Survival prediction based on qualitative MRI diffusion signature in patients with recurrent high grade glioma treated with bevacizumab
Introduction
Glioblastoma (GBM) is the most common primary malignant CNS tumor with poor prognosis even after standard treatment of surgery, postoperative temozolomide (TMZ) sensitized radiochemotherapy followed by TMZ monotherapy with a median survival of 14 months (1). At recurrence, patients typically survive only another 30 weeks (2-4). In recurrent or progressive GBM following the standard therapy, several biological therapeutic agents were tested, out of which the angiogenesis inhibitor bevacizumab (Avastin) was approved by the FDA in 2009 (5). There has been improved radiographic response, progression-free survival (6) but inconsistent improvements of overall survival (OS) in patients with recurrent GBM on bevacizumab (7-11). Tumor treatment response is typically assessed by gadolinium enhanced MRI. However, when treatment with anti-VEGF agents such as bevacizumab is utilized there is a common phenomenon of marked decrease in enhancement post treatment known as pseudoresponse. Pseudoresponse relates to block of neovascularization with abnormally permeable microvessels and is mostly noted with antiangiogenic treatment. This leads to a rapid decrease in contrast leakage of gadolinium, but it does not reflect the decrease in tumor activity or size (12). Therefore, the traditional method of evaluating response by evaluating for decrease in enhancing tumor may not be accurate when antiangiogenic treatments utilized, and other methods of evaluation are needed. As a result, Response Assessment for Neuro-Oncology (RANO) response criteria added some new suggestions that include anti-angiogenic therapy (13).
Though, diffusion imaging once gained attraction as an alternative marker to assess treatment response and tumor progression, however in last few years, there has been great interest in applying other multi-parametric MRI sequences in detecting infiltrative tumor and determining treatment response. Dynamic susceptibility weighted magnetic resonance (DSC-MR) perfusion imaging have been shown to be effective at measuring the response to bevacizumab (14-16). Advanced DWI techniques such as graded functional diffusion maps, ADC histogram analysis within both enhancing and non-enhancing components of tumor can be used to predict the response to bevacizumab in recurrent GBMs (17-20). More recently, another advanced DWI technique restriction spectrum imaging (RSI) which along with DSC-MR perfusion study could differentiate Bevacizumab related necrosis from viable tumor (21). However, measurement of all these advanced perfusions and DWI techniques is cumbersome and requires additional software’s and expertize to familiarize with concepts. Moreover, routine diffusion sequences (DWI and ADC maps) are routinely performed as part of every brain study in all clinical and research settings, whereas MR perfusion and advanced DWI techniques are not. Finding early and easy to interpret imaging biomarkers for GBM progression may have a significant impact on clinical treatment decision-making, especially during bevacizumab therapy.
We hypothesized that qualitative focal diffusion restriction, in and around the enhancing tumor, may be a useful predictor of survival. Therefore, our purpose was to determine the utility of qualitative diffusion signature at initial 8 weeks MRI follow-up to assess treatment response to anti-VEGF therapy (bevacizumab) in patients with recurrent GBM. In addition, we sought to assess patient survival following the appearance of restricted diffusion during the course of treatment for recurrent GBM. There have been reports which suggest that progressing bevacizumab-related FDR is associated with coagulative necrosis surrounded by viable tumor and worst OS (22) whereas stable FDR lesions were associated with greatest OS (22,23). However, survival prediction in these studies was based on serial MRI studies. We hereby, hypothesized that even a single qualitative diffusion weighted imaging (DWI) at initial 8 weeks follow-up can predict median OS or effectiveness of bevacizumab in recurrent tumor.
Methods
Patients and follow-up
We performed an IRB approved, retrospective study on patients who underwent MRI of the brain around 8 weeks after starting treatment with bevacizumab for recurrent gliomas. Patients were retrospectively selected from institution’s neuro-oncology database and data acquisition was performed in compliance with all applicable regulations of the Health Insurance Portability and Accountability Act (HIPAA) from December 2009 to March 2015. All patients were treated with maximal gross tumor resection and radiation therapy (60 Gy given over 6 weeks)/temozolomide (TMZ) + adjuvant TMZ at time of initial tumor presentation. A total of n=47 patients who met the following criteria were initially selected: (I) had pathologically confirmed initial high grade gliomas with recurrence based on MRI, clinical data and in some cases with histology; (II) were regularly treated every 2 weeks per cycle with bevacizumab (Avastin, Genentech; 5 or 10 mg/kg body weight) alone or in combination with chemotherapy; (III) had baseline (pre-bevacizumab treatment) and minimum of 1 follow-up MRI scan near 8 weeks post bevacizumab therapy. We excluded patients who received bevacizumab within 3 months of completing radiotherapy, since pseudoprogression typically occurs within the first three months following radiotherapy. Of these recurrent GBM patients, n=39 had good-quality diffusion-weighted images before and after initiation of bevacizumab treatment and were retrospectively assessed to determine if focal diffusion-restricted (FDR) lesions developed in or adjacent to the tumor following treatment onset.
