Selective dorsal rhizotomy: current state of practice and the role of imaging
Introduction
Spastic diplegia is a subtype of bilateral spastic cerebral palsy (CP). It is the most common form of CP and is strongly associated with prematurity (1). While spasticity is present in all four limbs, the lower limbs are consistently more severely affected. The upper limbs may appear relatively normal but fine motor function and dexterity is diminished. These motor difficulties may represent motor planning difficulties or even dystonia (variable muscle tone) which is not uncommon to see even in children with classical spastic diplegia and periventricular leukomalacia (PVL) on magnetic resonance imaging (MRI) brain scan. The motor disorder may evolve as muscle shortening, tendon contractures and rotational bone and joint deformities develop. Patients may develop a crouch gait as they grow, which makes walking increasingly difficult. As a result, although the primary neurological lesion is non-progressive, CP can appear to clinically deteriorate as the child grows (2-4).
The severity of the motor disability in spastic CP is classified according to the Gross Motor Function Classification System (GMFCS) (5). Definition of a child’s GMFCS level requires detailed physical assessment and carries prognostic implication (6). Patients with GMFCS grade III–V demonstrate a decline in function over time (6), highlighting the need for early and effective treatment of their spasticity.
Although the diagnosis of CP is clinical, MRI contributes to establishing the diagnosis in equivocal cases (7). PVL is seen in over 70% of patients with bilateral diplegia (8). PVL compromises supratentorial influence to the spinal neuronal pool, and abnormal inputs through the vestibular and reticular nuclei and their tracts results in loss of inhibition to the spinal reflex arcs. This results in an increase in tone, and, in particular, damage to the vestibulospinal tracts increases extensor tone. Due to the topographic arrangement of the periventricular white matter, smaller lesions, usually limited to the trigonal regions of the ventricles, primarily affect the lower limbs, while more extensive lesions also cause upper limb spasticity.
A goal-oriented multidisciplinary approach to the management of CP should be considered throughout the patient’s life (2). Conservative, pharmacological and surgical interventions all play an important part. Among these, selective dorsal rhizotomy (SDR) is a valuable neurosurgical treatment option for spastic diplegia (3,9). While SDR has been used in patients with spastic quadriplegia, these patients generally have poorer outcomes (10). SDR in patients with spastic diplegia involves the selective division of afferent L1-S2 nerve roots in order to target aberrant reflex arcs contributing to spasticity of the lower limbs.
This article discusses the state of practice of SDR, emphasising the patient work up and technique. Long term outcomes are also discussed.
Patient workup
Patient selection for SDR is variable and has not been generally validated. It is typically based on clinical rationale rather than scientific evidence (11). The general selection criteria defined by Peacock in 1987 still apply (12), but the use of strict patient selection criteria can enhance outcomes. The current criteria at Great Ormond Street Hospital for Children (GOSH) are shown in Table 1 (9), which is broadly similar to the Peacock selection criteria.
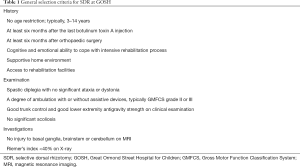
Full table
Two contemporaneous landmark case series from Cape Town and Oswestry highlight the significant advantage of strict selection criteria (12,13). The Cape Town series used Peacock’s criteria (12). Their results were consistent with randomised controlled trials and meta-analyses, demonstrating reduction in spasticity, as well as improvement in gait and range of movement; although GMFM scores improved, children tended to stay within their GMFCS grade (12). In contrast, Oswestry conducted a strict selection process, including a multidisciplinary assessment that encompassed elements of the Peacock criteria (13). Their selection criteria are shown in Table 2; it encompassed elements of the Peacock criteria as well as spine and hip radiographs, gait analysis, EMG, and MRI of the brain and spinal cord (13). Only 35% of referred children were selected for SDR. 90% of the selected patients were able to improve their GMFCS level (13), though the long term results are not known.
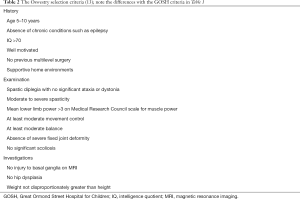
Full table
It should be noted that the strict Oswestry criteria select for patients who are more likely to have a good outcome, and may exclude patients who could benefit from the reduction in spasticity even though they may not improve their GMFCS level. Benefits beyond GMFCS improvement are real and need to be balanced in the choice of selection criteria.
