Advanced endoscopic methods in gastrointestinal diseases: a systematic review
Introduction
Gastrointestinal diseases seriously affect human health, and gastrointestinal tumors are characterized by high morbidity and mortality. Cancer statistics, 2018 shows that the incidence of cancer in the digestive tract is the highest in the United States (1).
As the main method of detecting gastrointestinal diseases, traditional endoscopy is of great significance for improving the quality of life of humans. In recent years, endoscopic techniques have made numerous advancements, providing a powerful guarantee for the accurate diagnosis of gastrointestinal diseases. This article reviews the available advanced endoscopic imaging modalities, as well as relevant evidence that may improve the diagnosis of gastrointestinal diseases.
White light endoscopy (WLE)
As the first method used in endoscopic examination, WLE remains the fundamental step for the detection and characterization of gastrointestinal diseases in clinical practice. Considering the poor correlation between WLE findings and histopathological analysis (2), WLE cannot reliably diagnose Helicobacter pylori gastritis (3,4). However, some studies have shown that WLE remains promising for the detection of preneoplastic lesions. Real-time high-definition WLE (HD-WLE) has an accuracy rate of 88% for the identification of intestinal metaplasia, with a sensitivity of 74.6% and specificity of 94.2% (5). WLE is still an effective method to detect gastrointestinal diseases. However, in some previously published studies, a poor correlation between macroscopic findings and histopathological diagnosis was found (2). Further, for the same images obtained by WLE, endoscopists with different abilities will make different diagnoses (6). In addition, for early lesions of digestive tract diseases, because of the lack of clear endoscopic viewing, WLE is prone to misdiagnosis. Thus, the diagnostic accuracy mostly depends on the ability of the endoscopist (7).
Chromoendoscopy (CE)
CE refers to the topical application of biocompatible dye agents at the time of endoscopy in an effort to enhance tissue characterization and differentiation, and thus improve the detection rate of gastrointestinal diseases (8). Common stains include Lugol’s solution, methylene blue, and toluidine blue. Because of the use of dyes, the pathological tissue and normal tissue are distinguished based on their appearance. CE is considered safely at the dye concentration used.
Currently, CE has been widely used for the diagnosis of esophageal squamous neoplasia (9), Barrett’s esophagus (10), gastric neoplasia (7), colorectal neoplasia (11), and other diseases (8). CE with Lugol’s solution can significantly increase the image contrast between normal and pathological tissues to determine the lesion areas (Figure 1) (12). Zhao et al. showed that the detection rates for early gastric cancer (P<0.01) and precancerous lesions (P<0.01) were higher by CE than by standard WLE (13). Compared with conventional endoscopy, Brown et al. showed that CE had a higher detection rate for colorectal polyps (11).
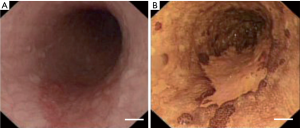
The use of dyes can enhance image contrast, while introducing other issues. After staining the tissue with Lugol’s solution, early esophageal cancer can be graded according to the lesion edge clearance, size of lesion range, depth of lesion staining, degree of swelling of lesion site, and changes in esophageal mucosa (14). With the characteristics of fast staining and high accuracy, CE is currently the main method for diagnosing early esophageal cancer, but it is forbidden for patients with allergies to iodine or hyperthyroidism (9). Intestinal metaplasia cells and necrotic tissue can be stained with methylene blue, whereas normal epithelial tissue remains unstained; thus, methylene blue is mostly used in the diagnosis of Barrett's esophagus and early esophageal adenocarcinoma. CE with methylene blue staining has improved the identification of gastric lesions (7,15). However, this method has a long dyeing time and high technical requirements, resulting in few clinical applications. Toluidine blue has been used for the detection of premalignant and malignant lesions because of its affinity for nuclear matter. Using this stain, the premalignant and malignant cells are stained blue, whereas normal cells are unstained or not significantly stained. It has a high sensitivity and specificity in the diagnosis of Barrett’s esophagus and adenocarcinoma with a characteristic of staining the columnar epithelium(16). However, this method still has limitations of a long staining time and high technical requirements, which limit its clinical application.
Computerized virtual chromoendoscopy (CVC)
Although CE is effective in many applications, this method still has some problems, such as the difficulty of completely and evenly coating the mucosal surface with the dye, additional cost of the equipment for dye spraying, and extra time required to perform the procedure (17). Computerized virtual CE can realize tissue staining by an optical method and enhance the contrast of the mucosa, thereby enabling the distinction between the normal mucosa and diseased tissue. CVC technology includes narrow band imaging (NBI), flexible spectral imaging color enhancement (FICE), and i-Scan.
NBI
NBI techniques use a special filter, electronically activated by a switch in the conventional endoscope, to act on the light source, leaving narrow bands with central wavelengths of 415 and 540 nm to enhance the detail of certain aspects on the surface of the mucosa. Because the peak light absorption of hemoglobin occurs at these wavelengths, the blood vessels appear very dark, which improves their visibility and the identification of other surface structures, facilitating the observation of tissues for diseases associated with microvasculature (17-19). Currently, NBI has been widely used in the detection of gastrointestinal diseases (18,20,21).
NBI can be used to perform preliminary histological diagnosis of early esophageal lesions and is useful for guiding targeted biopsy of lesions. Brownish epithelium and brownish dots found by NBI are used for the diagnosis of squamous mucosal high-grade neoplasia of the esophagus (22). NBI had a sensitivity of 97% for the detection of high-grade dysplasia or carcinoma in Barrett’s esophagus, with a specificity of 94% and an accuracy of 96% (23). NBI has established definitions of gastric mucosal tumors. Muto et al. (24) proposed a diagnostic algorithm for magnifying endoscopy of early gastric cancer based on the previously developed vessel plus surface NBI classification by Yao et al. (25) (Figure 2). Compared with WLE, NBI has a higher detection rate for intestinal metaplasia of local lesions in the stomach (26). NBI can diagnose diseases associated with micro-vessels. However, because of the limited resolution of NBI and its inability to detect cell-level lesions, it is prone to show false-negative results (25). In a previous study, endoscopic findings using magnifying NBI suggested that the tissue is normal, whereas the histopathological findings of biopsy specimens suggested a diagnosis of signet-ring cell carcinoma (25).