Of these 39 retrospective patients, status of initial tumor’s isocitrate dehydrogenase (IDH-1) mutation and O6-methylguanine DNA methyltransferase (MGMT) promoter methylation (described elsewhere) (24) was available only in 21 patients. An additional analysis was done assessing Karnofsky performance score. Demography of patients is described in Table 1.
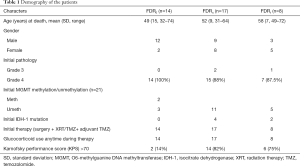
Full table
Patients were divided into three groups based on qualitative diffusion signature: (I) lesion not bright on isotropic diffusion map (DWI) suggestive of no restricted diffusion (FDR0); (II) lesion bright on DWI with corresponding dark signal on apparent diffusion coefficient (ADC) maps suggestive of focal restricted diffusion, likely due to bevacizumab induced necrosis (FDRn) (Figure 1); and (III) lesion bright on DWI with corresponding homogenous faint dark signal on ADC maps suggestive of focal restricted diffusion, likely due to viable tumor or heterogeneous spectrum of dark and faint dark signals on ADC maps suggestive of focal restricted diffusion, likely due to viable tumor surrounding the bevacizumab induced necrosis (FDRt) (Figures 2,3). No patient had clinical signs or symptoms of acute/sub-acute ischemic stroke as an alternative explanation for restricted diffusion, and on MRI dark-ADC lesions were not explained by subacute haemorrhage, ischemia, or postoperative changes.
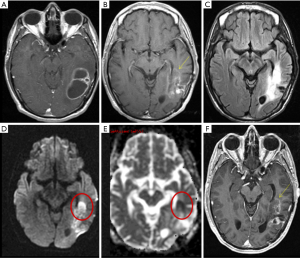
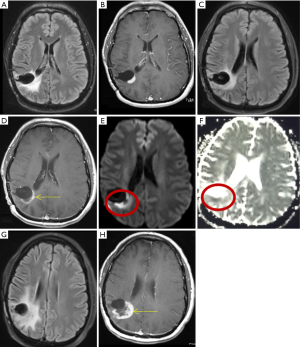
MR imaging
Various MR imaging sequences were obtained on a 1.5/3 T MR scanner (Signa LX Scanner, and DiscoveryTM 750; GE Healthcare, Milwaukee, Wisconsin, USA) with the help of standard 8-channel head coil. All studies were performed according to a standardized protocol that included conventional sequences such as axial T1 FSE, T2FLAIR and T2 FSE as well as DWI, ADC maps, and triplane contrast T1-weighted images. DWI were collected using single-shot echo-planar imaging with TE/TR =100 ms/8,000 ms, FOV =240 mm using a twice refocused spin-echo echo planar preparation, number of excitations (NEX) =1, 5-mm section thickness with 1 mm intersection gap, matrix size =128×128 (reconstructed images were interpolated to 256×256). Post-contrast T1-weighted images in 3 planes were acquired immediately after contrast injection using standard doses of 0.1-mmol/kg gadodiamide (Omniscan; Winthrop Laboratories, Rensselaer, New York, USA).
Image registration
ADC maps were calculated from DWI using commercially available software (FuncTool, GE Healthcare) and the equation ADC =(1/1,000)*ln (b0/b1000). Morphologic and diffusion images were co-registered, allowing for the production of ADC maps.
Image interpretation
Two experienced board certified neuroradiologists (RM and MF) with 8 and 3 years of experience in MRI post-processing, performed ADC analyses (RM holds a Certificate of Added Qualification in Neuroradiology). The DWI and axial contrast-enhanced T1-weighted images were transferred to an off-line workstation and analyzed using available commercial software (Nordic ICE, Nordic Neuro-Lab). ADC maps were calculated from the DWI and low-ADC lesions in and around the tumor area were noted.
Statistical analysis
Differences in OS between the three groups were calculated using Kaplan-Meier Survival curves (Figure 4) and Log-rank t-test via IBM SPSS 22 (Chicago, IL, USA). The time between the date of start of bevacizumab and that of death was defined as the event time. Survival was determined by both documented date of death in the patient’s medical record and by the Social Security Death Index if this information was not available in the medical record. A P value of less than 0.05 was considered to indicate a statistically significant difference.