History
Age is an important consideration for SDR due to both patient maturity and the natural history of CP. Below the age of 3, children are unlikely to respond well to intensive postoperative physiotherapy. Young children are still establishing motor patterns and it is important to understand the natural motor trajectory of the child before significant intervention. Abnormal muscle tone such as dystonia may be overcome with motor maturation but in some instances it can become more evident as the child develops.
These factors are not necessarily exclusions for going forward with SDR but may alter the final outcome and goals for the child and family. It is therefore often sensible not to simply rush to SDR without suitable MDT assessment. Over the age of 10 weakness and lower limb deformity are often more relevant than spasticity, and these children may have better outcomes with multi-level orthopaedic surgery (14). However, older children and young adults with predominant spasticity still benefit from SDR (15). This raises the importance of quality selection algorithms for patients with bilateral spastic CP (11).
The establishment of realistic and specific goals prior to SDR is essential. The evidence supports the use of SDR to address spasticity (16) but children are unlikely to progress to higher GMFCS levels following rehabilitation. In practice, the importance of setting appropriate specific objectives with families, within the context of the surgical risks and the need for intensive post-operative rehabilitation, cannot be overemphasised. In our experience in ambulant children SDR leads to improved mobility, increased stamina, better balance and fewer falls. Children who walk with assistance become more independent. Sitting and standing posture improves. In addition, the pain associated with spasticity responds well to SDR (9).
Examination
Although the GMFCS is useful to provide a clear clinical description, it does not provide sufficient detail to evaluate a child’s baseline disability and its change following interventions, including SDR. The Gross Motor Function Measure (GMFM) is widely used to provide the finer quantitative detail for assessment (17). More recently, the gradual decline in GMFM scores in older children and young adults, within the five GMFCS bands, has been defined (6). This is helpful in evaluating the effects of an intervention over a background of the natural history of progressive deterioration in CP for the individual child.
Investigations
Brain MRI is essential prior to SDR and in most cases confirms PVL. Typical features of PVL are shown in Figure 1. Children with PVL in which the white matter scarring extends significantly anteriorly to involve the basal ganglia and thalami are generally considered a contraindication to SDR (11).
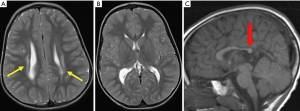
The highest risk for PVL occurs between 23 and 32 weeks’ gestational age (2). PVL may be focal or diffuse, ranging from areas of hyperintensity within the deep white matter adjacent to the ventricular ependyma at the trigone, evident on T2-weighted MR imaging, to extensive involvement around the entire ventricular system. When severe, it leads to loss of white matter tissue, cavitation and cyst formation, as well as passive ventriculomegaly with a thin corpus callosum. Figure 2 shows a more severe case of PVL with extensive scarring, which is assessed as unlikely to have a good outcome.
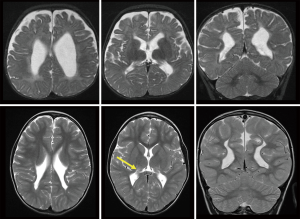
MRI is particularly important in defining the extent of brain injury and potential improvement with SDR. Severe hypoxic ischemic injury may lead to watershed infarcts involving cortical tissue and subcortical white matter, as well as metabolically active tissues such as the thalamus, hippocampus and pyramidal tracts. Extensive involvement of the basal ganglia, as shown in Figure 3 is also consistent with hypoxic ischaemic injury sustained at term. This is associated with a poor prognosis; children often demonstrate significant dystonia in addition to spasticity and do not benefit from SDR to the same degree. Children with significant involvement of the thalami, basal ganglia, cerebellum or brainstem are likely to have difficulties in the postoperative rehabilitation phase.
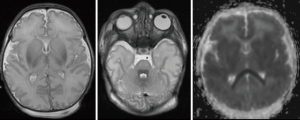
The single level procedure is dependent on a physiological location of the conus and a plain spinal radiograph is required to exclude spinal dysraphism. Radiological investigations are used to investigate hip subluxation, which is measured by Reimer’s index (18). It is measured by the percentage of the femoral head that is lateral to Perkin’s line on a plain radiograph. The relative risk of hip subluxation increases with GMFCS level (19) and increases over time as the disease clinically deteriorates (20).