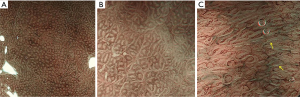
FICE
NBI is very useful for cancer detection (27-29), but it is difficult to get a clear image using NBI because of respiratory motion, heartbeat, and insufficient light intensity of the system, and an experienced endoscopic operator is required to diagnose diseases (30). FICE technology was invented by Miyake et al. (31-34). Contrary to NBI, this technology does not rely on filters. Spectral images of each wavelength are generated from conventional endoscopic images by reflectance spectrum estimation technique. Three spectral images are selected and allocated to the three channels of red, green, and blue to reconstruct the image (30). FICE can detect changes in early gastric cancer and confirm the diagnosis of cancer with low or half magnification (35). It also can enhance color contrast between normal and malignant lesions without magnification (36). In the study by Pittayanon et al., the accuracy rate of FICE for intestinal metaplasia was 85.5%, and the accuracy with the observation of a light blue crest was 95.2% and that with the observation of a long large crest was 96.8% (37).
i-Scan
Endoscopy should not only identify minute mucosal lesions but also accurately characterize the identified lesions during ongoing endoscopy (38). The i-Scan technique is developed based on the difference in the reflective properties of the normal and abnormal mucosa. After optical staining, the normal mucosa and the lesion can be distinguished with different colors. The i-Scan technology consists of three types of algorithms: surface enhancement (SE), contrast enhancement (CE), and tone enhancement (TE). SE and CE are suitable for the detection of gastrointestinal tumors at an early stage, and TE is suitable for detailed examination of lesions (39). It can provide detailed analysis based on blood vessels (i-Scan V), patterns (i-Scan P), and surface architecture (i-Scan SE) (38), as well as imaging modes for different tissues, enabling multi-channel and multi-color contrast (39).
A previous study shows that high-definition endoscopy combined with i-Scan and CE can ideally detect the micro-damage of the esophageal mucosa and guide targeted biopsy, which can pathologically confirm the diagnosis of non-erosive reflux disease (38). Qi et al. found that compared with magnification WLE, magnifying i-Scan can provide a better image quality for type 2–3 gastric mucosal pit pattern, and the accuracy of diagnosis for Helicobacter infection using i-Scan is 94%, with a sensitivity of 95.5% and a specificity of 93.5% (40).
Owing to the limited data on FICE and i-Scan for the diagnosis of precancerous lesions and neoplasia in the digestive tract, there is currently insufficient evidence to confirm their clinical availability in the detection of digestive tract tumors and precancerous lesions (41).
Although there are many endoscopic methods for the diagnosis of digestive tract mucosal diseases, only macroscopic structures such as blood vessels and polyps can be observed by endoscopy. Biopsy is still the gold standard for the diagnosis of gastrointestinal diseases. However, such operations and analysis take a longer time and affect the doctor to make diagnosis in time. It is desirable to acquire a direct histological image of the in vivo human gastrointestinal living mucosa without an actual biopsy. This method also means that digital images can be acquired without damaging the tissue, and such digital images are comparable to the images seen by conventional cytological methods (42). An endoscope with the ability of cell resolving is of great significance for "in vivo biopsy" to assist doctors in diagnosis.
Confocal laser endomicroscopy (CLE)
CLE is an endoscopic modality that obtains high magnification and resolution images of the mucosal layer of the gastrointestinal tract, presenting cellular morphology which is highly consistent with that presented by biopsy, making it a promising modality for early cancer detection. It is based on the principle of a confocal microscope, using low-power lasers to illuminate tissue, and then detecting tissue fluorescence through a pinhole for selective imaging with “optical sectioning” capability (43). Optical fibers and fiber bundles are not only used as light guiding media, an optical fiber, and a single fiber in the fiber bundle but also acts as a pinhole. Studies have shown that CLE has great potential in the diagnosis of early colon cancer lesions, Barrett’s esophagus, gastroesophageal reflux, and other diseases of the digestive tract (44).
Contrast agents
An exogenous contrast agent is usually used to achieve good contrast images using CLE. Currently, fluorescence contrast agents reported for human in vivo microscopic imaging include acriflavine hydrochloride and cresyl violet, which can be applied topically, and fluorescein sodium and indocyanine green (ICG) that can be administered intravenously (45-47). The most commonly used agents are acriflavine hydrochloride, fluorescein sodium, and ICG. Acridine hydrochloride can be used topically to stain nuclei and cytoplasm in the superficial epithelium of the mucosa. After a few seconds of spraying, it gets absorbed, allowing judging of the nuclear structure. However, it is not allowed for clinical examination owing to the risk for possible mutagenic activity. In contrast, fluorescein sodium is administered intravenously for imaging. It is found to bind extensively to the serum albumin, labeling the extracellular matrix and basement membrane of the surface epithelium, showing the crypt structure of the mucosa, epithelial cells, connective tissue of the lamina propria, vascular morphology, and red blood cells in it, and resulting in a strong contrast between the connective tissue and microvascular system (48). The absorption and fluorescence spectrum of both acriflavine hydrochloride and fluorescein sodium are in the visible region, whereas those of ICG are in the near infrared region. Combined with fluorescence detection, lasers with a wavelength of around 780 nm are commonly used. At this wavelength, it is still possible to detect the fluorescence of ICG by filtering out the scattered light from the excitation beam (49). After intravenous administration, ICG tightly binds to plasma proteins, albumin, or alpha-lipoprotein, and is eliminated directly from the liver (50,51). Fluorescence contrast agents approved for in vivo optical imaging in humans by the United States Food and Drug Administration are fluorescein sodium and ICG (52). Although other contrast agents are not clinically available, they can still be used in basic research in biology.
CLE based on distal scanning
CLE has a magnification of 1,000× and can observe the mucosa at a cellular level. CLE instruments are generally divided into two categories based on distal or proximal scanning and the type of light guide. The distally scanned endomicroscope performs beam scanning at the distal or tissue end, using one or two optical fibers for illumination and signal collection. One endomicroscope that uses an optical fiber for illumination and signal collection is the single-axis confocal laser endomicroscope developed by Optiscan Pty. Ltd (53). It is also known as endoscope-based CLE (eCLE), and a miniaturized confocal scanner has been integrated into the distal tip of these flexible endoscopes (54). Scanning of the distal end of an optical fiber is performed via cantilever mechanism, and a GRIN objective is used to focus the beam. eCLE has a high resolution of 0.7 µm with a field of view of 475 µm × 475 µm. eCLE has been widely used in the detection of digestive tract diseases. However, it is an integrated endoscope with a 12.8-mm outer diameter, which is too large to be compatible with biopsy channels of conventional endoscopes. Further, its imaging speed is slow, 0.8 fps or 1.6 fps, which greatly affects the diagnostic speed and limits its clinical application. A dual-axis CLE was proposed by Liu et al., separating the illumination and collection beams along different optical axes, using the region of overlap between the two beams to define the resolution (55) (Figure 3). A long working distance was made by low numerical aperture (NA) objectives so that the scanner can be placed on the tissue side or in the post-objective position. Further, a microelectromechanical system (MEMS) scanner performs beam scanning while maintaining the fixed region of intersection between the two focal beams below the tissue surface. With an outer diameter of 5.5 mm and a lateral resolution of 5 µm, images can be acquired with a field of view of 362 µm × 212 µm at 5 fps. Dual-axis CLE was first applied for in vivo imaging of human lower gastrointestinal tract in 2012, and the individual human colon crypts could be visualized in real time (47). However, it cannot realize a high level of cell recognition with its limited resolution. Nevertheless, for both eCLE and dual-axis CLE, the miniaturization of the distal scanning device remains a problem for compatibility with conventional endoscopes and other applications (48).