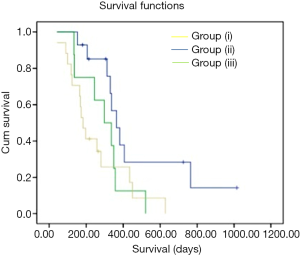
Results
Based on the qualitative diffusion signature manifested by variable degrees of dark signal on ADC maps after bevacizumab therapy, total number of patients with no restriction FDR0 n=14 (36%), with FDRn n=17 (44%); and with FDRt n=8 (20%). For all patients, median OS =313 days (95% CI, 232–393 days) from the time of the first bevacizumab treatment. The median OS from the time of recurrence in patients belonging to FDRn was 364 days (95% CI, 295–432 days) vs. FDR0 183 days (95% CI, 145–220 days) vs. FDRt 298 days (95% CI, 171-424 days) (Table 2). On simultaneous comparison of survival differences in all three groups by Kaplan-Meier analysis, group (II) was significant in predicting survival with P values for the log-rank tests <0.033 (Table 3). Tumor samples from 2 of 14 patients in the no diffusion restriction group (I) were found to be MGMT methylated, whereas 3 of 14 patients of group (I), 11 of 17 patients of group (II) and 5 of 8 patients of group (III) were found to be MGMT un-methylated (Table 1). None of group (I) patients had IDH-1 mutation whereas mutation was present in 4 patients from group (II) and 2 patients from group (III).
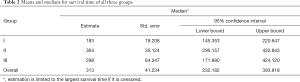
Full table
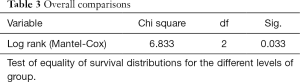
Full table
Discussion
Based on our and others experience, often quantitative measurement of ADC values from the ADC map can be challenging even to experienced personnel due to heterogeneous distribution of nests of tumor cells within the tumor, the presence of tumor necrosis, hemorrhage and ischemia, and may cause significant sampling bias. We tried to explore if morphologic diffusion signature rather than quantitative ADC values can be used to assess tumor progression in recurrent GBM. Because our study was retrospective and our patients didn’t undergo autopsy due to medicolegal and other reasons, we utilized cut-off values of mean ADC reported previously (22) which were determined from autopsy samples of coagulative necrosis and viable tumor.
Our study explored qualitative diffusion signature manifested by variable degrees of dark signal on ADC maps following bevacizumab treatment. Earlier glioma studies have found an inverse correlation between ADC and cellularity tumor grade, and Ki-67 proliferation indices (25,26). Besides increasing cellularity and subsequent decreases in the free extracellular space and water proton diffusivity, low ADC values in GBMs are possibly related to relative tumor ischemia (25,26). However, mean ADC values of hypercellularity of GBM has been reported to be near 0.9×10−3 mm2/s (22) which is closer to mean ADC value of early subacute infarct (27), so should match phenotypically with early subacute infarct (variable degrees of faint dark signal on ADC maps) (Figure 3).
Anti-VEGF therapy associated tumor necrosis has been found to be coagulative necrosis (22), and in contrast to liquefactive necrosis which is associated with radiation necrosis in brain and TACE (trans-arterial chemoembolization)/ablation associated necrosis of hepatocellular carcinoma or other solid tumors (28). Whereas liquefactive necrosis is associated with high diffusion and consequent bright signal on ADC images, coagulative necrosis on the other hand is associated with restricted diffusion with low ADC values (22). There are few theories regarding the mechanism behind diffusion-restriction in coagulative necrosis. Jeyaretna et al., in their case report hypothesized that focal regions of coagulative necrosis may result from exacerbated radiation necrosis by bevacizumab (29). Others hypothesized that such regions result from bevacizumab-induced chronic hypoxia (30). Moreover, lack of hypoxia expression within recurrent high-grade glioma following bevacizumab has also been suggested by prior investigations using 18F Fluoromisonidazole (FMISO) positron emission tomography and MR imaging (31). We believe the reason behind diffusion restriction is bevacizumab induced tumor ischemia. This concept of tumor ischemia is supported by histopathologic findings of perivascular tumor deposits, vasculopathy, and small brain infarcts in bevacizumab-treated patients (32). Mean ADC value in pathologically confirmed bevacizumab coagulative necrosis is reported to be in the range of 0.63×10−3–0.736×10−3 mm2/s (22) which is closer to mean ADC value of acute infarct (0.57±0.16)×10−3 mm2/s (27), so it should match phenotypically with acute infarct (dark on ADC) (Figure 3).
