Intraoperative ultrasound in spine surgery: history, current applications, future developments
Introduction
Precise identification of anatomical landmarks and their variants, localization and delineation of the extent of lesions, and anticipation of surgical maneuvers to optimize surgical access and enhance dexterity are critical in the planning and execution of any surgical procedure. In spine surgery, these concepts are considered alongside four basic principles, defined by AOSpine, which can be universally applied to develop diagnostic and treatment strategies for different pathologies; these principles are: stability, alignment, function, and biology (1). Accordingly, the evolution of spine surgery has been accompanied by the development and adoption of numerous technological aids meant to allow surgeons to preserve and/or restore mechanical stability, alignment, integrity of neural tissues, and functionality. The objectives of implementing the use of such technological aids are multifold: on one hand, they allow for minimally invasive access to respect the biology and function of the surrounding tissues; on the other hand, they make possible a safe maximal resection of spinal cord lesions by favoring a better understanding the nature of lesion and its relationship with normal neural structures. Neuronavigation systems have been among the most prominent and successful of these technological tools, which rely on images acquired preoperatively or intraoperatively to guide the surgical team step by step along difficult procedures (2). Many imaging modalities can be employed to acquire baseline images; the most commonly used are computed tomography (CT) and magnetic resonance imaging (MRI), but more recently other modalities are also being employed, from preoperatively acquired metabolic imaging, as in the case of positron emission tomography (PET), to intraoperatively acquired ultrasound (IoUS) and immunofluorescence, based on 5-aminolevulinic acid or indocyanine green (3-7).
Since its inception, IoUS has evolved as a standalone option for real time image guided surgery and is now becoming more interconnected with other technological aids meant to ensure greater safety and accuracy. Herein we review the historical timeline of the diffusion of IoUS in spine surgery, aiming to better understand its utility in various applications, and describe the multitude of opportunities and challenges that it faces moving forward.
Diffusion of IoUS: historical timeline
The first attempts to introduce ultrasound in neurosurgery date back to 1951, but this diagnostic modality failed to become an established tool for the imaging of the brain and spinal cord over the following decades (8). Several issues, including anatomical constraints (due to the fact that high frequency ultrasound does not readily penetrate the skull and spinal laminae), and technical aspects (since the A-mode scanning available then presented problems of interpretation), prevented widespread application of this diagnostic tool to pathologies affecting the central nervous system (9). In a way, this represents a paradox in the history of medical ultrasonography, which rapidly pervaded the diagnostic approach to pathological conditions in many other medical specialties during the second half of last century (10).
Neurosurgical applications of IoUS, mostly related to brain space occupying lesions, were widely reported in the 1980s; the widespread interest toward IoUS was due in part to the possibility of demonstrating various aspects of solido-cystic lesions, including: septated fluid collections, areas of thick septations, nodularity and solid components. In 1978, Reid exploited the natural opening of the interlaminar window in a flexed neck and employed focused ultrasound to preoperatively evaluate the spinal cord in a patient with cystic astrocytoma: his experimental approach confirmed that the penetration and beam collimation of a 4.0-MHz transducer could well match the width of the interlaminar spaces (11). In the following years spine surgeons started publishing few reports regarding the intraoperative use of this imaging modality: Dohrmann and Rubin authored one of the first articles on this technique, describing a dynamic intraoperative imaging of the spinal cord using a real-time ultrasound scanner (12). Their approach represents a cornerstone for today’s IoUS in spine surgery: in fact, they were the first to describe the possibility to “explore” the intradural space and with a 7.5-MHz transducer visualize the normal spinal cord, including the central canal and the dentate ligaments before opening the dura mater. The IoUS systems available at the time already allowed to visualize the anterior and posterior spinal arteries, define the fluid-filled cavities in patients with syringomyelia and assist the surgeons in draining and shunting them. Quencer and Montalvo, published an illustrative report on their observations concerning the normal spinal cord, its highly reflective dorsal and ventral surfaces, its uniform hypoechogenicity, and the presence of a central echo (13). The accurate depiction of normal intraoperative spine sonography and the possibility to use this knowledge to interpret many pathological conditions was deemed important for surgical guidance. Hence, those initial studies warranted further attempts to expand the use of IoUS initially to spinal cord cysts or cystic components of tumors, and later on to many other groups of intradural extramedullary tumors as well as those with both extradural and intradural components. In the early 1990s, Mimatsu et al. stressed the importance of using IoUS in ventrally located lesions, including meningiomas, neurilemmomas and dermoid cysts (14); whereas Kawakami et al. exploited IoUS to predict the degree of parenchymal infiltration in pediatric patients operated for spinal cord astrocytomas and ependymomas (15).