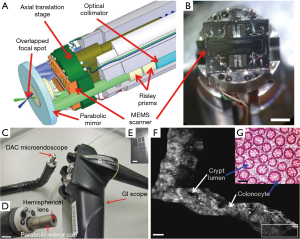
CLE based on proximal scanning
Probe-based confocal laser endomicroscopy (pCLE) for in vivo high-resolution imaging uses high-performance probes based on the proximal scanning mechanism (56). pCLE employs approximal scanning on the sides of the system using a fiber bundle instead of a single optical fiber for light guidance (56,57). The advantage of this technique is that the scanning system is not contained in the endoscopic probe, and therefore, the size of the scanning device is not limited; thus, commercial scanning systems can be used without miniaturization. However, the resolution of the pCLE is limited by the distance between neighboring fiber cores within the fiber bundle. To avoid this limitation, a high-NA miniature objective can be used at the distal end of the probe to focus the illuminated light passing through the fiber bundle into the tissue by collecting the light signal and coupling it into the same fiber to compensate for the resolution loss. Thus, various probes can be designed for different applications. In recent years, Mauna Kea technologies (MKT) has developed a variety of probes for different purposes, such as the S-series for surface imaging, M-series for high-resolution imaging, and Z-series for depth imaging (58). The pCLE can provide in vivo imaging at a speed of 12 fps.
Our group has developed a visible probe-based confocal laser endomicroscope in which a 488-nm laser source was used for illumination, which is similar to the endomicroscope developed by MKT. The outer diameter of the visible probe is 2.6 mm, enabling real-time imaging of the colon mucosa of C57BL/6 mice with a lateral resolution of 1.4 µm and a field of view of 300 µm × 300 µm at the depth of 150 µm from the surface (59,60). To achieve deep imaging, we have also developed a near-infrared endomicroscope. The optical structure of a near-infrared probe-based confocal laser endomicroscope is similar to that of a visible probe-based confocal laser endomicroscope (59,60). The excitation light was emitted from the laser (OBIS 785 LX, Coherent) and was expanded and then reflected by a dichroic mirror (DM, Di02-R785-25 × 36, Semrock). A two-dimensional scanner (CRS, Cambridge, 6215H, Cambridge) was used to scan the light, and then the beam was relayed into the back aperture of the objective lens (UMPLANFL N 20×/0.50, Olympus) and coupled with the fiber bundle. A miniature objective lens at the distal end of the fiber bundle focused the beam onto the tissue while collecting the fluorescent signal. The fluorescence returned along the same path, and passed through a dichroic mirror and a filter ((FF01-835/70-25, Semrock). Finally, the fluorescence was focused by a condenser through a pinhole and collected by a photomultiplier tube (PMT, R3896, Hamamatsu) (Figure 4). Compared with the outer diameter of a visible endomicroscope probe, that of a near-infrared endomicroscope probe remains 2.6 mm, yet it can achieve real-time deep imaging with a higher contrast, lateral resolution of 1.55 µm, and field of view of 330 µm × 330 µm at the depth of 300 µm from the surface. Deep tissue imaging using near-infrared probe-based confocal laser endomicroscopy has been demonstrated in mice for esophageal imaging at different depths. Deep tissue imaging of ulcerative colitis shows the ability of this system to diagnose diseases of deep-layer tissues (61). Table 1 compares the main parameters of our system with those of other CLE systems. In distally scanned endomicroscopy, the single-axis system has a higher resolution than the two-axis system, but the outer diameter of probe is lager in the former. Compared with the distally scanned CLE system, pCLE system, which uses a proximal scanning device, has a smaller outer diameter and can address different clinical applications as a variety of probes can be developed for it. For pCLE, the near-infrared system and not the visible system enables deep tissue imaging, and is useful for clinical disease diagnosis.
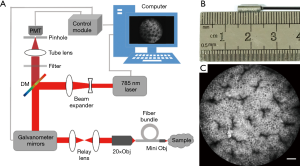
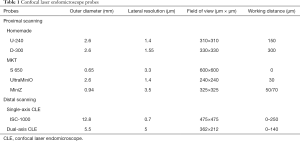
Full table
pCLE system is compatible with biopsy channels of traditional endoscopes for its small outer diameter, and enables real-time microscopic imaging of upper and lower gastrointestinal mucosa (62-65). Currently, commercial visible pCLE systems are widely used for the detection of diseases related to the esophagus (66,67), stomach (68,69) and intestine (70,71). Endoscopists can make a diagnosis by observing structural changes in these organs. Studies have shown that pCLE has a sensitivity of 100% and a specificity of 56% for the diagnosis of Barrett's esophagus (72). The overall accuracy of pCLE in the diagnosis of adenocarcinoma is 91.7%, suggesting that pCLE can provide an accurate diagnosis for superficial gastric tumors (68). For the diagnosis of gastrointestinal metaplasia, pCLE has a high sensitivity and accuracy of 90.9% and 88.0%, respectively (69). pCLE can be used for monitoring ulcerative colitis with a sensitivity, specificity, and accuracy of 65%, 82%, and 81%, respectively (70). Compared with standard histological analysis, the diagnostic accuracy of pCLE for the detection of dysplasia is higher with a sensitivity of 100%, specificity of 90%, and positive predictive value of 83% (71). pCLE has been used for characterizing abnormalities of the capillary and lymphatic vessels and epithelium in the small bowel. Significant changes can be observed before and after treatment, indicating that pCLE is useful for detecting small bowel diseases (73) (Figure 5).
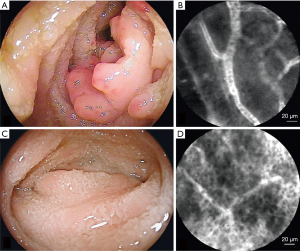
Moreover, visible pCLE is not only used for the clinical detection of gastrointestinal diseases but also for basic biological research, such as deep brain imaging, functional imaging, and observation of substance distribution. Vincent et al. achieved in situ deep-brain imaging at single-cell resolution, with the visualization of the striatum and ventral tegmental area, which is 4.5 mm below the surface of the mouse skull, and monitored neural activity in deep-brain regions for the first time, using a pCLE system with a probe with an outer diameter of 300 µm (74). Gore et al. visualized the fear-induced calcium signals in midbrain dopamine neurons with a probe identical to that used by Vincent et al. (75). pCLE is used for imaging the distribution of unmodified nanoparticles and IL-13p onto nanoparticles from blood vessels to tumor cells for analyzing the work of tumor-targeted delivery systems (76). Furthermore, pCLE is used to monitor the functional angiogenesis of endometriotic lesions by measuring the flow of the fluorescent dye in new microvessel (77), and assessing tumor vascular morphology and permeability (78).
pCLE can provide high-resolution images of the tissue with features similar to those of histological analysis. It can be used to guide biopsy to the target site, reduce unnecessary biopsies, increase the diagnostic yield, and reduce the incidence of surgical treatments. pCLE provides images and diagnostic information in real time, allowing endoscopists make prompt decisions. It has been shown to have high sensitivity and specificity compared with traditional endoscopies. pCLE provides an important tool for the in vivo investigation of many biological questions under a variety of physiological and pathological conditions.
