunar infarct” has classically been believed to be due to SAD. However, lacunar infarct may also result from LAD or cardioembolic disease. In the previous series, patients with symptoms of one of the six characteristic lacunar syndromes and with CT or MRI features of lacunar infarct were classified as having lacunar infarct (2,3), without paying attention to the possibility of a LAD (especially ipsilateral artery). In clinical practice, however, there are no differences in clinical presentation between patients with lacunar infarct due to SAD and LAD. The risk factors are very similar in both groups (12); however, stroke recurrence seems to be more frequent in lacunar infarct associated with LAD (13). In our study, we used a multimodal MRI to assess LAD and infarct in the brain at a one-stop examination. We found that 47.8% (22/46) of diabetic patients and 30.5% (29/95) of nondiabetic patients with symptomatic stenosis or occlusion had a lacunar infarction. Recent studies show that diabetic patients with lacunar stroke have a distinctive clinical profile that includes a 2-fold higher prevalence of systemic and intracranial atherosclerosis, predilection for involvement of the posterior circulation, and poor prognosis (14). Taken together, these findings suggest that the prevalence of lacunar infarct caused by LAD was underestimated.
There are four main hypothesized mechanisms of stroke related to atherosclerosis in extracranial and intracranial large arteries: hypoperfusion, artery-to-artery embolism, plaque extension over the ostia of a small penetrating artery (also known as branch atheromatous disease), and in situ thrombo-occlusion (6,15). However, infarct caused by LAD may be lacunar or non-lacunar. Some patients with symptomatic stenosis in extracranial and intracranial large arteries presented with lacunar stroke. This was partly due to the degree of stenosis. How the other characteristics of atherosclerosis (i.e., location, presence of a tandem lesion, bilateral lesion) and the type and scale of collateral circulation affect stroke subtype are still uncertain. Multivariate logistic regression analyses in our study showed that patients with symptomatic stenosis or occlusion in an extracranial artery and with abundant collateral circulation appear to be at increased risk for lacunar stroke.
In contrast, patients with atherosclerosis in an intracranial artery and a poor collateral circulation had a significantly higher risk for large artery stroke. Our result supports the concept that collateral circulation status may influence infarct size and clinical severity (8). A previous study has also demonstrated that recurrent strokes were typically nonlacunar in patients with prior history of symptomatic intracranial stenosis and lacunar stroke (7). Therefore, patients with lacunar strokes should not be assumed to have isolated SAD; LAD and other potential causes should be ruled out.
This study has several limitations. Firstly, our study did not access the SAD, and the diagnosis of lacunar strokes had relied on lacunar syndromes or MR imaging findings. This poses the question of whether the infarction is caused by the stenosis or a result of coexistent penetrating SAD with the intracranial stenosis. Further study is warranted to identify the exact mechanism of lacunar stroke. Secondly, the association of stratified duration of diabetes with atherosclerosis in intracranial and extracranial vessels and ischemic stroke subtype is not analyzed in this study, which needs further research. Thirdly, in this study, 721 patients with no vascular image for further analysis because of various reasons and it may introduce exclusion bias and increase the uncertainty for the results, which required a further study that has large samples recruited from multi-centers. Lastly, this was a hospital-based study with a relatively small number of patients, and the sample might not be representative of all diabetic patients. Population-based studies with the big sample are needed to identify the exact prevalence.
Conclusions
The prevalence and location of atherosclerosis differ between diabetic and nondiabetic patients. DM increases the severity of atherosclerosis in extracranial vessels and seems to be closely associated with lacunar infarction. For the prevention and treatment of stroke in diabetic patients, these differences should be taken into account. Population-based studies are needed to clarify the mechanisms of stroke and strategies for prevention in diabetic patients.
Acknowledgments
Funding: This study received funding by the National Natural Science Foundation of China (Nos. 81000652; 81370041, 81271683), Science Foundation of Shanghai Jiaotong University Affiliated Sixth People’s Hospital (No. ynlc201611; 201818), the Cross Project of Medicine and Engineering from Shanghai Jiao Tong University (Grant YG2015MS21) and Shanghai key discipline of medical imaging (No: 2017ZZ02005).
Footnote
Conflicts of Interest: The authors have no conflicts of interest to declare.
Ethical Statement: This study complied with the principles of the Declaration of Helsinki [1964], and the approval was obtained from the local institutional review board.