Technique
SDR has evolved significantly since Foerster first performed dorsal rhizotomy for the relief of spasticity in 1908 (9). The single-level technique was first described by Park and Johnston (15). While it is technically more difficult than the multilevel approach, it does reduce operative time and recovery time.
A small midline fenestration is made at T12-L1 and allows identification of the conus using ultrasonography. The conus, and the L2 to S2 nerve roots, are then exposed through a single level laminectomy, usually at L1 or T12. There is a clear identifiable anatomical plane between the ventral (motor) and dorsal (sensory) roots (Figure 4); the motor roots are protected throughout the procedure. The L2 to S1 dorsal nerve roots are individually identified and divided into 4–5 rootlets.
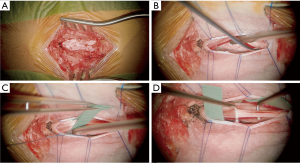
The L2 to S1 sensory nerve roots are systematically stimulated using intraoperative electromyography (EMG) to determine their threshold amplitude. Electrodes are placed in the psoas (L2), vastus lateralis (L3), tibialis anterior (L4), peroneus longus (L5) and gastrocnemius (S1). External anal sphincter tone is monitored for damage to the pudendal nerve during the operation.
Each rootlet is stimulated at the threshold amplitude at a frequency of 50 Hz and then graded as shown in Table 3 (9). The objective of the procedure is to divide approximately 60% to 70% of the sensory roots between L2 and S1. Rootlets with grade 3 to 4 responses are preferentially divided. In addition, 50% of the L1 sensory nerve root is divided as it exits its foramen. Intraoperative monitoring of the pudendal nerve together with limited division of the S2 nerve root is now commonly practised to reduce the risk of incontinence and to prevent unmasking excessive weakness and precipitating crouch gait.
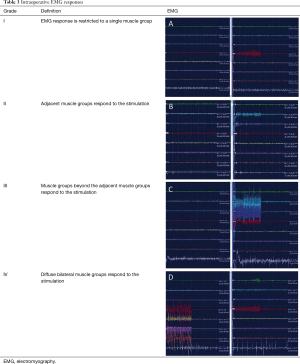
Full table
Reported intraoperative complications are primarily focused on anaesthetic risks, such as bronchospasm and aspiration due to pre-existing respiratory distress or gastro-oesophageal reflux disease, which are common in this patient population (21). Intraoperative surgical complications however may include bleeding, damage to surrounding structures, inadequate closure of the dura or technical difficulties relating to the spinal anatomy that could result in the abandonment of the surgery; these may result in acute complications discussed below.
Outcomes
Possible acute complications of the procedure include infection, haemorrhage and leak of cerebrospinal fluid (16). Patients commonly experience transient dysaesthesiae or itch9. Permanent complications are rare following SDR. Multi-level SDR has been reported to result in spinal abnormalities, such as scoliosis, kyphosis, lordosis and spondylolisthesis, as well as hip subluxation (11,22-27). However, there is no clear evidence whether these abnormalities are the direct result of SDR or integral to the natural history of spastic CP (11). Nevertheless, the single level laminectomy aims to reduce the likelihood of such abnormalities, and this has been confirmed in a large patient series (28).
The evidence from randomised control trials suggests that functional gains following SDR are likely to be moderate (16). A meta-analysis (29) of three RCTs comparing SDR combined with physiotherapy to physiotherapy alone (30-32) found that GMFM increases by approximately 4% following the combined treatment, which is almost double what physiotherapy alone would achieve. Of these three RCTs, only McLaughlin et al. found no significant increase in GMFM (32). However, this can be attributed to the division of fewer dorsal rootlets than the current technique (9). In an RCT comparing SDR to botulinum toxin A injections, the effects of SDR were more enduring than botulinum toxin A injections and SDR patients had fewer orthopaedic interventions (33).
The long term effects of SDR are emerging. Daunter, Kratz and Hurvitz’s recent case-control study found that patients who underwent SDR as children were significantly more likely to have less functional decline compared with age-matched controls, however there was no significant difference in pain or fatigue (34). Park et al. recently followed up 294 adults who underwent SDR as children (28). They found that the benefits of SDR continued into adulthood with no long-term complications. A relevant limitation to these studies however relates to a relatively low response rate in a questionnaire-based study. Other studies on the long term effects are generally positive (9) with sustained improvements in lower limb muscle tone and no recurrence of spasticity (35-37). Notably, the Paediatric Evaluation of Disability Inventory (PEDI) scores of children classified as GMFCS I–III showed improvement in the functional skills, mobility and caregiver domains, whereas children classified as GMFCS IV–V showed small changes (35,36). While Park et al. found 59% of patients underwent subsequent orthopaedic surgery (28), other studies have found a significant reduction in the requirement for further orthopaedic intervention compared to other studies of children of similar ages who did not undergo SDR (37,38).