Other endoscopic imaging techniques
Endoscopic optical coherence tomography (EOCT)
Optical coherence tomography (OCT) is a cross-sectional imaging method that uses a low-coherence near-infrared laser to identify optical inhomogeneities in biological tissues (79). EOCT is a method for endoscopic imaging based on OCT principle (80). To date, EOCT has been used for the imaging of the esophagus, stomach, and colon, among other organs (81). EOCT images reveal five distinct layers of normal human esophagus, namely epithelium, lamina propria, muscularis mucosa, submucosa, and muscularis propria (80,82). The ability of EOCT for imaging Barrett’s esophagus, high-grade dysplasia, and esophageal adenocarcinoma has been demonstrated (83). Although it can easily identify intestinal metaplasia within a normal esophagus, its ability to identify dysplasia within Barrett’s esophagus is relatively poor. EOCT has an overall accuracy rate of 92.7% for assessing tumor invasion in superficial esophageal squamous cell carcinomas (84). A study has shown that EOCT has a sensitivity of 90.0% and specificity of 83.3% in detecting the structure of the disrupted layer of the colon wall, indicative of transmural inflammation (85). OCT is a promising imaging technology that is easily accessible through the working channel of an endoscope. Standardized terminology and criteria for normal and neoplastic tissue are needed for OCT imaging. However, further studies on identification specificity and yield rate are required before it can be routinely used in clinical practice.
Photoacoustic endoscopy (PAE)
Photoacoustic imaging is a technique that acquires tissue images based on photoacoustic effect (86,87). Photoacoustic effect refers to changes in stress caused by the absorption of electromagnetic wave energy when the tissue is irradiated by a pulsed laser or an amplitude modulated laser, thereby emitting an acoustic wave, namely a photoacoustic signal. The photoacoustic signal is detected by the ultrasound transducer, and the tissue image is subsequently reconstructed (88-90). An ultrasound sensor with a high sensitivity, high frequency, and wide frequency band is beneficial for improving the performance in photoacoustic imaging (91,92). Based on the principle of photoacoustic imaging, a photoacoustic endoscope uses a miniaturized probe for both light delivery and acoustic detection to achieve endoscopic imaging (93-97). So far, PAE has been used for detection in the upper gastrointestinal tract of rabbits and lower gastrointestinal tract of rats (98,99). The clinical feasibility of using PAE has been demonstrated by an experiment distinguishing normal human colorectal tissue from colorectal cancer tissue in a previous study (100). With further enhancement of image contrast and accuracy of PAE, it has the potential to be used for the clinical detection of gastrointestinal diseases.
Future directions
With the development of the technology, endoscopy provides a powerful assurance for the detection of digestive tract diseases; in particular, CLE for cell resolution imaging is promising for providing virtual histology. However, pCLE systems with visible light still have some problems. Because of the fixed-focus probe, it can only observe the information on the fixed-deep mucosal surface. Accordingly, deep mucosal imaging using a near-infrared light source has also been reported (61,101). Moreover, two-photon imaging technology is also promising for endoscopic deep tissue imaging.
However, irrespective of the light source being visible light or near-infrared light, pCLE has the following limitations: (I) as the field of view is small, the area that can be observed at one time is limited and (II) it is difficult to detect small lesions and to assess the lesions that have completely developed. These limitations may be overcome in our next study, in which we include fiber bundles with a larger diameter and a custom-designed miniature objective with a large field of view. Also, professional training is necessary in pCLE for an accurate diagnosis. However, intelligent diagnosis may reduce the requirement of professional training of endoscopists, which is beneficial for the popularization of pCLE.
In addition to these endoscopic imaging techniques, other methods, such as autofluorescence endoscopy (102,103), Raman spectroscopy (104,105), endocytoscopy (106-108), and photodynamics (109) also have potential for clinical application in the diagnosis of gastrointestinal diseases. However, the specificity and accuracy of a single endoscopy for diagnosing gastrointestinal diseases are limited, and a combination of multiple endoscopic imaging methods can improve the diagnostic accuracy. Furthermore, the compact and cost-effective smartphone-based endoscope may be a powerful tool for detecting gastrointestinal diseases in resource-poor settings (110).
Conclusions
Early diagnosis of digestive tract diseases is beneficial for early treatment and better prognosis. Table 2 provides a summary of several endoscopic imaging methods discussed in this review. WLE is still the basic step in the detection of gastrointestinal diseases; the diseases can then be characterized by CE or CVC methods, and finally a biopsy is performed for accurate diagnosis. However, such procedures are complicated and insensitive to early lesions, and ultimately biopsy is still the “gold standard” for diagnosis, increasing patient suffering. pCLE enables early diagnosis because of the ability of cellular-level imaging. Further, it is promising to achieve “in vivo biopsy” using pCLE systems. In addition, EOCT and PAE are promising for detecting gastrointestinal diseases. The application of multiple endoscopic techniques is more helpful for making an accurate diagnosis of digestive tract diseases.
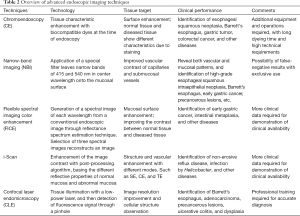
Full table
Acknowledgments
Funding: This study was supported by funding from the National Key Research and Development Program of China (2016YFA0201403, 2017YFC0110002 and 2017YFC0110003).
Footnote
Conflicts of Interest: The authors have no conflicts of interest to declare.