References
- Kim BJ, Lee SH, Kang BS, Yoon BW, Roh JK. Diabetes increases large artery diseases, but not small artery diseases in the brain. J Neurol 2008;255:1176-81. [Crossref] [PubMed]
- Karapanayiotides T, Piechowski-Jozwiak B, van Melle G, Bogousslavsky J, Devuyst G. Stroke patterns, etiology, and prognosis in patients with diabetes mellitus. Neurology 2004;62:1558-62. [Crossref] [PubMed]
- Megherbi SE, Milan C, Minier D, Couvreur G, Osseby GV, Tilling K, Di Carlo A, Inzitari D, Wolfe CD, Moreau T, Giroud M. European BIOMED Study of Stroke Care Group. Association between diabetes and stroke subtype on survival and functional outcome 3 months after stroke: data from the European BIOMED Stroke Project. Stroke 2003;34:688-94. [Crossref] [PubMed]
- Wu Q, Li MH. A comparison of 4D time-resolved MRA with keyhole and 3D time-of-flight MRA at 3.0 T for the evaluation of cerebral aneurysms. BMC Neurol 2012;12:50. [Crossref] [PubMed]
- Chappell FM, Wardlaw JM, Young GR, Gillard JH, Roditi GH, Yip B, Pell JP, Rothwell PM, Brown MM, Gough MJ, Randall MS. Carotid artery stenosis: accuracy of noninvasive tests--individual patient data meta-analysis. Radiology 2009;251:493-502. [Crossref] [PubMed]
- Kim JS, Nah HW, Park SM, Kim SK, Cho KH, Lee J, Lee YS, Kim J, Ha SW, Kim EG, Kim DE, Kang DW, Kwon SU, Yu KH, Lee BC. Risk factors and stroke mechanisms in atherosclerotic stroke: intracranial compared with extracranial and anterior compared with posterior circulation disease. Stroke 2012;43:3313-8. [Crossref] [PubMed]
- Inzitari D, Eliasziw M, Gates P, Sharpe BL, Chan RK, Meldrum HE, Barnett HJ. The causes and risk of stroke in patients with asymptomatic internal-carotid-artery stenosis. North American Symptomatic Carotid Endarterectomy Trial Collaborators. N Engl J Med 2000;342:1693-700. [Crossref] [PubMed]
- Liebeskind DS, Cotsonis GA, Saver JL, Lynn MJ, Turan TN, Cloft HJ, Chimowitz MI. Warfarin-Aspirin Symptomatic Intracranial Disease (WASID) Investigators. Collaterals dramatically alter stroke risk in intracranial atherosclerosis. Ann Neurol 2011;69:963-74. [Crossref] [PubMed]
- Lukovits TG, Mazzone TM, Gorelick TM. Diabetes mellitus and cerebrovascular disease. Neuroepidemiology 1999;18:1-14. [Crossref] [PubMed]
- Arboix A, Rivas A, García-Eroles L, de Marcos L, Massons J, Oliveres M. Cerebral infarction in diabetes: clinical pattern, stroke subtypes, and predictors of in-hospital mortality. BMC Neurol 2005;5:9. [Crossref] [PubMed]
- Lei C, Wu B, Liu M, Chen Y. Risk factors and clinical outcomes associated with intracranial and extracranial atherosclerotic stenosis acute ischemic stroke. J Stroke Cerebrovasc Dis 2014;23:1112-7. [Crossref] [PubMed]
- Khan A, Kasner SE, Lynn MJ, Chimowitz MI. Warfarin Aspirin Symptomatic Intracranial Disease (WASID) Trial Investigators. Risk factors and outcome of patients with symptomatic intracranial stenosis presenting with lacunar stroke. Stroke 2012;43:1230-3. [Crossref] [PubMed]
- Roquer J, Campello AR, Gomis M. Association of lacunar infarcts with small artery and large artery disease: a comparative study. Acta Neurol Scand 2004;110:350-4. [Crossref] [PubMed]
- Palacio S, McClure LA, Benavente OR, Bazan C 3rd, Pergola P, Hart RG. Lacunar strokes in patients with diabetes mellitus: risk factors, infarct location, and prognosis: the secondary prevention of small subcortical strokes study. Stroke 2014;45:2689-94. [Crossref] [PubMed]
- Holmstedt CA, Turan TN, Chimowitz MI. Atherosclerotic intracranial arterial stenosis: risk factors, diagnosis, and treatment. Lancet Neurol 2013;12:1106-14. [Crossref] [PubMed]