Although there has been a suggestion of role of pre and post treatment quantitative DWI (33,34), qualitative diffusion signatures are not fully explored and not commonly implemented into treatment decision-making trees due to the controversial significance of new restricted diffusion during antiangiogenic treatment in recurrent GBM. There are several studies showing both increased and decreased survival associated with diffusion restriction in patients receiving bevacizumab (12-15,19-21,23,29,30,35-40). However, recent reports suggest that progressing bevacizumab related FDR lesions due to coagulative necrosis surrounded by viable tumor are associated with worst OS (22) whereas stable FDR lesions are associated with greatest OS (22,23). In our study, we found that FDRn group (OS =364 days), which should correspond to coagulative necrosis, was associated with better OS than FDRt group (OS =298 days), which corresponds to the combination of viable tumor and coagulative necrosis. However, contrary to results of Nguyen et al. (22), OS of our patients with no restriction (those who did not develop lesions in first 2 months) in FDR0 group was shorter than FDRt group (183 vs. 298 days). We think poor survival in group (I), despite younger mean age among all three groups, might be due to unfavorable tumor biology, as 100% were grade IV and most of them were probably primary due to the absence of IDH-1 mutation (Table 1). Moreover, Karnofsky performance scores were also poor in group (I) compared to group (II) and (III) patients. Leaving aside the group (I) due to the unbalanced distribution of prognostic factors, our study is of value for all other patients with restricted diffusion during antiangiogenic treatment in recurrent GBM, where the most controversy exists. Survival differences in group (II) and group (III) in our study should be considered significant as the distribution of various other prognostic markers which can influence survival such as age, performance status, tumor biology including MGMT methylation as well IDH-1 mutation status were balanced (Table 1).
Our study is different from other recent studies in which survival prediction or effectiveness of bevacizumab was based on serial MRI studies which can result in the loss of valuable time. Whereas, based on our study we can predict statistically significant OS even on a single MRI follow-up at 8 weeks. GBM progresses with time as evident from natural history, and it is true even for recurrent GBM on combination chemotherapy including anti-VEGF. Lesions which demonstrate FDRn within 2 months eventually will develop viable tumor with longer time duration and will show heterogeneous spectrum of dark and faint dark signals on ADC due to the combination of FDRn and FDRt. So, the importance of morphologic diffusion signature decreases with longer or serial follow-up period.
Our study suggests that on ADC maps, the patients with the homogenous dark signal (like acute infarct phenotype), should have better OS than with patients with homogenous faint dark signal (subacute phenotype) or heterogeneous spectrum of dark and faint dark signals (like both acute and subacute phenotype). Though there is no doubt about the importance of quantitative cut-off ADC value of 0.736×10−3 mm2/s separating diffusion-restricted necrosis and viable hypercellular tumor which is based on autopsy analysis by Nguyen et al. (22), there is limitation of universal application of quantitative ADC values as: (I) there is reported variability in ADC values among and even within vendors (41); (II) also, they measured the ADC values in the region of autopsy confirmed coagulative necrosis and viable tumors, whereas most common method of ADC value measurement is ADCmin, in which out of 4–5 low ADC values, the lowest ADC value is taken into consideration. This method is good for grading of gliomas and should be considered when done for initial evaluation of tumor. However, it can give false negative result in recurrent gliomas with coagulative necrosis surrounded by viable tumor, as there is a possibility that one or all the 4–5 measurements may be taken from coagulative necrosis and lowest ADC value come below cut-off ADC value of 0.736×10−3 mm2/s. Whereas, our method of diffusion signature which manifest as variable degrees of dark signals on ADC maps is though subjective, the lesions in group (II) and group (III) were visibly apparent and clearly abnormal even without any quantitative measurements and could predict statistically significant median OS. On the other hand, we do not undermine the importance of quantitative measurement, but suggest caution while interpreting the ADC values in recurrent gliomas, and recommend if there is heterogeneous spectrum of dark and faint dark signals on ADC maps, ADC values should be taken from most dark and least dark area and instead of considering the lowest of 4–5 ADC values (ADCmin), mean of 4–5 ADC values (ADCmean) should be taken into consideration and value above cut-off is suggestive of viable tumor or worse prognosis. Interpretation of homogenous dark signal is straightforward, however though rarely, there might come difficulty in interpretation of a lesion which demonstrates homogenous intermediate dark signal on ADC maps; in that case also, quantitative ADCmean can be helpful. Moreover, if need arises, morphologic diffusion signature can also be used for tissue sampling in regions of FDRt as this could reduce the likelihood of false-negative results.