References
- Siegel RL, Miller KD, Jemal A. Cancer statistics, 2018. CA Cancer J Clin 2018;68:7-30. [Crossref] [PubMed]
- Atkins L, Benedict EB. Correlation of gross gastroscopic findings with gastroscopic biopsy in gastritis. N Engl J Med 1956;254:641-4. [Crossref] [PubMed]
- Kato T, Yagi N, Kamada T, Shimbo T, Watanabe H, Ida K. Diagnosis of Helicobacter pylori infection in gastric mucosa by endoscopic features: A multicenter prospective study. Dig Endosc 2013;25:508-18. [Crossref] [PubMed]
- Cho JH, Chang YW, Jang JY, Shim JJ, Lee CK, Dong SH, Kim HJ, Kim B-H, Lee TH, Cho JY. Close observation of gastric mucosal pattern by standard endoscopy can predict Helicobacter pylori infection status. J Gastroenterol Hepatol 2013;28:279-84. [Crossref] [PubMed]
- Panteris V, Nikolopoulou S, Lountou A, Triantafillidis JK. Diagnostic capabilities of high-definition white light endoscopy for the diagnosis of gastric intestinal metaplasia and correlation with histologic and clinical data. Eur J Gastroenterol Hepatol 2014;26:594-601. [PubMed]
- Laine L, Cohen H, Sloane R, Marin-Sorensen M, Weinstein WM. Interobserver agreement and predictive value of endoscopic findings for H. pylori and gastritis in normal volunteers. Gastrointest Endosc 1995;42:420-3. [Crossref] [PubMed]
- Dinis-Ribeiro M, da Costa-Pereira A, Lopes C, Lara-Santos L, Guilherme M, Moreira-Dias L, Lomba-Viana H, Ribeiro A, Santos C, Soares J, Mesquita N, Silva R, Lomba-Viana R. Magnification chromoendoscopy for the diagnosis of gastric intestinal metaplasia and dysplasia. Gastrointest Endosc 2003;57:498-504. [Crossref] [PubMed]
- Wong Kee Song LM, Adler DG, Chand B, Conway JD, Croffie JMB, DiSario JA, Mishkin DS, Shah RJ, Somogyi L, Tierney WM, Petersen BT. Chromoendoscopy. Gastrointest Endosc 2007;66:639-49. [Crossref] [PubMed]
- Curvers WL, Kiesslich R, Bergman JJ. Novel imaging modalities in the detection of oesophageal neoplasia. Best Pract Res Clin Gastroenterol 2008;22:687-720. [Crossref] [PubMed]
- Wang KK, Okoro N, Prasad G. WongKeeSong M, Buttar NS, Tian J. Endoscopic evaluation and advanced Iiaging of Barrett’s esophagus. Gastrointest Endosc Clin N Am 2011;21:39-51. [Crossref] [PubMed]
- Brown SR, Baraza W, Din S, Riley S. Chromoscopy versus conventional endoscopy for the detection of polyps in the colon and rectum. Cochrane Database Syst Rev 2016;4:CD006439. [PubMed]
- Trivedi PJ, Braden B. Indications, stains and techniques in chromoendoscopy. QJM 2013;106:117-31. [Crossref] [PubMed]
- Zhao Z, Yin Z, Wang S, Wang J, Bai B, Qiu Z, Zhao Q. Meta-analysis: The diagnostic efficacy of chromoendoscopy for early gastric cancer and premalignant gastric lesions. J Gastroenterol Hepatol 2016;31:1539-45. [Crossref] [PubMed]
- Mori M, Adachi Y, Matsushima T, Matsuda H, Kuwano H, Sugimachi K. Lugol staining pattern and histology of esophageal lesions. Am J Gastroenterol 1993;88:701-5. [PubMed]
- Areia M, Amaro P, Dinis-Ribeiro M, Moreira-Dias L, Romozinho JM, Gouveia H, Leitao MC. Estimation of the extent of gastric intestinal metaplasia by methylene blue chromoendoscopy. Eur J Gastroenterol Hepatol 2008;20:939-40. [PubMed]
- Chobanian SJ, Cattau EL, Winters C, Johnson DA, Van Ness MM, Miremadi A, Horwitz SL, Colcher H. In vivo staining with toluidine blue as an adjunct to the endoscopic detection of Barrett’s esophagus. Gastrointest Endosc 1987;33:99-101. [Crossref] [PubMed]
- Gono K, Obi T, Yamaguchi M, Oyama N, Machida H, Sano Y, Yoshida S, Hamamoto Y, Endo T. Appearance of enhanced tissue features in narrow-band endoscopic imaging. J Biomed Opt 2004;9:568-77. [Crossref] [PubMed]
- Singh R, Mei SCY, Sethi S. Advanced endoscopic imaging in Barrett's oesophagus: a review on current practice. World J Gastroenterol 2011;17:4271-6. [Crossref] [PubMed]
- Gono K. Narrow band imaging: technology basis and research and development history. Clin Endosc 2015;48:476-80. [Crossref] [PubMed]
- Tanaka S, Sano Y. Aim to unify the narrow band imaging (nbi) magnifying classification for colorectal tumors: current status in japan from a summary of the consensus symposium in the 79th annual meeting of the japan gastroenterological endoscopy society. Dig Endosc 2011;23:131-9. [Crossref] [PubMed]
- Efthymiou M, Taylor AC, Kamm MA. Cancer surveillance strategies in ulcerative colitis: the need for modernization. Inflamm Bowel Dis 2011;17:1800-13. [Crossref] [PubMed]
- Ishihara R, Inoue T, Uedo N, Yamamoto S, Kawada N, Tsujii Y, Kanzaki H, Hanafusa M, Hanaoka N, Takeuchi Y, Higashino K, Iishi H, Tatsuta M, Tomita Y, Ishiguro S. Significance of each narrow-band imaging finding in diagnosing squamous mucosal high-grade neoplasia of the esophagus. J Gastroenterol Hepatol 2010;25:1410-5. [Crossref] [PubMed]
- Curvers WL, Broek FJCVD, Reitsma JB, Dekker E, Bergman JJGHM. Systematic review of narrow-band imaging for the detection and differentiation of abnormalities in the esophagus and stomach (with video). Gastrointest Endosc 2009;69:307-17. [Crossref] [PubMed]
- Muto M, Yao K, Kaise M, Kato M, Uedo N, Yagi K, Tajiri H. Magnifying endoscopy simple diagnostic algorithm for early gastric cancer (MESDA-G). Dig Endosc 2016;28:379-93. [Crossref] [PubMed]
- Yao K, Doyama H, Gotoda T, Ishikawa H, Nagahama T, Yokoi C, Oda I, Machida H, Uchita K, Tabuchi M. Diagnostic performance and limitations of magnifying narrow-band imaging in screening endoscopy of early gastric cancer: a prospective multicenter feasibility study. Gastric Cancer 2014;17:669-79. [Crossref] [PubMed]
- Ang TL, Pittayanon R, Lau JYW, Rerknimitr R, Ho SH, Singh R, Kwek ABE, Ang DSW, Chiu PWY, Luk S, Goh KL, Ong JPL, Tan JY-L, Teo EK, Fock KM. A multicenter randomized comparison between high-definition white light endoscopy and narrow band imaging for detection of gastric lesions. Eur J Gastroenterol Hepatol 2015;27:1473-8. [Crossref] [PubMed]
- Tajiri H, Doi T, Endo H, Nishina T, Terao T, Hyodo I, Matsuda K, Yagi K. Routine endoscopy using a magnifying endoscope for gastric cancer diagnosis. Endoscopy 2002;34:772-7. [Crossref] [PubMed]
- Yao K, Oishi T, Matsui T, Yao T, Iwashita A. Novel magnified endoscopic findings of microvascular architecture in intramucosal gastric cancer. Gastrointest Endosc 2002;56:279-84. [Crossref] [PubMed]
- Otsuka Y, Niwa Y, Ohmiya N, Ando N, Ohashi A, Hirooka Y, Goto H. Usefulness of magnifying endoscopy in the diagnosis of early gastric cancer. Endoscopy 2004;36:165-9. [Crossref] [PubMed]
- Yoshizawa M, Osawa H, Yamamoto H, Satoh K, Nakano H, Tsukui M, Sugano K. Newly developed optimal band imaging system for the diagnosis of early gastric cancer. Dig Endosc 2008;20:194-7. [Crossref]
- Miyake Y, Sekiya T, Yano T, Kubo S, Hara T. A new spectrophotometer for measuring the spectral reflectance of gastric mucous membrane. J Photographic Sci 1989;37:134-8. [Crossref]
- Shiobara T, Zhou S, Haneishi H, Tsumura N, Miyake Y. Improved color reproduction of electronic endoscopes. J Imaging Sci Technol 1996;40:494-501.
- Tsumura N, Tanaka T, Haneishi H, Miyake Y. Optimal design of mosaic color filters for the improvement of image quality in electronic endoscopes. Opt Commun 1998;145:27-32. [Crossref]
- Shiobara T, Zhou S, Haneishi H, Miyake Y. Improved color reproduction of electronic endoscopes (II)-Estimation of spectral reflectance of gastric mucous membrane by electronic endoscope. Journal of The Society of Photographic Science and Technology of Japan 1995;58:242-7.
