Ultrasound in neonatal lung disease
Introduction
Lung disease is the most common cause of neonatal respiratory distress, which can result in respiratory failure and death in severe cases. In the United States, the mortality of neonatal respiratory failure is approximately 11%, and the rate can go up to 32% in China (1,2). Thus, identification of the etiology of lung disease is important for neonatologists. Traditionally, chest X-ray has been considered as the most valuable imaging modality for the diagnosis of lung diseases, but it unavoidably causes radiation damage to the patient. Neonates are susceptible to radiation because they have rapidly dividing cells that cannot repair mutated DNA. According to one study, the risk of cancer induction in infants receiving a single small dose of radiation is 2–3 times higher than the average population and 6–9 times higher than the risk from an exposure of a 60-year-old patient (3). In recent years, a new imaging application has been introduced in clinical practice—lung ultrasound. Several studies have demonstrated that lung ultrasound is an accurate and reliable technique for the diagnosis of neonatal lung diseases (4,5). Other advantages of lung ultrasound include that it is non-ionizing, easy to operate, and the imaging is performed in real-time, thus making it as a potential tool to be used in neonatal intensive care units (NICUs). In this paper, we will introduce the current state of knowledge about neonatal lung ultrasound, show the imaging of typical cases and provide a very good starting point for residents or novices to get a better understanding of lung ultrasound.
Lung ultrasound technique
All diagnostic ultrasound methods are based on the principle that ultrasound is reflected by an interface between media with different acoustic impedance (6). Ultrasound is limited in normal aerated lungs because no acoustic mismatch occurs in the ultrasound beam when it encounters air (7). The pleural line and repetitive hyperechoic horizontal lines (A-lines) can be visualized by ultrasound. The pleural line is a smooth and regular hyperechoic line that moves to-and-fro during respiration, which is called lung sliding (8). The A-lines are a series of parallel lines at regular intervals below the pleural line. They represent the large change in acoustic impedance at the pleura-lung interface and generate horizontal artifacts (Figure 1A) (9). When the air content decreases, i.e., subpleural interstitial edema, the ultrasound beam generates an acoustic mismatch between the fluid interfaces surrounded by air and reflects repeatedly at the deeper zones (10). This phenomenon creates vertical reverberation artifacts called B-lines. B-lines are hyperechoic, laser-like images that originate from the pleural line and reach the edge of the screen, moving with respiration (Figure 1B) (9). B-lines are correlated with lung interstitial fluid content, and their number increases with decreasing air content. Multiple B-lines indicate alveolar-interstitial syndrome, which has been confirmed in several studies by clinical and radiologic diagnoses (11,12). Evidence of compact B-lines in lung fields implies a severe alveolar-interstitial syndrome, known as “white lung” (Figure 1C). When the air content further decreases, i.e., lung consolidation, lung parenchyma is directly visualized by opening an acoustic window on the lung (7). Lung consolidation is described as a region of hypoechoic, poorly defined or wedge-shaped borders. The presence of air bronchogram or vascular pattern may help to identify the etiology of the consolidation (Figure 1D) (13).
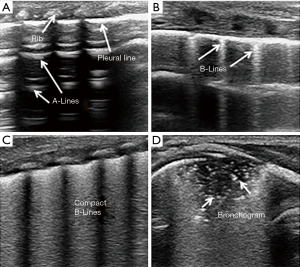
For the relatively thinner chest walls and smaller thoraxes in neonates, a high frequency linear probe is preferred, which enables better image quality and allows the entire surface of the lungs to be visualized. Lung ultrasound is performed in a supine, lateral or prone position in neonates. Each hemithorax is divided into anterior, lateral, and posterior regions by the anterior and posterior axillary lines, longitudinal and transverse scans are performed in all areas.
Clinical applications
Respiratory distress syndrome (RDS)
RDS, also known as hyaline membrane disease, is a major cause of neonatal mortality. It mainly occurs in preterm infants and correlates with structural and functional lung immaturity. Published data have shown that approximately 70% of cases occur in infants at less than 28 weeks of gestation, whereas 15–30% of cases occur in infants between 32 to 36 weeks of gestation (14). Pulmonary surfactant deficiency is an important pathogenesis of RDS. Surfactant is a phospholipid mixture produced by type II pneumocytes that enables a decrease in alveolar surface tension to support breathing. Immature alveolar cells produce less surfactant, resulting in poor gas exchange and alveoli collapse. Infants with RDS show signs of respiratory distress after birth or within 4–6 hours and usually require mechanical ventilation.