Thanks to advances in the sophistication of ultrasound techniques, Avila et al. and Kataoka et al. brought IoUS to the next level by introducing the use of color Doppler flow images, which provided improved delineation of all lesions compared with the images produced with standard gray-scale IoUS (16,17). This expanded the range of application to vascular lesions such as hemangioblastomas, spinal dural arteriovenous fistulas, etc. The experience with IoUS of the spinal cord matured over the last two decades and is currently an established intraoperative companion for any experienced spinal surgeon.
Current applications in spine surgery
A number of modern devices for IoUS are currently on the market, those mobile units with dedicated transducers can easily serve in different surgical scenarios for both brain and spine surgery. With regards to IoUS for spinal surgery, the most appropriate transducer probes have usually a 20-mm or smaller diameter and a frequency range from 4 to 10 MHz, and allow to obtain images in both transverse and longitudinal planes. The scan probe is usually covered with sterile disposable plastic bags, and introduced in the surgical site following removal of the laminae and exposure of the dura. The surgical field should be filled with saline solution for acoustic coupling, and the probe does not need to be in contact with the dura or the spinal cord to obtain images.
The normal structure of the spinal canal that can be appreciated include the dura-arachnoid layer, subarachnoid space, denticulate ligament, dorsal arachnoid septations, ventral and dorsal roots, including those pertaining to the cauda equina. In addition to the clear definition of normal structures and pathologic lesions, IoUS allows a clear definition of iatrogenically introduced material including hemostatic agents, cottonoid pledgets, suture material, etc. The current literature on IoUS confirms that this methodology is safe and easy to use; it requires a short learning curve and can be used in many clinical scenarios (see Table 1 for a list of landmark papers supporting the multifold use of IoUS in today’s spinal surgery).
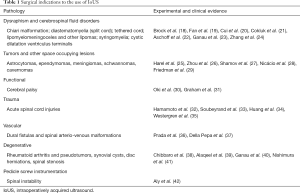
Full table
Many authors have suggested that IoUS can ensure a satisfactory working corridor while reducing: (I) the incision dimension, (II) the need for intraoperative fluoroscopy, and (III) the number of laminectomies required to expose an intradural or discal pathology (25). Based on our personal surgical experience, we agree on all the above and advocate the use of IoUS not only for identification of the spinal pathologies operated, but also to confirm the degree of decompression of the neural structures (Figure 1), and guide during screw insertion in instrumented spinal surgery (42,43).
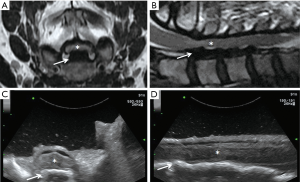
Challenges and future opportunities
IoUS is now widely available and relatively inexpensive compared to other imaging modalities, but it suffers from major limitations such as the lack of orientation and panoramic view; to overcome these shortcomings, fusion of preoperative MRI and IoUS images has been proposed (44). In fact, the intrinsically high resolution of IoUS, associated with complementary imaging technologies, could provide a powerful means of quantitatively monitoring changes in anatomy, structure, physiology and function of the living cord over time after onset of disease, or therapeutic intervention (45). Protocols for fusion of IoUS images are the same used for intraoperative acquisition of CT images and their fusion on preoperative MRI: in both cases the imaging modality adopted intraoperatively serves to recalibrate the navigated MRI imaging, and by continuously updating the two modalities to adjust for artifacts and distortions due to technical issues or anatomic structures (i.e., adjacent bone, hemosiderin, etc.) (46,47). Whereas integrated systems already allow for a true real-time feedback during surgery, the possibility to optimize the imaging definition with the use of innovative contrast agents could move forward the state of the art of IoUS. Microbubbles and nanoparticles contrast agents (CA) have been recently proposed as the latest innovation for ultrasound imaging: following endovenous administration they are free to circulate in the blood stream and, because of their micrometer or nanometer size and the binding ligands on their surfaces, can bypass vascular endothelial cells and accumulate at tissue sites that overexpress those molecular targets (48-51).