- Yoshizawa M, Osawa H, Yamamoto H, Kita H, Nakano H, Satoh K, Shigemori M, Tsukui M, Sugano K. Diagnosis of elevated-type early gastric cancers by the optimal band imaging system. Gastrointest Endosc 2009;69:19-28. [Crossref] [PubMed]
- Osawa H, Yamamoto H, Miura Y, Yoshizawa M, Sunada K, Satoh K, Sugano K. Diagnosis of extent of early gastric cancer using flexible spectral imaging color enhancement. World J Gastrointest Endosc 2012;4:356-61. [Crossref] [PubMed]
- Pittayanon R, Rerknimitr R, Wisedopas N, Ridtitid W, Kongkam P, Treeprasertsuk S, Angsuwatcharakon P, Mahachai V, Kullavanijaya P. Flexible spectral imaging color enhancement plus probe-based confocal laser endomicroscopy for gastric intestinal metaplasia detection. J Gastroenterol Hepatol 2013;28:1004-9. [Crossref] [PubMed]
- Hoffman A, Basting N, Goetz M, Tresch A, Mudter J, Biesterfeld S, Galle PR, Neurath MF, Kiesslich R. High-definition endoscopy with i-Scan and Lugol's solution for more precise detection of mucosal breaks in patients with reflux symptoms. Endoscopy 2009;41:107-12. [Crossref] [PubMed]
- Kodashima S, Fujishiro M. Novel image-enhanced endoscopy with i-scan technology. World J Gastroenterol 2010;16:1043-9. [Crossref] [PubMed]
- Qi QQ, Zuo XL, Li CQ, Ji R, Li Z, Zhou CJ, Li YQ. High-definition magnifying endoscopy with i-scan in the diagnosis of Helicobacter pylori infection: A pilot study. J Dig Dis 2013;14:579-86. [PubMed]
- Lee JW, Lim LG, Yeoh KG. Advanced endoscopic imaging in gastric neoplasia and preneoplasia. BMJ Open Gastroenterol 2017;4:e000105. [Crossref] [PubMed]
- Inoue H, Igari T, Nishikage T, Ami K, Yoshida T, Iwai T. A novel method of virtual histopathology using laser-scanning confocal microscopy in-vitro with untreated fresh specimens from the gastrointestinal mucosa. Endoscopy 2000;32:439-43. [Crossref] [PubMed]
- Minsky M. Memoir on inventing the confocal scanning microscope. Scanning 1988;10:128-38. [Crossref]
- Goetz M, Malek NP, Kiesslich R. Microscopic imaging in endoscopy: endomicroscopy and endocytoscopy. Nat Rev Gastroenterol Hepatol 2014;11:11-8. [Crossref] [PubMed]
- Kiesslich R, Goetz M, Vieth M, Galle PR, Neurath MF. Confocal Laser Endomicroscopy. Gastrointest Endosc Clin N Am 2005;15:715-31. [Crossref] [PubMed]
- Lane PM, Lam S, Mcwilliams A, Leriche JC, Anderson MW, Macaulay CE. Confocal fluorescence microendoscopy of bronchial epithelium. J Biomed Opt 2009;14:024008. [Crossref] [PubMed]
- Piyawattanametha W, Ra H, Qiu Z, Friedland S, Liu JT, Loewke K, Kino GS, Solgaard O, Wang TD, Mandella MJ. In vivo near-infrared dual-axis confocal microendoscopy in the human lower gastrointestinal tract. J Biomed Opt 2012;17:021102. [Crossref] [PubMed]
- Polglase AL, Mclaren WJ, Skinner SA, Kiesslich R, Neurath MF, Delaney PM. A fluorescence confocal endomicroscope for in vivo microscopy of the upper- and the lower-GI tract. Gastrointest Endosc 2005;62:686-95. [Crossref] [PubMed]
- Sabapathy V, Mentam J, Jacob PM, Kumar S. Noninvasive optical imaging and in vivo cell tracking of indocyanine green labeled human stem cells transplanted at superficial or in-depth tissue of SCID mice. Stem Cells Int 2015;2015:606415. [Crossref] [PubMed]
- Goetz M, Deris I, Vieth M, Murr E, Hoffman A, Delaney P, Galle PR, Neurath MF, Kiesslich R. Near-infrared confocal imaging during mini-laparoscopy: A novel rigid endomicroscope with increased imaging plane depth. J Hepatol 2010;53:84-90. [Crossref] [PubMed]
- Yoneya S, Saito T, Komatsu Y, Koyama I, Takahashi K, Duvoll-Young J. Binding properties of indocyanine green in human blood. Invest Ophthalmol Vis Sci 1998;39:1286-90. [PubMed]
- Administration USFaD. Drugs@FDA: FDA Approved Drug Products. Available online: http://www.accessdata.fda.gov/scripts/cder/drugsatfda/index.cfm. 2011.
- Delaney PM, Harris MR, King RG. Fiber-optic laser scanning confocal microscope suitable for fluorescence imaging. Appl Opt 1994;33:573-7. [Crossref] [PubMed]
- Kiesslich R, Burg J, Vieth M, Gnaendiger J, Enders M, Delaney P, Polglase A, Mclaren W, Janell D, Thomas S. Confocal laser endoscopy for diagnosing intraepithelial neoplasias and colorectal cancer in vivo. Gastroenterology 2004;127:706-13. [Crossref] [PubMed]
- Liu JT, Mandella MJ, Ra H, Wong LK, Solgaard O, Kino GS, Piyawattanametha W, Contag CH, Wang TD. Miniature near-infrared dual-axes confocal microscope utilizing a two-dimensional microelectromechanical systems scanner. Opt Lett 2007;32:256-8. [Crossref] [PubMed]
- Gmitro AF, Aziz D. Confocal microscopy through a fiber-optic imaging bundle. Opt Lett 1993;18:565-7. [Crossref] [PubMed]
- Juškattis R, Wilson T, Watson TF. Real-time white light reflection confocal microscopy using a fibre-optic bundle. Scanning 1997;19:15-9. [Crossref]
- Jabbour JM, Saldua MA, Bixler JN, Maitland KC. Confocal Endomicroscopy: Instrumentation and Medical Applications. Ann Biomed Eng 2012;40:378-97. [Crossref] [PubMed]
- Wang J, Yang M, Yang L, Zhang Y, Yuan J, Liu Q, Hou X, Fu L. A Confocal Endoscope for Cellular Imaging. Engineering 2015;1:351-60. [Crossref]
- Yang L, Wang J, Tian G, Yuan J, Liu Q, Fu L. Five-lens, easy-to-implement miniature objective for a fluorescence confocal microendoscope. Opt Express 2016;24:473-84. [Crossref] [PubMed]
- Wang J, Li H, Tian G, Deng Y, Liu Q, Fu L. Near-infrared probe-based confocal microendoscope for deep-tissue imaging. Biomed Opt Express 2018;9:5011-25. [Crossref] [PubMed]
- Laemmel E, Genet M, Le Goualher G, Perchant A, Le Gargasson JF, Vicaut E. Fibered confocal fluorescence microscopy (Cell-viZio™) facilitates extended imaging in the field of microcirculation. J Vasc Res 2004;41:400-11. [Crossref] [PubMed]
- Perchant A, Goualher GL, Genet M, Viellerobe B. An integrated fibered confocal microscopy system for in vivo and in situ fluorescence imaging - applications to endoscopy in small animal imaging. Proc IEEE Int Symp Biomed Imaging 2004.