The ultrasonic features of RDS are compact B-lines with an echographic white lung appearance, the presence of a thickened and irregular pleural line, and multiple subpleural lung consolidations indicating alveolar collapse (Figure 2). These signs were confirmed by Copetti et al. to have sensitivity and specificity of 100% for the diagnosis of RDS (15). Vergine et al. enrolled 59 neonates with clinically suspected respiratory distress, and 23 of them were diagnosed as RDS. The sensitivity and specificity of lung ultrasound for the diagnosis of RDS were 95.6% and 94.4%, respectively, which were higher than those of chest X-ray (91.3% and 84.2%, respectively) (16).
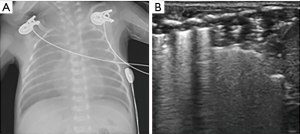
Sonographic appearance of RDS is not always symmetrically, not only inconsistent in bilateral lung but also varied between the different lung fields on the same side (Figure 3). In neonates, due to their continuous supine position, lung consolidations and compact B-lines are often presented at the posterior areas, which may be associated with reduced ventilation in these areas (17). Therefore, it is necessary to strengthen the scanning of the posterior areas. The administration of surfactant rapidly and effectively improves chest X-ray images of RDS patients by increasing lung aeration. However, the clinical conditions of patients are not always equally improved and may require maintenance of mechanical ventilation for several days. Similarly, lung ultrasound shows persistent compact B-lines that do not substantially change within the first 48 hours or even in the following days (15). Surfactant administration did not improve lung fluid clearance in experiments on preterm rabbits. Persistent compact B-lines reflect the impairment of lung fluid clearance and may be associated with interstitial immaturity in preterm neonates (18).
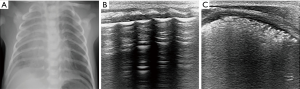
Transient tachypnea of the newborn (TTN)
TTN or “wet lung” is the most common cause of neonatal respiratory distress. This disorder is a benign condition, caused by the delayed clearance of fetal lung fluid. The fetal lungs are filled with fluid before birth, a fraction of the fluid is removed by the vaginal squeezing of the thorax during delivery, and the remaining fluid is transported to the interstitial space by lung epithelial sodium channels (ENaC), followed by removal via pulmonary circulation and the lymphatic system (19). Elective cesarean section and immature gestational age have been confirmed as the main risk factors for TTN, which may be associated with the diminished activity or immaturity of ENaC (20). Most infants with TTN present mild or transient respiratory distress and usually rapidly improve within 24 hours but in some cases may persist for several days.
The main characteristic of TTN is pulmonary edema, which primarily presents as double lung points, alveolar interstitial syndrome or white lung. The pleural line is normal, well-defined and regular in bilateral lungs. The double lung point is a demarcation point for echogenic differences in the lung field. Ultrasound images show compact B-lines in the inferior lung field and rare B-lines in the superior lung field (Figure 4). In the past, the presence of a double lung point exhibited 100% sensitivity and specificity for the diagnosis of TTN (21). However, a large sample study found that the double lung point exhibited only 45.6% sensitivity, 95.8% specificity for TTN. Mild TTN primarily manifested as double lung point or alveolar interstitial syndrome, whereas severe TTN presented as white lung. Additionally, the double lung point also appears during the recovery period of diseases, such as severe TTN, RDS, and pneumonia (22). TTN diagnosis remains a source of confusion for clinicians because patients present respiratory distress in the early stages, which is difficult to distinguish between RDS. Secondly, X-ray has a low sensitivity and specificity in the detection of pulmonary edema. One study indicated only 48% agreement in the diagnosis of TTN between clinicians and radiologists compared with 95% agreement for RDS (23). Lung ultrasound enables the detection of pulmonary edema and thus can effectively diagnose TTN (Figures 5,6). In a prospective cohort study of 59 neonates with respiratory distress, Vergina et al. identified that the sensitivity and specificity of lung ultrasound for TTN were 93.3% and 96.5%, which were higher than 89.4% and 91.3% for X-ray, respectively (16). Thus, current evidence on lung ultrasound for the diagnosis of TTN is accurate and reliable.