Of note, the fragility of spinal cord compared to brain parenchyma has represented the main limitation to the use of ultrasound elastography in spinal cord surgery. Elastography measures tissue strain, which can be interpreted under certain simplifying assumptions to be representative of the underlying stiffness distribution: this appears particularly useful in the surgical approach to tumors which tend to have a different stiffness to healthy tissue, and has also shown potential to provide indication of the degree of bonding at tumor-tissue boundaries, which is clinically useful because of its dependence on tumor pathology grade and aggressiveness (52). The external compression to the tissue studied by quasistatic elastography made this kind of investigation not suitable for spinal cord lesions. Nonetheless the introduction of related techniques, based on acoustic radiation force impulse imaging and shear-wave elasticity imaging, allowed to overcome this limitation and to reconstruct 2-D and 3-D stiffness maps of a given tissue (53). Those new concepts have been recently extended also to the field of neurosurgery; for instance Uff et al. demonstrated that elastograms can be successfully generated in the spinal cord using vascular pulsations to generate internal strains: their results revealed a correlation between strain data and the surgeon’s assessment of the stiffness of the tissues; furthermore they found that areas of reduction in cross-correlation coefficient and very high axial strain at tumor boundaries predicted cleavage planes (54). Hopefully fine tuning of the methodologies for ultrasound elastography will bring additional benefits to IoUS systems: this will enhance our ability to predict the stiffness of spinal cord lesions and identify their dissection planes, both of which will eventually lead to safer surgery especially in case of small lesions such as intramedullary cavernomas.
Conclusions
The optimization of neuronavigation systems and the introduction of IoUS for spine surgery have been fostered by numerous advancements in several scientific fields (including: biomedical engineering, imaging, electronics, nanotechnology, etc.). Those technological aids serve now as an excellent tool for surgical planning and help spine surgeons to preserve vital structures encountered intraoperatively. Being a real time imaging modality, IoUS has the advantage over other preoperative imaging modalities, to take into account intraoperative changes, therefore it appears to be a reliable tool for image-guided spine surgery for intradural pathologies and to localize and visualize non-intradural pathologies. As such, many spine surgeons all over the world are now utilizing IoUS in their practice, this trend should foster the incorporation of IoUS in spinal training and fellowship programs; in fact, the use of IoUS is likely to further increase in the coming decade when the use of ultrasonic contrast agents will further enhance the definition of images acquired intraoperatively.
Acknowledgements
None.
Footnote
Conflicts of Interest: The authors have no conflicts of interest to declare.
References
- Fehlings MG, Ahuja CS, Mroz T, Hsu W, Harrop J. Future Advances in Spine Surgery: The AOSpine North America Perspective. Neurosurgery 2017;80:S1-8. [Crossref] [PubMed]
- Härtl R, Lam KS, Wang J, Korge A, Kandziora F, Audigé L. Worldwide survey on the use of navigation in spine surgery. World Neurosurg 2013;79:162-72. [Crossref] [PubMed]