There are few limitations in our study; one potential limitation is the relatively small patient cohort. Despite the small numbers, we achieved statistically significant results. However, worst OS in those with no restriction in our study was unexpected, likely reasons behind this, already explained earlier. Further research is necessary to establish the biological basis for bevacizumab causing these lesions. Another potential limitation is the lack of locus-specific histopathologic correlation in our series to confirm the homogenous dark signal (FDRn) lesions as coagulative necrosis rather than viable tumor. However, others have reported histologic proof of restricted diffusion with mean ADC values below 0.736×10−3 mm2/s as coagulative necrosis, which correspond to dark signal on ADC maps. Larger prospective trials are needed to confirm our findings, but our data support the role of using diffusion signature in predicting median OS of recurrent GBM on bevacizumab at early follow-up within 8 weeks. Additionally, our study is limited to the biologic effect of bevacizumab therapy with and without TMZ in recurrent GBM, however due to current practice of different combination adjuvant therapy, biological effects may vary and further research is necessary to establish the effects of different combination adjuvant therapy in recurrent GBM.
Therefore, recognitions of these qualitative diffusion signatures have the widest potential impact on routine clinical care for patients with recurrent GBMs without requiring any additional imaging time or imaging technique beyond standard practice.
Conclusions
DWI is routinely performed as part of every brain study in all research and clinical settings; whereas MR perfusion and other advanced DWI techniques are not universally performed. As everyone is familiar with the appearance of acute and subacute infarct on ADC maps, we tried to use diffusion signature as an easy method to interpret imaging biomarkers for GBM progression. We proposed if lesions demonstrating homogenous dark signal (acute infarct phenotype) on diffusion map will have better survival than both the lesions with a faint dark signal (subacute phenotype) or heterogeneous spectrum of dark and faint dark signals (subacute and acute phenotypes) and the lesions with no restriction. The use of survival prediction in patients with recurrent gliomas may have a significant impact on clinical treatment decision-making, especially as early as 8 weeks of bevacizumab therapy. This prediction of tumor response is especially important given a recent meta-analysis that showed an associated increase in treatment-related mortality for bevacizumab when added to other chemotherapy as compared to chemotherapy alone (6). Therefore, if bevacizumab is to be used as a major treatment, it is essential to identify patients who will respond well to therapy versus those who are unlikely to respond as early in the course of treatment as possible.
Therefore, the recognition of this qualitative diffusion signature in adjunct to contrast enhanced MRI has the widest potential impact on routine clinical care for patients with recurrent GBMs, without requiring any additional imaging time or imaging technique beyond standard practice. Prospective studies analysing its predictive value are warranted.
Acknowledgements
None.
Footnote
Conflicts of Interest: The authors have no conflicts of interest to declare.
Ethical Statement: The study was approved by University of Rochester Medical Center, Rochester, New York, USA.
References
- Stupp R, Mason WP, van den Bent MJ, Weller M, Fisher B, Taphoorn MJ, Belanger K, Brandes AA, Marosi C, Bogdahn U, Curschmann J, Janzer RC, Ludwin SK, Gorlia T, Allgeier A, Lacombe D, Cairncross JG, Eisenhauer E, Mirimanoff RO. European Organisation for Research and Treatment of Cancer Brain Tumor and Radiotherapy Groups. National Cancer Institute of Canada Clinical Trials Group. Radiotherapy plus concomitant and adjuvant temozolomide for glioblastoma. N Engl J Med 2005;352:987-996. [Crossref] [PubMed]