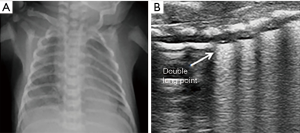
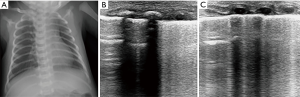
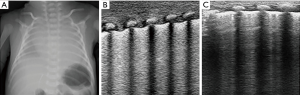
Pneumonia
Pneumonia carries the greatest risk of death during the neonatal period and it is responsible for approximately 0.75–1.2 million neonatal deaths per year, accounting for 10% of global child mortality (24). The pathogens associated with neonatal pneumonia include numerous bacteria, fungi and viruses, which may be acquired by intrauterine or postpartum, e.g., via maternal chorioamnionitis, inhalation of infected amniotic fluid or cross-contamination (25). These pathogens may cause airway epithelial injury, protein fluid leakage and alveolar interstitial edema. The clinical signs of neonatal pneumonia are nonspecific and presented as respiratory distress, which is often difficult to distinguish from RDS and TTN. Clinical information and laboratory investigations may be helpful for distinguishing pneumonia.
The ultrasonographic findings of pneumonia in child include lung consolidation with irregular margins and surrounding multiple B-lines. The pleural line is not visible in the area, and lung sliding is absent (Figure 7). Dynamic air bronchograms (branch structures) are visible in large consolidations and represent bronchial patency, which excludes atelectasis (26). Few studies have reported the role of ultrasound in the detection of neonatal pneumonia. Liu et al. studied lung ultrasound in 40 neonates with radiological and clinical histories of pneumonia and 40 without lung disease. The ultrasound signs included large areas of lung consolidation with air bronchograms, pleural line abnormalities, and interstitial syndrome. The authors concluded that lung ultrasound is a reliable tool for diagnosing neonatal pneumonia and it is suitable for routine use in the NICU (27). A meta-analysis of eight studies found that the sensitivity and specificity were 96% and 93%, respectively, for the diagnosis of childhood pneumonia. Thus, lung ultrasound may be used as an imaging alternative for the diagnosis of childhood pneumonia (28). However, there was a certain degree of limitation due to the low sensitivity of lung ultrasound for detecting pneumonia in perihilar area (29,30).
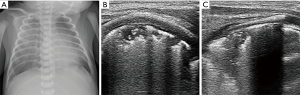
Meconium aspiration syndrome (MAS)
The inhalation of meconium-stained amniotic fluid during parturition in infants may result in MAS, particularly in the term or post-term infants. Meconium is a green viscous substance normally stored in the fetal intestines. When the fetus suffers from hypoxia, acidemia, or infection, it may trigger meconium passage (31). Meconium can cause airway obstruction, surfactant dysfunction, chemical pneumonitis and secondary infection. Airway obstruction has been considered to be the primary mechanism of MAS, which can lead to ventilation/perfusion mismatch and air leak (32). Infants with MAS show signs of yellowish or greenish meconium staining on the skin, umbilical cord and nails, and respiratory distress may occur immediately after birth.
MAS is regarded as a specific type of pneumonia, its ultrasonographic appearances are similar to pneumonia, manifests as irregular subpleural consolidations and coalescent B-lines, and the consolidation distribution may be more evident on one side (33). Piastra et al. conducted a study of 6 neonates with MAS of variable severity using different ultrasound probes. The findings showed that ultrasound signs of coalescent or sparse B-lines, consolidations, atelectasis and bronchograms, which were corresponded well with X-ray findings (34). Similar ultrasonographic characteristics were also found in a study by Liu et al., which concluded that lung ultrasound is an accurate, reliable, convenient and non-invasive method to diagnose MAS (35).