- Muroi C, Fandino J, Coluccia D, Berkmann S, Fathi AR, Landolt H. 5-Aminolevulinic acid fluorescence-guided surgery for spinal meningioma. World Neurosurg 2013;80:223.e1-3. [Crossref] [PubMed]
- De la Garza-Ramos R, Bydon M, Macki M, Huang J, Tamargo RJ, Bydon A. Fluorescent techniques in spinesurgery. Neurol Res 2014;36:928-38. [Crossref] [PubMed]
- Ganau L, Prisco L, Ligarotti GKI, Ambu R, Ganau M. Understanding the Pathological Basis of Neurological Diseases Through Diagnostic Platforms Based on Innovations in Biomedical Engineering: New Concepts and Theranostics Perspectives. Medicines (Basel) 2018;5:E22. [Crossref] [PubMed]
- Farooq H, Genis H, Alarcon J, Vuong B, Jivraj J, Yang VX, Cohen-Adad J, Fehlings MG, Cadotte DW. High-resolution imaging of the central nervous system: how novel imaging methods combined with navigation strategies will advance patient care. Prog Brain Res 2015;218:55-78. [Crossref] [PubMed]
- Grech-Sollars M, Vaqas B, Thompson G, Barwick T, Honeyfield L, O'Neill K, Waldman AD. An MRS- and PET-guided biopsy tool for intraoperative neuronavigational systems. J Neurosurg 2017;127:812-8. [Crossref] [PubMed]
- French LA, Wild JJ, Neal D. The experimental application of ultrasonics to the localization of brain tumors; preliminary report. J Neurosurg 1951;8:198-203. [Crossref] [PubMed]
- Machi J, Sigel B, Jafar JJ, Menoni R, Beitler JC, Bernstein RA, Crowell RM, Ramos JR, Spigos DG. Criteria for using imaging ultrasound during brain and spinal cord surgery. J Ultrasound Med 1984;3:155-61. [Crossref] [PubMed]
- Kane RA. Intraoperative ultrasonography: history, current state of the art, and future directions. J Ultrasound Med 2004;23:1407-20. [Crossref] [PubMed]
- Reid MH. Ultrasonic visualization of a cervical cord cystic astrocytoma. AJR Am J Roentgenol 1978;131:907-8. [Crossref] [PubMed]
- Dohrmann GJ, Rubin JM. Intraoperative ultrasound imaging of the spinal cord: syringomyelia, cysts, and tumors--a preliminary report. Surg Neurol 1982;18:395-9. [Crossref] [PubMed]
- Quencer RM, Montalvo BM. Normal intraoperative spinal sonography. AJR Am J Roentgenol 1984;143:1301-5. [Crossref] [PubMed]
- Mimatsu K, Kawakami N, Kato F, Saito H, Sato K. Intraoperative ultrasonography of extramedullary spinal tumours. Neuroradiology 1992;34:440-3. [Crossref] [PubMed]
- Kawakami N, Mimatsu K, Kato F. Intraoperative sonography of intramedullary spinal cord tumours. Neuroradiology 1992;34:436-9. [Crossref] [PubMed]
- Avila NA, Shawker TH, Choyke PL, Oldfield EH. Cerebellar and spinal hemangioblastomas: evaluation with intraoperative gray-scale color Doppler flow US. Radiology 1993;188:143-7. [Crossref] [PubMed]
- Kataoka H, Miyamoto S, Nagata I, Ueno Y, Hashimoto N. Intraoperative microdoppler monitoring for spinal dural arteriovenous fistulae. Surg Neurol 1999;52:466-72. [Crossref] [PubMed]
- Brock RS, Taricco MA, de Oliveira MF, de Lima Oliveira M, Teixeira MJ, Bor-Seng-Shu E. Intraoperative Ultrasonography for Definition of Less Invasive Surgical Technique in Patients with Chiari Type I Malformation. World Neurosurg 2017;101:466-75. [Crossref] [PubMed]
- Fan T, Zhao H, Zhao X, Liang C, Wang Y, Gai Q. Surgical management of Chiari I malformation based on different cerebrospinal fluid flow patterns at the cranial-vertebral junction. Neurosurg Rev 2017;40:663-670. [Crossref] [PubMed]
- Cui LG, Jiang L, Zhang HB, Liu B, Wang JR, Jia JW, Chen W. Monitoring of cerebrospinal fluid flow by intraoperative ultrasound in patients with Chiari I malformation. Clin Neurol Neurosurg 2011;113:173-6. [Crossref] [PubMed]