- Pohl H, Rösch T, Vieth M, Koch M, Becker V, Anders M, Khalifa AC, Meining A. Miniprobe confocal laser microscopy for the detection of invisible neoplasia in patients with Barrett’s oesophagus. Gut 2008;57:1648-53. [Crossref] [PubMed]
- Viellerobe B, Osdoit A, Cavé C, Lacombe F, Loiseau S, Abrat B. Mauna Kea technologies' F400 prototype: a new tool for in vivo microscopic imaging during endoscopy. Proc. SPIE 6082, Endoscopic Microscopy, 60820C (23 February 2006); doi: 10.1117/12.646625. Available online: https://doi.org/10.1117/12.646625 [Crossref]
- Sharma P, Meining AR, Coron E, Lightdale CJ, Wolfsen HC, Bansal A, Bajbouj M, Galmiche J-P, Abrams JA, Rastogi A, Gupta N, Michalek JE, Lauwers GY, Wallace MB. Real-time increased detection of neoplastic tissue in Barrett's esophagus with probe-based confocal laser endomicroscopy: final results of an international multicenter, prospective, randomized, controlled trial. Gastrointest Endosc 2011;74:465-72. [Crossref] [PubMed]
- Bertani H, Frazzoni M, Dabizzi E, Pigò F, Losi L, Manno M, Manta R, Bassotti G, Conigliaro R. Improved detection of incident dysplasia by probe-based confocal laser endomicroscopy in a Barrett’s esophagus surveillance program. Dig Dis Sci 2013;58:188-93. [Crossref] [PubMed]
- Bok GH, Jeon SR, Cho JY, Cho J-H, Lee WC, Jin SY, Choi IH, Kim HG, Lee TH, Park EJ. The accuracy of probe-based confocal endomicroscopy versus conventional endoscopic biopsies for the diagnosis of superficial gastric neoplasia (with videos). Gastrointest Endosc 2013;77:899-908. [Crossref] [PubMed]
- Lim LG, Yeoh KG, Srivastava S, Chan YH, Teh M, Ho KY. Comparison of probe-based confocal endomicroscopy with virtual chromoendoscopy and white-light endoscopy for diagnosis of gastric intestinal metaplasia. Surg Endosc 2013;27:4649-55. [Crossref] [PubMed]
- van den Broek FJC, van Es JA, van Eeden S, Stokkers PCF, Ponsioen CY, Reitsma JB, Fockens P, Dekker E. Pilot study of probe-based confocal laser endomicroscopy during colonoscopic surveillance of patients with longstanding ulcerative colitis. Endoscopy 2011;43:116-22. [Crossref] [PubMed]
- Rispo A, Castiglione F, Staibano S, Esposito D, Siano M, Maione F, Salvatori F, Masone S, Persico M, Palma GDD. Diagnostic accuracy of confocal laser endomicroscopy in diagnosing dysplasia in patients affected by long-standing ulcerative colitis. World J Gastrointest Endosc 2012;4:414-20. [Crossref] [PubMed]
- Sharma P, Meining AR, Coron E, Lightdale CJ, Wolfsen HC, Bansal A, Bajbouj M, Galmiche JP, Abrams JA, Rastogi A, Gupta N, Michalek JE, Lauwers GY, Wallace MB. Real-time increased detection of neoplastic tissue in Barrett's esophagus with probe-based confocal laser endomicroscopy: final results of an international multicenter, prospective, randomized, controlled trial. Gastrointest Endosc 2011;74:465-72. [Crossref] [PubMed]
- Ohmiya N, Horiguchi N, Tahara T, Nagasaka M, Nakagawa Y, Shibata T, Tsukamoto T, Kuroda M. In vivo characterization of abnormalities in small-bowel diseases using probe-based confocal laser endomicroscopy. Endosc Int Open 2017;5:E547-E558. [Crossref] [PubMed]
- Vincent P, Maskos U, Charvet I, Bourgeais L, Stoppini L, Leresche N, Changeux JP, Lambert R, Meda P, Paupardin‐Tritsch D. Live imaging of neural structure and function by fibred fluorescence microscopy. EMBO Rep 2006;7:1154-61. [Crossref] [PubMed]
- Gore BB, Soden ME, Zweifel LS. Visualization of plasticity in fear-evoked calcium signals in midbrain dopamine neurons. Learn Mem 2014;21:575-9. [Crossref] [PubMed]
- Gao H, Yang Z, Zhang S, Cao S, Pang Z, Yang X, Jiang X. Glioma-homing peptide with a cell-penetrating effect for targeting delivery with enhanced glioma localization, penetration and suppression of glioma growth. J Control Release 2013;172:921-8. [Crossref] [PubMed]
- Wang CC, Xu H, Man GCW, Zhang T, Chu KO, Chu CY, Cheng JTY, Li G, He YX, Qin L, Lau TS, Kwong J, Chan TH. Prodrug of green tea epigallocatechin-3-gallate (Pro-EGCG) as a potent anti-angiogenesis agent for endometriosis in mice. Angiogenesis 2013;16:59-69. [Crossref] [PubMed]
- Wang K, Zhang X, Liu Y, Liu C, Jiang B, Jiang Y. Tumor penetrability and anti-angiogenesis using iRGD-mediated delivery of doxorubicin-polymer conjugates. Biomaterials 2014;35:8735-47. [Crossref] [PubMed]
- Huang D, Swanson EA, Lin CP, Schuman JS, Stinson WG, Chang W, Hee MR, Flotte T, Gregory K, Puliafito CA. Optical coherence tomography. Science 1991;254:1178-81. [Crossref] [PubMed]
- Jäckle S, Gladkova N, Feldchtein F, Terentieva A, Brand B, Gelikonov G, Gelikonov V, Sergeev A, Fritscher-Ravens A, Freund J, Seitz U, Schröder S, Soehendra N. In vivo endoscopic optical coherence tomography of the human gastrointestinal tract - toward optical biopsy. Endoscopy 2000;32:743-9. [Crossref] [PubMed]
- Kirtane TS, Wagh MS. Endoscopic optical coherence tomography (OCT): advances in gastrointestinal imaging. Gastroenterol Res Pract 2014;2014:376367. [Crossref] [PubMed]
- Li XD, Boppart SA, Van Dam J, Mashimo H, Mutinga M, Drexler W, Klein M, Pitris C. L. Krinsky M, Brezinski ME, Fujimoto JG. Optical coherence tomography: advanced technology for the endoscopic imaging of Barrett's esophagus. Endoscopy 2000;32:921-30. [Crossref] [PubMed]
- Chen Y, Aguirre A, Hsiung P-L, Desai S, Herz P, Pedrosa M, Huang Q, Figueiredo M, Huang S-W, Koski A. Ultrahigh resolution optical coherence tomography of Barrett’s esophagus: preliminary descriptive clinical study correlating images with histology. Endoscopy 2007;39:599-605. [Crossref] [PubMed]