Bronchopulmonary dysplasia (BPD)
BPD is a common complication related to preterm delivery, with high mortality and long-term morbidity. It mainly occurs in infants with a gestational age less than 28 weeks and birth weights below the median (36). Aberrant and arrested pulmonary development is the predominant characteristics of BPD, its factors involved structurally and biochemically immature lung, infection, oxidant injury, and poor nutrition (37). BPD is a common complication of RDS, and exhibits a high incidence in surviving infants with RDS. These patients often require long-term oxygen therapy and may experience lifelong alterations in cardiopulmonary function.
In BPD, coalescent B-lines are non-homogeneously distributed and always accompanied with multiple different-sized subpleural consolidations, as well as thickened pleural lines (Figures 8,9) (33). These ultrasonographic changes usually occur in infants with RDS who have been treated for serval weeks. Previous studies have shown that a transabdominal probe can be used to detect retrodiaphragmatic hyperechogenicity to predict the development of BPD (38,39). However, this method was not adopted in later study because it is difficult to observe the changes in subpleural consolidation and abnormal pleural line.

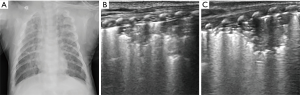
Atelectasis
Atelectasis is a collapse of the lung parenchyma that results in lack of gas exchange and loss of volume in lung. It is an underlying disease that may be associated with a variety of pulmonary disorders, rather than the disease per se. The mechanisms of atelectasis can be divided into three types: (I) airway obstruction; (II) compression of parenchyma by intrathoracic or extrathoracic factors; and (III) increased surface tension in alveoli and bronchioli (40). Airway obstruction (e.g., mucus plug or meconium) is the main cause of atelectasis in infants. As the airways in infants are relatively narrow, obstruction leads to gas being trapped in the distal airway and absorbed by the blood that flows through the region of the lung, producing alveolar hypoxia and collapse. In addition, the incidence of right upper lobe atelectasis in mechanically ventilated infants may be related to the traumatic damage to the mucosa of the right sided bronchi (41).
The ultrasonographic features of atelectasis include large lung consolidation with well-defined borders, pleural line abnormalities, and the absence of lung sliding (Figure 10). A study by Liu et al. evaluated the usefulness of lung ultrasound in the diagnosis of neonatal atelectasis and compared it with chest X-ray. The findings showed that the sensitivity of ultrasound and chest X-ray were 100% and 75%, respectively. Cases of atelectasis that were not detected by chest X-ray were eventually confirmed by CT (42). In another study, Acosta et al. investigated the accuracy of lung ultrasound in the diagnosis of anesthesia-induced atelectasis in children using MRI as reference. Lung ultrasound exhibited 88% sensitivity, 89% specificity, and 88% accuracy for the detection of atelectasis (43). Thus, lung ultrasound is an accurate, reliable, and simple bedside method for diagnosing atelectasis.
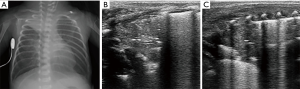
Pneumothorax
Pneumothorax refers to air that accumulates in the pleural space and exerts pressure to collapse the lung. The incidence of pneumothorax in neonates is only 1–2%, however the rate may be as high as 30% in patients with underlying lung diseases or mechanical ventilation (44). According to the cause of the pneumothorax, it can be categorized as spontaneous, tension, or traumatic. Neonatal spontaneous pneumothorax tends to occur in clinical situations, such as aspiration of meconium or amniotic fluid. Airway obstruction creates the ball-valve effect and pressure increased can lead to alveolar rupture. Tension pneumothorax mainly occurs in neonates receiving mechanical ventilation and may be associated with disease, improper suctioning techniques, or birth trauma (45).