- Cokluk C, Jobe KW, Senel A, Iyigun O, Aydin K. Use of intraoperative ultrasonography during the surgical repair of pediatric cystic spinal dysraphism-technical note. Neurol Med Chir (Tokyo) 2004;44:502-5. [Crossref] [PubMed]
- Aschoff A, Albert F, Mende U, Kunze S. Intra-operative sonography in syringomyelia-technique, results, limitations. Acta Neurochir (Wien) 1993;123:176-7. [PubMed]
- Ganau M, Talacchi A, Cecchi PC, Ghimenton C, Gerosa M, Faccioli F. Cystic dilation of the ventriculus terminalis. J Neurosurg Spine 2012;17:86-92. [Crossref] [PubMed]
- Zhang D, Papavassiliou E. Spinal Intradural Arachnoid Webs Causing Spinal Cord Compression with Inconclusive Preoperative Imaging: A Report of 3 Cases and a Review of the Literature. World Neurosurg 2017;99:251-8. [Crossref] [PubMed]
- Harel R, Knoller N. Intraoperative spine ultrasound: application and benefits. Eur Spine J 2016;25:865-9. [Crossref] [PubMed]
- Zhou H, Miller D, Schulte DM, Benes L, Bozinov O, Sure U, Bertalanffy H. Intraoperative ultrasound assistance in treatment of intradural spinal tumours. Clin Neurol Neurosurg 2011;113:531-7. [Crossref] [PubMed]
- Shamov T, Eftimov T, Kaprelyan A, Enchev Y. Ultrasound-based neuronavigation and spinal cord tumour surgery - marriage of convenience or notified incompatibility? Turk Neurosurg 2013;23:329-35. [PubMed]
- Nicácio JM, Rodrigues JC, Galles MH, Faquini IV, de Brito Pereira CA, Ganau M. Cervical intramedullary schwannoma: a case report and review of the literature. Rare Tumors 2009;1:e44. [Crossref] [PubMed]
- Friedman JA, Wetjen NM, Atkinson JL. Utility of intraoperative ultrasound for tumors of the caudaequina. Spine (Phila Pa 1976) 2003;28:288-90; discussion 291. [Crossref] [PubMed]
- Oki A, Oberg W, Siebert B, Plante D, Walker ML, Gooch JL. Selective dorsal rhizotomy in children with spastic hemiparesis. J Neurosurg Pediatr 2010;6:353-8. [Crossref] [PubMed]
- Graham D, Aquilina K, Mankad K, Wimalasundera N. Selective dorsal rhizotomy: current state of practice and the role of imaging. Quant Imaging Med Surg 2018;8:209-18. [Crossref] [PubMed]
- Hamamoto Y, Ogata T, Morino T, Hino M, Yamamoto H. Real-time direct measurement of spinal cord blood flow at the site of compression: relationship between blood flow recovery and motor deficiency in spinal cord injury. Spine (Phila Pa 1976) 2007;32:1955-62. [Crossref] [PubMed]
- Soubeyrand M, Badner A, Vawda R, Chung YS, Fehlings MG. Very high resolution ultrasound imaging for real-time quantitative visualization of vascular disruption after spinal cord injury. J Neurotrauma 2014;31:1767-75. [Crossref] [PubMed]
- Huang L, Chen K, Chen FC, Shen HY, Ye JC, Cai ZP, Lin X. Intraoperative contrast-enhanced ultrasonography for microcirculatory evaluation in rhesus monkey with spinal cord injury. Oncotarget 2017;8:40756-64. [PubMed]
- Westergren H, Farooque M, Olsson Y, Holtz A. Spinal cord blood flow changes following systemic hypothermia and spinal cord compression injury: an experimental study in the rat using Laser-Doppler flowmetry. Spinal Cord 2001;39:74-84. [Crossref] [PubMed]
- Prada F, Del Bene M, Faragò G, DiMeco F. Spinal Dural Arteriovenous Fistula: Is There a Role for Intraoperative Contrast-Enhanced Ultrasound? World Neurosurg 2017;100:712.e15-712.e18. [Crossref] [PubMed]
- Della Pepa GM, Sabatino G, Sturiale CL, Marchese E, Puca A, Olivi A, Albanese A. Association of real-time intraoperative contrast enhanced ultrasound (CEUS) and color-Doppler ultrasound in the surgical treatment of spinal cord dural arteriovenous fistulae. A technical note. World Neurosurg 2018;112:138-42. [Crossref] [PubMed]