- Hatta W, Uno K, Koike T, Yokosawa S, Iijima K, Imatani A, Shimosegawa T. Optical coherence tomography for the staging of tumor infiltration in superficial esophageal squamous cell carcinoma. Gastrointest Endosc 2010;71:899-906. [Crossref] [PubMed]
- Shen B, Zuccaro G Jr, Gramlich TL, Gladkova N, Trolli P, Kareta M, Delaney CP, Connor JT, Lashner BA, Bevins CL. In vivo colonoscopic optical coherence tomography for transmural inflammation in inflammatory bowel disease. Clin Gastroenterol Hepatol 2004;2:1080-7. [Crossref] [PubMed]
- Wang LV, Hu S. Photoacoustic tomography: in vivo imaging from organelles to organs. Science 2012;335:1458-62. [Crossref] [PubMed]
- Zou C, Wu B, Dong Y, Song Z, Zhao Y, Ni X, Yang Y, Liu Z. Biomedical photoacoustics: fundamentals, instrumentation and perspectives on nanomedicine. Int J Nanomedicine 2016;12:179-95. [Crossref] [PubMed]
- Beard P. Biomedical photoacoustic imaging. Interface Focus 2011;1:602-31. [Crossref] [PubMed]
- Xu M, Wang LV. Photoacoustic imaging in biomedicine. Rev Sci Instrum 2006;77:041101. [Crossref]
- Wu HQ, Wang HY, Xie WM, Wu SL, Li ZF, Zhang XM, Li H. Scanning photoacoustic imaging of submucosal gastric tumor based on a long focused transducer in phantom and in vitro experiments. J Innov Opt Heal Sci 2019. DOI:. [Crossref]
- Chow CM, Zhou Y, Guo Y, Norris TB, Wang X, Deng CX, Ye JY. Broadband optical ultrasound sensor with a unique open-cavity structure. J Biomed Opt 2011;16:017001. [Crossref] [PubMed]
- Peterson R, Solis S, Zhang B, Huang H, Ye JY. Sensitivity enhancement of an open-cavity-based optoacoustic sensor. Opt Lett 2013;38:2739-41. [Crossref] [PubMed]
- Yang J-M, Maslov K, Yang H-C, Zhou Q, Shung KK, Wang LV. Photoacoustic endoscopy. Opt Lett 2009;34:1591-3. [Crossref] [PubMed]
- Su JL, Wang B, Wilson KE, Bayer CL, Chen YS, Kim S, Homan KA, Emelianov SY. Advances in clinical and biomedical applications of photoacoustic imaging. Expert Opin Med Diagn 2010;4:497-510. [Crossref] [PubMed]
- Guo H, Song C, Xie H, Xi L. Photoacoustic endomicroscopy based on a MEMS scanning mirror. Opt Lett 2017;42:4615-8. [Crossref] [PubMed]
- Ansari R, Zhang E, Mathews S, Desjardins AE, Beard PC. Photoacoustic endoscopy probe using a coherent fibre-optic bundle. Proc. SPIE 9539, Opto-Acoustic Methods and Applications in Biophotonics II, 953905 (16 July 2015); doi: 10.1117/12.2183820; available online: https://doi.org/10.1117/12.2183820 [Crossref]
- Yang JM, Li C, Chen R, Zhou Q, Shung KK, Wang LV. Catheter-based photoacoustic endoscope. J Biomed Opt 2014;19:066001. [Crossref] [PubMed]
- Yang JM, Favazza C, Chen R, Yao J, Cai X, Maslov K, Zhou Q, Shung KK, Wang LV. Volumetric photoacoustic endoscopy. Biomedical Optics 2012; Miami, Florida: Optical Society of America.
- Yang JM, Chen R, Favazza C, Yao J, Li C, Hu Z, Zhou Q, Shung KK, Wang LV. A. 2.5-mm diameter probe for photoacoustic and ultrasonic endoscopy. Opt Express 2012;20:23944-53. [Crossref] [PubMed]
- Yuan Y, Yang S, Xing D. Preclinical photoacoustic imaging endoscope based on acousto-optic coaxial system using ring transducer array. Opt Lett 2010;35:2266-8. [Crossref] [PubMed]
- Pierangelo A, Fuks D, Validire P, Benali A, Gayet B. Diagnostic accuracy of confocal laser endomicroscopy for the characterization of liver nodules. Eur J Gastroenterol Hepatol 2017;29:42. [Crossref] [PubMed]
- Tajiri H. Autofluorescence endoscopy for the gastrointestinal tract. Proc Jpn Acad Ser B Phys Biol Sci 2007;83:248-55. [Crossref] [PubMed]
- Falk GW. Autofluorescence Endoscopy. Gastrointest Endosc Clin N Am 2009;19:209-20. [Crossref] [PubMed]
- Kendall C, Stone N, Shepherd N, Geboes K, Warren B, Bennett R, Barr H. Raman spectroscopy, a potential tool for the objective identification and classification of neoplasia in Barrett's oesophagus. J Pathol 2003;200:602-9. [Crossref] [PubMed]
- Teh SK, Zheng W, Ho KY, Teh M, Yeoh KG, Huang Z. Near-infrared Raman spectroscopy for optical diagnosis in the stomach: identification of Helicobacter-pylori infection and intestinal metaplasia. Int J Cancer 2010;126:1920-7. [Crossref] [PubMed]
- ASGE Technology Committee. Kwon RS, Wong Kee Song LM, Adler DG, Conway JD, Diehl DL, Farraye FA, Kantsevoy SV, Kaul V, Kethu SR, Mamula P, Pedrosa MC, Rodriguez SA, Tierney WM. Endocytoscopy. Gastrointest Endosc 2009;70:610-3.
- Neumann H, Fuchs FS, Vieth M, Atreya R, Siebler J, Kiesslich R, Neurath MF. Review article: in vivo imaging by endocytoscopy. Aliment Pharmacol Ther 2011;33:1183-93. [Crossref] [PubMed]
- Sato H, Inoue H, Hayee BH, Ikeda H, Sato C, Phalanusitthepha C, Santi EGR, Kobayashi Y, Kudo S-e. In vivo histopathology using endocytoscopy for non-neoplastic changes in the gastric mucosa: a prospective pilot study (with video). Gastrointest Endosc 2015;81:875-81. [Crossref] [PubMed]
- Pavlov A, Borisova E, Pavlova O, Agranovich I, Khorovodov A, Terskov A, Mamedova A, Klimova M, Avramov L, Semyachkina-Glushkovskaya O. Photodynamic diagnostics of early gastric cancer: Complexity measures of gastric microcirculation and new model of metastatic adenocarcinoma of rat stomach. J Innov Opt Heal Sci 2019;12:1950007. [Crossref]
- Hong X, Nagarajan VK, Mugler DH, Yu B. Smartphone microendoscopy for high resolution fluorescence imaging. J Innov Opt Heal Sci 2016;09:1650046. [Crossref]