Ultrasound images of pneumothorax exhibit the absence of lung sliding, absence of B-lines, and presence of a lung point (Figure 11). The absence of lung sliding and B-lines is caused by air accumulation in the pleural cavity, resulting in a lack of movement of the visceral pleura. The lung point is a specific sign of pneumothorax, the appearance of a normal lung pattern is replaced by a pneumothorax pattern in a particular location on the chest wall (46). It is worth noting that the lung point may be missing in patients with severe pneumothorax. Raimondi et al. conducted a study to evaluate the accuracy of lung ultrasound for the detection of pneumothorax. The study enrolled 42 infants in six centers; pneumothorax was detected in 26 infants. Lung ultrasound had sensitivity, specificity, positive predictive value and negative predictive value of 100% for the diagnosis of pneumothorax (47). Cattarossi et al. conducted a study to compare the sensitivity and specificity for pneumothorax diagnosis in neonates using three imaging techniques. The study showed that the sensitivity and specificity were 100% for lung ultrasound, 96% and 100% for chest X-ray, and 87% and 96% for chest transillumination. Thus, the authors concluded that lung ultrasound is as accurate as X-ray for the diagnosis of pneumothorax (48).
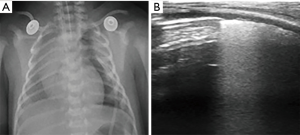
Neonatal lung diseases often cause confusion for clinicians due to the non-specific of respiratory distress in patients. Lung ultrasound is a new tool for detecting lung diseases in clinical practice and exhibits high sensitivity and specificity. However, lung ultrasound is suggested not to be performed in isolation; it should be combined with the clinical findings of patients. In addition, it should be kept in mind that lung ultrasound has a limitation in evaluating the consolidation not in contact with the pleura. Table 1 summarizes the clinical findings in various types of neonatal lung disease, as well as the features of chest X-ray and lung ultrasound.
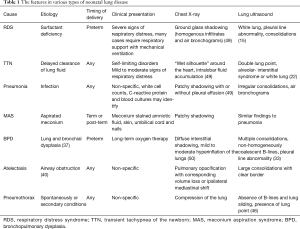
Full table
Other applications
At present, lung ultrasound is not only used for the diagnosis of neonatal lung diseases but also plays a positive role in real-time monitoring and assessment. Sameshima et al. reported a case of a 3-month-old infant who achieved lung recruitment with positive end-expiratory pressure under real-time ultrasound guidance, thereby avoiding ionizing radiation injury by CT (51). Liu et al. performed bronchoalveolar lavage in 57 neonates with atelectasis under ultrasound monitoring, and the results indicated that the total effective rate of was 93%. The authors concluded that lung ultrasound can be an effective method for clinically monitoring bronchoalveolar lavage in neonates (52). Studies by Raimondi et al. used three simple ultrasound patterns (white lung, B-lines, and A-lines) to monitor fluid clearance and respiratory adaptation in neonates, and the results indicated that lung ultrasound was useful in predicting the need for respiratory support (53). Based on the above pattern, Brat et al. further incorporated lung consolidation into examinations and assigned a score to each pattern. The study showed that the lung ultrasound score was significantly correlated with the oxygenation status in neonates and showed good reliability for predicting the need for surfactant administration in premature infants (54). Lung ultrasound provides valuable real-time information, which makes it particularly promising for further applications in NICUs.
Conclusions
In recent years, neonatal lung ultrasound has been widely used in clinical practice. Ultrasound findings combined with clinical information are useful for the diagnosis of neonatal lung diseases. In addition, lung ultrasound is a safe, low-cost, and easy to operate tool that can be repeatedly and rapidly performed at the bedside without anesthetic drugs. Given all its advantages, lung ultrasound is worthy of clinical application and promotion.
Acknowledgements
Funding: This work was supported by the Research Projects of Nature Science Fund of China (No. 81671707), Research Projects of Guangdong Science and Technology (No. 2016A030311054), Higher Education Colleges and Universities Innovation Strong School Project (Q17024072), and Research Fund of National Education Steering Committee for Graduates in Medical Degree (B3-20170302-06).
Footnote
Conflicts of Interest: The authors have no conflicts of interest to declare.