- Iwamoto FM, Abrey LE, Beal K, Gutin PH, Rosenblum MK, Reuter VE, DeAngelis LM, Lassman AB. Patterns of relapse and prognosis after bevacizumab failure in recurrent glioblastoma. Neurology 2009;73:1200-6. [Crossref] [PubMed]
- Wong ET, Hess KR, Gleason MJ, Jaeckle KA, Kyritsis AP, Prados MD, Levin VA, Yung WK. Outcomes and prognostic factors in recurrent glioma patients enrolled onto phase II clinical trials. J Clin Oncol 1999;17:2572-8. [Crossref] [PubMed]
- Lamborn KR, Yung WK, Chang SM, Wen PY, Cloughesy TF, DeAngelis LM, Robins HI, Lieberman FS, Fine HA, Fink KL, Junck L, Abrey L, Gilbert MR, Mehta M, Kuhn JG, Aldape KD, Hibberts J, Peterson PM, Prados MD. North American Brain Tumor Consortium. Progression-free survival: an important end point in evaluating therapy for recurrent high-grade gliomas. Neuro-oncology 2008;10:162-70. [Crossref] [PubMed]
- Cohen MH, Shen YL, Keegan P, Pazdur R. FDA drug approval summary: bevacizumab (Avastin) as treatment of recurrent glioblastoma multiforme. Oncologist 2009;14:1131-8. [Crossref] [PubMed]
- Friedman HS, Prados MD, Wen PY, Mikkelsen T, Schiff D, Abrey LE, Yung WK, Paleologos N, Nicholas MK, Jensen R, Vredenburgh J, Huang J, Zheng M, Cloughesy T. Bevacizumab alone and in combination with irinotecan in recurrent glioblastoma. J Clin Oncol 2009;27:4733-40. [Crossref] [PubMed]
- Lai A, Tran A, Nghiemphu PL, Pope WB, Solis OE, Selch M, Filka E, Yong WH, Mischel PS, Liau LM, Phuphanich S, Black K, Peak S, Green RM, Spier CE, Kolevska T, Polikoff J, Fehrenbacher L, Elashoff R, Cloughesy T. Phase II study of bevacizumab plus temozolomide during and after radiation therapy for patients with newly diagnosed glioblastoma multiforme. J Clin Oncol 2011;29:142-8. [Crossref] [PubMed]
- Kreisl TN, Kim L, Moore K, Duic P, Royce C, Stroud I, Garren N, Mackey M, Butman JA, Camphausen K, Park J, Albert PS, Fine HA. Phase ii trial of single-agent bevacizumab followed by bevacizumab plus irinotecan at tumor progression in recurrent glioblastoma. J Clin Oncol 2009;27:740-5. [Crossref] [PubMed]
- Chamberlain MC. Bevacizumab for the treatment of recurrent glioblastoma. Clin Med Insights Oncol 2011;5:117-29. [Crossref] [PubMed]
- Vredenburgh JJ, Desjardins A, Herndon JE 2nd, Dowell JM, Reardon DA, Quinn JA, Rich JN, Sathornsumetee S, Gururangan S, Wagner M, Bigner DD, Friedman AH, Friedman HS. phase ii trial of bevacizumab and irinotecan in recurrent malignant glioma. Clin Cancer Res 2007;13:1253-9. [Crossref] [PubMed]
- Vredenburgh JJ, Desjardins A, Herndon JE 2nd, Marcello J, Reardon DA, Quinn JA, Rich JN, Sathornsumetee S, Gururangan S, Sampson J, Wagner M, Bailey L, Bigner DD, Friedman AH, Friedman HS. Bevacizumab plus irinotecan in recurrent glioblastoma multiforme. J Clin Oncol 2007;25:4722-9. [Crossref] [PubMed]
- de Groot JF, Fuller G, Kumar AJ, Piao Y, Eterovic K, Ji Y, Conrad CA. Tumor invasion after treatment of glioblastoma with bevacizumab: radiographic and pathologic correlation in humans and mice. Neuro Oncol 2010;12:233-42. [Crossref] [PubMed]
- Wen PY, Macdonald DR, Reardon DA, Cloughesy TF, Sorensen AG, Galanis E, Degroot J, Wick W, Gilbert MR, Lassman AB, Tsien C, Mikkelsen T, Wong ET, Chamberlain MC, Stupp R, Lamborn KR, Vogelbaum MA, van den Bent MJ, Chang SM. Updated response assessment criteria for high-grade gliomas: response assessment in neuro-oncology working group. J Clin Oncol 2010;28:1963-72. [Crossref] [PubMed]
- LaViolette PS, Cohen AD, Prah MA, Rand SD, Connelly J, Malkin MG, Mueller WM, Schmainda KM. Vascular change measured with independent component analysis of dynamic susceptibility contrast MRI predicts bevacizumab response in high-grade glioma. Neuro Oncol 2013;15:442-50. [Crossref] [PubMed]