- Chibbaro S, Cebula H, Aldea S, Baussart B, Tigan L, Todeschi J, Romano A, Ganau M, Debry C, Servadei F, Proust F, Gaillard S. Endonasal Endoscopic Odontoidectomy in Ventral Diseases of the Craniocervical Junction: Results of a Multicenter Experience. World Neurosurg 2017;106:382-93. [Crossref] [PubMed]
- Alaqeel A, Abou Al-Shaar H, Alaqeel A, Al-Habib A. The utility of ultrasound for surgical spinal decompression. Med Ultrason 2015;17:211-8. [Crossref] [PubMed]
- Ganau M, Ennas F, Bellisano G, Ganau L, Ambu R, Faa G, Maleci A. Synovial cysts of the lumbar spine--pathological considerations and surgical strategy. Neurol Med Chir (Tokyo) 2013;53:95-102. [Crossref] [PubMed]
- Nishimura Y, Thani NB, Tochigi S, Ahn H, Ginsberg HJ. Thoracic discectomy by posterior pedicle-sparing, transfacet approach with real-time intraoperative ultrasonography: Clinical article. J Neurosurg Spine 2014;21:568-76. [Crossref] [PubMed]
- Aly AH, Ginsberg HJ, Cobbold RS. On ultrasound imaging for guided screw insertion in spinal fusion surgery. Ultrasound Med Biol 2011;37:651-64. [Crossref] [PubMed]
- Ganau M, Holly LT, Mizuno J, Fehlings MG. Future Directions and New Technologies for the Management of Degenerative Cervical Myelopathy. Neurosurg Clin N Am 2018;29:185-93. [Crossref] [PubMed]
- Liu TJ, Shen F, Zhang C, Huang PT, Zhu YJ. Real-time ultrasound-MRI fusion image virtual navigation for locating intraspinal tumour in a pregnant woman. Eur Spine J 2018. Epub ahead of print. [Crossref] [PubMed]
- Figley SA, Chen Y, Maeda A, Conroy L, McMullen JD, Silver JI, Stapleton S, Vitkin A, Lindsay P, Burrell K, Zadeh G, Fehlings MG, DaCosta RS. A spinal cord window chamber model for in vivo longitudinal multimodal optical and acoustic imaging in a murine model. PLoS One 2013;8:e58081. [Crossref] [PubMed]
- Miller JA, Fabiano AJ. Comparison of Operative Time with Conventional Fluoroscopy Versus Spinal Neuronavigation in Instrumented Spinal Tumor Surgery. World Neurosurg 2017;105:412-9. [Crossref] [PubMed]
- D'Andrea K, Dreyer J, Fahim DK. Utility of Preoperative Magnetic Resonance Imaging Coregistered with Intraoperative Computed Tomographic Scan for the Resection of Complex Tumors of the Spine. World Neurosurg 2015;84:1804-15. [Crossref] [PubMed]
- Kaneko OF, Willmann JK. Ultrasound for molecular imaging and therapy in cancer. Quant Imaging Med Surg 2012;2:87-97. [PubMed]
- Ganau M. Tackling gliomas with nanoformulated antineoplastic drugs: suitability of hyaluronic acid nanoparticles. Clin Transl Oncol 2014;16:220-3. [Crossref] [PubMed]
- Ganau M, Syrmos NC, D'Arco F, Ganau L, Chibbaro S, Prisco L, Ligarotti GKI, Ambu R, Soddu A. Enhancing contrast agents and radiotracers performance through hyaluronic acid-coating in neuroradiology and nuclear medicine. Hell J Nucl Med 2017;20:166-8. [PubMed]
- Lee JH, Park G, Hong GH, Choi J, Choi HS. Design considerations for targeted optical contrast agents. Quant Imaging Med Surg 2012;2:266-73. [PubMed]
- Uff C, Garcia L, Fromageau J, Dorward N, Bamber JC. Real-time ultrasound elastography in neurosurgery. Rome: IEEE Xplore International Ultrasonic Symposium 2009:467-70.
- Langlais T, Vergari C, Pietton R, Dubousset J, Skalli W, Vialle R. Shear-wave elastography can evaluate annulus fibrosus alteration in adolescent scoliosis. Eur Radiol 2018. Epub ahead of print. [Crossref] [PubMed]
- Uff C, Garcia L, Fromageau J, Chakraborty A, Dorward N, Bamber J. Further characterization of changes in axial strain elastograms due to the presence of slippery tumor boundaries. J Med Imaging (Bellingham) 2018;5:021211. [Crossref] [PubMed]