References
- Angus DC, Linde-Zwirble WT, Clermont G, Griffin MF, Clark RH. Epidemiology of neonatal respiratory failure in the United States: projections from California and New York. Am J Respir Crit Care Med 2001;164:1154-60. [Crossref] [PubMed]
- Qian L, Liu C, Zhuang W, Guo Y, Yu J, Chen H, Wang S, Lin Z, Xia S, Ni L, Liu X, Chen C, Sun B. Chinese Collaborative Study Group for Neonatal Respiratory Diseases. Neonatal respiratory failure: a 12-month clinical epidemiologic study from 2004 to 2005 in China. Pediatrics 2008;121:e1115-24. [Crossref] [PubMed]
- Hall EJ. Lessons we have learned from our children: cancer risks from diagnostic radiology. Pediatr Radiol 2002;32:700-6. [Crossref] [PubMed]
- Liu J, Cao HY, Wang XL, Xiao LJ. The significance and the necessity of routinely performing lung ultrasound in the neonatal intensive care units. J Matern Fetal Neonatal Med 2016;29:4025-30. [Crossref] [PubMed]
- Chen SW, Fu W, Liu J, Wang Y. Routine application of lung ultrasonography in the neonatal intensive care unit. Medicine (Baltimore) 2017;96. [Crossref] [PubMed]
- Schiller NB, Shah PM, Crawford M, DeMaria A, Devereux R, Feigenbaum H, Gutgesell H, Reichek N, Sahn D, Schnittger I, et al. American Society of Echocardiography Committee on Standards, Subcommittee on Quantitation of Two-dimensional Echocardiograms. J Am Soc Echocardiogr 1989;2:358-67. [Crossref] [PubMed]
- Gargani L. Lung ultrasound: a new tool for the cardiologist. Cardiovasc Ultrasound 2011;9:6. [Crossref] [PubMed]
- Mathis G., Thoraxsonography-Part I. Chest wall and pleura. Ultrasound Med Biol 1997;23:1131-9. [Crossref] [PubMed]
- Lichtenstein DA, Mezière GA, Lagoueyte JF, Biderman P, Goldstein I, Gepner A. A-lines and B-lines: lung ultrasound as a bedside tool for predicting pulmonary artery occlusion pressure in the critically ill. Chest 2009;136:1014-20. [Crossref] [PubMed]
- Jambrik Z, Monti S, Coppola V, Agricola E, Mottola G, Miniati M, Picano E. Usefulness of ultrasound lung comets as a nonradiologic sign of extravascular lung water. Am J Cardiol 2004;93:1265-70. [Crossref] [PubMed]
- Lichtenstein D, Mézière G, Biderman P, Gepner A, Barré O. The comet-tail artifact. An ultrasound sign of alveolar-interstitial syndrome. Am J Respir Crit Care Med 1997;156:1640-6. [Crossref] [PubMed]
- Volpicelli G, Mussa A, Garofalo G, Cardinale L, Casoli G, Perotto F, Fava C, Frascisco M. Bedside lung ultrasound in the assessment of alveolar-interstitial syndrome. Am J Emerg Med 2006;24:689-96. [Crossref] [PubMed]
- Weinberg B, Diakoumakis EE, Kass EG, Seife B, Zvi ZB. The air bronchogram: sonographic demonstration. AJR Am J Roentgenol 1986;147:593-5. [Crossref] [PubMed]
- Hjalmarson O. Epidemiology and classification of acute, neonatal respiratory disorders: a prospective study. Acta Paediatr Scand 1981;70:773-83. [Crossref] [PubMed]
- Copetti R, Cattarossi L, Macagno F, Violino M, Furlan R. Lung ultrasound in respiratory distress syndrome: a useful tool for early diagnosis. Neonatology 2008;94:52-9. [Crossref] [PubMed]
- Vergine M, Copetti R, Brusa G, Cattarossi L. Lung ultrasound accuracy in respiratory distress syndrome and transient tachypnea of the newborn. Neonatology 2014;106:87-93. [Crossref] [PubMed]
- Lovrenski J. Lung ultrasonography of pulmonary complications in preterm infants with respiratory distress syndrome. Ups J Med Sci 2012;117:10-7. [Crossref] [PubMed]