- Schmainda KM, Prah M, Connelly J, Rand SD, Hoffman RG, Mueller W, Malkin MG. Dynamic-susceptibility contrast agent MRI measures of relative cerebral blood volume predict response to bevacizumab in recurrent high-grade glioma. Neuro Oncol 2014;16:880-8. [Crossref] [PubMed]
- Sawlani RN, Raizer J, Horowitz SW, Shin W, Grimm SA, Chandler JP, Levy R, Getch C, Carroll TJ. Glioblastoma: a method for predicting response to antiangiogenic chemotherapy by using MR perfusion imaging--pilot study. Radiology 2010;255:622-8. [Crossref] [PubMed]
- Ellingson BM, Cloughesy TF, Lai A, Mischel PS, Nghiemphu PL, Lalezari S, Schmainda KM, Pope WB. Graded functional diffusion map- defined characteristics of apparent diffusion coefficients predict overall survival in recurrent glioblastoma treated with bevacizumab. Neuro Oncol 2011;13:1151-61. [Crossref] [PubMed]
- Ellingson BM, Kim E, Woodworth DC, Marques H, Boxerman JL, Safriel Y, McKinstry RC, Bokstein F, Jain R, Chi TL, Sorensen AG, Gilbert MR, Barboriak DP. Diffusion MRI quality control and functional diffusion map results in ACRIN 6677/RTOG 0625: a multicenter, randomized, phase II trial of bevacizumab and chemotherapy in recurrent glioblastoma. Int J Oncol 2015;46:1883-92. [Crossref] [PubMed]
- Rahman R, Hamdan A, Zweifler R, Jiang H, Norden AD, Reardon DA, Mukundan S, Wen PY, Huang RY. Histogram analysis of apparent diffusion coefficient within enhancing and nonenhancing tumor volumes in recurrent glioblastoma patients treated with bevacizumab. J Neurooncol 2014;119:149-58. [Crossref] [PubMed]
- Pope WB, Kim HJ, Huo J, Alger J, Brown MS, Gjertson D, Sai V, Young JR, Tekchandani L, Cloughesy T, Mischel PS, Lai A, Nghiemphu P, Rahmanuddin S, Goldin J. Recurrent glioblastoma multiforme: ADC histogram analysis predicts response to bevacizumab treatment. Radiology 2009;252:182-9. [Crossref] [PubMed]
- Farid N, Almeida-Freitas DB, White NS, McDonald CR, Muller KA, Vandenberg SR, Kesari S, Dale AM. Restriction-Spectrum Imaging of bevacizumab-related necrosis in a patient with GBM. Front Oncol 2013;3:258. [Crossref] [PubMed]
- Nguyen HS, Milbach N, Hurrell SL, Cochran E, Connelly J, Bovi JA, Schultz CJ, Mueller WM, Rand SD, Schmainda KM, LaViolette PS. Progressing Bevacizumab-induced diffusion restriction is associated with coagulative necrosis surrounded by viable tumor and decreased overall survival in patients with recurrent glioblastoma. AJNR Am J Neuroradiol 2016;37:2201-8. [Crossref] [PubMed]
- Mong S, Ellingson BM, Nghiemphu PL, Kim HJ, Mirsadraei L, Lai A, Yong W, Zaw TM, Cloughesy TF, Pope WB. Persistent diffusion-restricted lesions in bevacizumab-treated malignant gliomas are associated with improved survival compared with matched controls. AJNR Am J Neuroradiol 2012;33:1763-70. [Crossref] [PubMed]
- Molenaar RJ, Verbaan D, Lamba S, Zanon C, Jeuken JW, Boots-Sprenger SH, Wesseling P, Hulsebos TJ, Troost D, Van Tilborg AA, Leenstra S. The combination of IDH1 mutations and MGMT methylation status predicts survival in glioblastoma better than either IDH1 or MGMT alone. Neuro-oncology 2014;16:1263-73. [Crossref] [PubMed]
- Gupta A, Young RJ, Karimi S, Sood S, Zhang Z, Mo Q, Gutin PH, Holodny AI, Lassman AB. Isolated diffusion restriction precedes the development of enhancing tumor in a subset of patients with glioblastoma. AJNR Am J Neuroradiol 2011;32:1301-6. [Crossref] [PubMed]
- Alvarez-Linera J, Benito-Leon J, Escribano J, Ray G. Predicting the histopatho-logical grade of cerebral gliomas using high b value MR DW imaging at 3-Tesla. J Neuroimaging 2008;18:276-81. [Crossref] [PubMed]
- Yamada N, Satoshi I, Sakuma T. Value of diffusion-weighted imaging and apparent diffusion coefficient in recent cerebral infarctions: a correlative study with contrast-enhanced T1-weigthed imaging. Am J Neuroradiol 1999;20:193-8. [PubMed]