- Cattarossi L, Copetti R, Poskurica B, Miserocchi G. Surfactant administration for neonatal respiratory distress does not improve lung interstitial fluid clearance: echographic and experimental evidence. J Perinat Med 2010;38:557-63. [Crossref] [PubMed]
- Yurdakök M. Transient tachypnea of the newborn: what is new? J Matern Fetal Neonatal Med 2010;23:24-26. [Crossref] [PubMed]
- Guglani L, Lakshminrusimha S, Ryan RM. Transient tachypnea of the newborn. Pediatr Rev 2008;29:e59-65. [Crossref] [PubMed]
- Copetti R, Cattarossi L. The ‘double lung point’: an ultrasound sign diagnostic of transient tachypnea of the newborn. Neonatology 2007;91:203-9. [Crossref] [PubMed]
- Liu J, Chen XX, Li XW, Chen SW, Wang Y, Fu W. Lung ultrasonography to diagnose transient tachypnea of the newborn. Chest 2016;149:1269-75. [Crossref] [PubMed]
- Kurl S, Heinonen KM, Kiekara O. The first chest radiograph in neonates exhibiting respiratory distress at birth. Clin Pediatr (Phila) 1997;36:285-9. [Crossref] [PubMed]
- Duke T. Neonatal pneumonia in developing countries. Arch Dis Child Fetal Neonatal Ed 2005;90:F211-9. [Crossref] [PubMed]
- Nissen MD. Congenital and neonatal pneumonia. Paediatr Respir Rev 2007;8:195-203. [Crossref] [PubMed]
- Copetti R, Cattarossi L. Ultrasound diagnosis of pneumonia in children. Radiol Med 2008;113:190-8. [Crossref] [PubMed]
- Liu J, Liu F, Liu Y, Wang HW, Feng ZC. Lung ultrasonography for the diagnosis of severe neonatal pneumonia. Chest 2014;146:383-8. [Crossref] [PubMed]
- Pereda MA, Chavez MA, Hooper-Miele CC, Gilman RH, Steinhoff MC, Ellington LE, Gross M, Price C, Tielsch JM, Checkley W. Lung ultrasound for the diagnosis of pneumonia in children: a meta-analysis. Pediatrics 2015;135:714-22. [Crossref] [PubMed]
- Urbankowska E, Krenke K, Drobczyński Ł, Korczyński P, Urbankowski T, Krawiec M, Kraj G, Brzewski M, Kulus M. Lung ultrasound in the diagnosis and monitoring of community acquired pneumonia in children. Respir Med 2015;109:1207-12. [Crossref] [PubMed]
- Reali F, Sferrazza Papa GF, Carlucci P, Fracasso P, Di Marco F, Mandelli M, Soldi S, Riva E, Centanni S. Can lung ultrasound replace chest radiography for the diagnosis of pneumonia in hospitalized children? Respiration 2014;88:112-5. [Crossref] [PubMed]
- Poggi SH, Ghidini A. Pathophysiology of meconium passage into the amniotic fluid. Early Hum Dev 2009;85:607-10. [Crossref] [PubMed]
- Chettri S, Bhat BV, Adhisivam B. Current Concepts in the Management of Meconium Aspiration Syndrome. Indian J Pediatr 2016;83:1125-30. [Crossref] [PubMed]
- Cattarossi L. Lung ultrasound: its role in neonatology and pediatrics. Early Hum Dev 2013;89 Suppl 1:S17-S19. [Crossref] [PubMed]
- Piastra M, Yousef N, Brat R, Manzoni P, Mokhtari M, De Luca D. Lung ultrasound findings in meconium aspiration syndrome. Early Hum Dev 2014;90 Suppl 2:S41-S43. [Crossref] [PubMed]
- Liu J, Cao HY, Fu W. Lung ultrasonography to diagnose meconium aspiration syndrome of the newborn. J Int Med Res 2016;44:1534-42. [Crossref] [PubMed]
- Kewitz G, Wudel S, Hopp H, Hopfenmüller W, Vogel M, Roots I. Below median birth weight in appropriate-for-gestational-age preterm infants as a risk factor for bronchopulmonary dysplasia. J Perinat Med 2008;36:359-64. [Crossref] [PubMed]
- McEvoy CT, Jain L, Schmidt B, Abman S, Bancalari E, Aschner JL. Bronchopulmonary dysplasia: NHLBI workshop on the primary prevention of chronic lung diseases. Ann Am Thorac Soc 2014;11 Suppl 3:S146-53. [Crossref] [PubMed]
- Avni EF, Cassart M, de Maertelaer V, Rypens F, Vermeylen D, Gevenois PA. Sonographic prediction of chronic lung disease in the premature undergoing mechanical ventilation. Pediatr Radiol 1996;26:463-9. [Crossref] [PubMed]
- Pieper CH, Smith J, Brand EJ. The value of ultrasound examination of the lungs in predicting bronchopulmonary dysplasia. Pediatr Radiol 2004;34:227-31. [Crossref] [PubMed]
- Peroni DG, Boner AL. Atelectasis: mechanisms, diagnosis and management. Paediatr Respir Rev 2000;1:274-8. [Crossref] [PubMed]
- Whitfield JM, Jones MD Jr. Atelectasis associated with mechanical ventilation for hyaline membrane disease. Crit Care Med 1980;8:729-31. [Crossref] [PubMed]
- Liu J, Chen SW, Liu F, Li QP, Kong XY, Feng ZC. The diagnosis of neonatal pulmonary atelectasis using lung ultrasound. Chest 2015;147:1013-9. [Crossref] [PubMed]
- Acosta CM, Maidana GA, Jacovitti D, Belaunzarán A, Cereceda S, Rae E, Molina A, Gonorazky S, Bohm SH, Tusman G. Accuracy of transthoracic lung ultrasound for diagnosing anesthesia-induced atelectasis in children. Anesthesiology 2014;120:1370-9. [Crossref] [PubMed]
- Apiliogullari B, Sunam GS, Ceran S, Koc H. Evaluation of neonatal pneumothorax. J Int Med Res 2011;39:2436-40. [Crossref] [PubMed]
- Wyatt TH. Pneumothorax in the neonate. J Obstet Gynecol Neonatal Nurs 1995;24:211-6. [Crossref] [PubMed]
- Lichtenstein D, Mezière G, Biderman P, Gepner A. The "lung point": an ultrasound sign specific to pneumothorax. Intensive Care Med 2000;26:1434-40. [Crossref] [PubMed]
- Raimondi F, Rodriguez Fanjul J, Aversa S, Chirico G, Yousef N, De Luca D, Corsini I, Dani C, Grappone L, Orfeo L, Migliaro F, Vallone G, Capasso L. Lung Ultrasound in the Crashing Infant (LUCI) Protocol Study Group. Lung ultrasound for diagnosing pneumothorax in the critically ill neonate. J Pediatr 2016;175:74-78.e1. [Crossref] [PubMed]
- Cattarossi L, Copetti R, Brusa G, Pintaldi S. Lung Ultrasound Diagnostic Accuracy in Neonatal Pneumothorax. Can Respir J 2016;2016. [Crossref] [PubMed]
- Edwards MO, Kotecha SJ, Kotecha S. Respiratory distress of the term newborn infant. Paediatr Respir Rev 2013;14:29-36. [Crossref] [PubMed]
- Lobo L. The neonatal chest. Eur J Radiol 2006;60:152-8. [Crossref] [PubMed]
- Sameshima YT, Lourenço de Almeida JF, Silva MM, Remondini R, Haddad LB, Neto MJ, Buarque de Gusmão Funari M. Ultrasound-guided lung recruitment in a 3-month-old infant with acute respiratory distress syndrome. Ultrasound Q 2014;30:301-5. [Crossref] [PubMed]
- Liu J, Ren XL, Fu W, Liu Y, Xia RM. Bronchoalveolar lavage for the treatment of neonatal pulmonary atelectasis under lung ultrasound monitoring. J Matern Fetal Neonatal Med 2017;30:2362-6. [Crossref] [PubMed]
- Raimondi F, Migliaro F, Sodano A, Ferrara T, Lama S, Vallone G, Capasso L. Use of neonatal chest ultrasound to predict noninvasive ventilation failure. Pediatrics 2014;134:e1089-94. [Crossref] [PubMed]
- Brat R, Yousef N, Klifa R, Reynaud S, Shankar Aguilera S, De Luca D. Lung ultrasonography score to evaluate oxygenation and surfactant need in neonates treated with continuous positive airway pressure. JAMA Pediatr 2015;169. [Crossref] [PubMed]