- Yuan YH, Xiao EH, Liu JB, He Z, Jin K, Ma C, Xiang J, Xiao JH, Chen WJ. Characteristics and pathological mechanism on magnetic resonance diffusion-weighted imaging after chemoembolization in rabbit liver VX-2 tumor model. World J Gastroenterol 2007;13:5699-706. [Crossref] [PubMed]
- Jeyaretna DS, Curry WT Jr, Batchelor TT, Stemmer-Rachamimov A, Plotkin SR. Exacerbation of cerebral radiation necrosis by bevacizumab. J Clin Oncol 2011;29:e159-62. [Crossref] [PubMed]
- Rieger J, Bahr O, Muller K, Franz K, Steinbach J, Hattingen E. Bevacizumab-induced diffusion-restricted lesions in malignant glioma patients. J Neurooncol 2010;99:49-56. [Crossref] [PubMed]
- Barajas RF Jr, Pampaloni MH, Clarke JL, Seo Y, Savic D, Hawkins RA, Behr SC, Chang SM, Berger M, Dillon WP, Cha S. Assessing biological response to bevacizumab using 18F-Fluoromisonidazole PET/MR imaging in a patient with recurrent anaplastic astrocytoma. Case Rep Radiol 2015;2015:731361. [PubMed]
- Kleinschmidt-DeMasters BK, Damek DM. The imaging and neuropathological effects of bevacizumab (Avastin) in patients with leptomeningeal carcinoma-tosis. J Neurooncol 2010;96:375-84. [Crossref] [PubMed]
- Saksena S, Jain R, Narang J, Scarpace L, Schultz LR, Lehman NL, Hearshen D, Patel SC, Mikkelsen T. Predicting survival in glioblastomas using diffusion tensor imaging metrics. J Magn Reson Imaging 2010;32:788-95. [Crossref] [PubMed]
- Murakami R, Sugahara T, Nakamura H, Hirai T, Kitajima M, Hayashida Y, Baba Y, Oya N, Kuratsu JI, Yamashita Y. Malignant supratentorial astrocytoma treated with postoperative radiation therapy: prognostic value of pre-treatment quantitative diffusion-weighted MR imaging. Radiology 2007;243:493-99. [Crossref] [PubMed]
- Chinot OL, Wick W, Mason W, Henriksson R, Saran F, Nishikawa R, Carpentier AF, Hoang-Xuan K, Kavan P, Cernea D, Brandes AA, Hilton M, Abrey L, Cloughesy T. Bevacizumab plus radiotherapy temozolomide for newly diagnosed glioblastoma. N Engl J Med 2014;370:709-722. [Crossref] [PubMed]
- Gilbert MR, Dignam JJ, Armstrong TS, Wefel JS, Blumenthal DT, Vogelbaum MA, Colman H, Chakravarti A, Pugh S, Won M, Jeraj R, Brown PD, Jaeckle KA, Schiff D, Stieber VW, Brachman DG, Werner-Wasik M, Tremont-Lukats IW, Sulman EP, Aldape KD, Curran WJ Jr, Mehta MP. A randomized trial of bevacizumab for newly diagnosed glioblastoma. N Engl J Med 2014;370:699-708. [Crossref] [PubMed]
- Gerstner ER, Frosch MP, Batchelor TT. Diffusion magnetic resonance imaging detects pathologically confirmed, nonenhancing tumor progression in a patient with recurrent glioblastoma receiving bevacizumab. J Clin Oncol 2010;28:e91-3. [Crossref] [PubMed]
- Paldino MJ, Desjardins A, Friedman HS, Vredenburgh JJ, Barboriak DP. A change in the apparent diffusion coefficient after treatment with bevacizumab is associated with decreased survival in patients with recurrent glioblastoma multiforme. Br J Radiol 2012;85:382-9. [Crossref] [PubMed]
- Bähr O, Harter P, Weise L, You SJ, Mittelbronn M, Ronellenfitsch MW, Rieger J, Steinbach JP, Hattingen E. Sustained focal antitumor activity of bevacizumab in recurrent glioblastoma. Neurology 2014;83:227-34. [Crossref] [PubMed]
- Zhang M, Gulotta B, Thomas A, Kaley T, Karimi S, Gavrilovic I, Woo KM, Zhang Z, Arevalo-Perez J, Holodny AI, Rosenblum M, Young RJ. Large-volume low lesion predicts poor survival in bevacizumab-treated glioblastoma patients. Neuro Oncol 2016;18:735-43. [Crossref] [PubMed]
- Sasaki M, Yamada K, Watanabe Y, Matsui M, Ida M, Fujiwara S, Shibata E. Variability in absolute apparent diffusion coefficient values across different platforms may be substantial: a multi-vendor, multi-institutional comparison study. Radiology 2008;249:624-30. [Crossref] [PubMed]