Less satisfactory long-term outcomes have been reported and provide a substantial contribution to the evidence base for patient selection (9). Based on this evidence, it is now generally agreed that good long-term results are achieved in young children (14), who are diplegic rather than quadriplegic (10), and those whose GMFCS grade is II–III (35-36). Indeed, MacWilliams et al. found that children over the age of 10 had better outcomes with multi-level orthopaedic surgery rather than SDR (14), which reflects the evolving nature of CP.
Patients tend to report improvements in activities of daily living (ADL) (24,39). For instance, the Cape Town experience found that 20 years after SDR there was a 58% improvement from baseline in ADL with no worsening of GMFCS grade (24). Moreover, one study reports that former patients would recommend SDR to other patients with CP, and are unlikely to report negative impressions of the procedure (40).
The postoperative care and rehabilitation is intensive and reinforces the need to select patients with a supportive home family and accessible rehabilitation services (41). Postoperatively, patients demonstrate muscle weakness and require intensive physiotherapy. Recovery following the single-level technique is generally rapid and children typically resume physiotherapy after 2 to 3 days of bed rest (42). Patients then undergo 3 weeks of twice daily physiotherapy. Depending on the child’s ability and progress, two to three sessions of physiotherapy are recommended every week for up to four months. Formal progress assessments are subsequently conducted at six, twelve and 24 months.
Conclusions
SDR has evolved over the past century to become a valuable management option in the treatment of spasticity in children with CP. The evidence base for patient selection criteria is emerging but will require a careful balance between optimal improvements in GMFCS and the impact on the natural history of spastic CP in a wider group of patients. Patients are unlikely to experience permanent complications with the use of the single laminectomy technique. Patients are likely to show a significant reduction in spasticity but are unlikely to advance in GMFCS level. Nevertheless, they are less likely to require further surgical interventions and improve their independence in ADL and quality of life (QOL) in the long term.
Acknowledgements
None.
Footnote
Conflicts of Interest: The authors have no conflicts of interest to declare.
References
- Huntsman R, Lemire E, Norton J, Dzus A, Blakley P, Hasal S. The differential diagnosis of spastic diplegia. Arch Dis Child 2015;100:500-4. [Crossref] [PubMed]
- Koman LA, Smith BP, Shilt JS. Cerebral palsy. Lancet 2004;363:1619-31. [Crossref] [PubMed]
- Albright AL. Neurosurgical options in cerebral palsy. Paediatrics and Child Health 2008;18:414-8. [Crossref]
- Wimalasundera N. Developmental problems and the child with special needs. In: Lissauer T, Clayden G, editors. Illustrated textbook of paediatrics (4 ed). Edinburgh: Mosby, 2012.
- Palisano R, Rosenbaum P, Walter S, Russell D, Wood E, Galuppi B. Development and reliability of a system to classify gross motor function in children with cerebral palsy. Dev Med Child Neurol 1997;39:214-23. [Crossref] [PubMed]
- Hanna SE, Rosenbaum PL, Bartlett DJ, Palisano RJ, Walter SD, Avery L, Russell DJ. Stability and decline in gross motor function among children and youth with cerebral palsy aged 2 to 21 years. Dev Med Child Neurol 2009;51:295-302. [Crossref] [PubMed]
- Korezeniewski SJ, Birbek G, DeLano MC, Potchen MJ, Paneth N. A systematic review of neuroimaging for cerebral palsy. J Child Neurol 2008;23:216-27. [Crossref] [PubMed]
- Bax M, Tydeman C, Flodmark O. Clinical and MRI correlates of cerebral palsy: the European Cerebral Palsy Study. JAMA 2006;296:1602-8. [Crossref] [PubMed]
- Aquilina K, Graham D, Wimalasundera N. Selective dorsal rhizotomy: the re-emergence of an old technique. Arch Dis Child 2015;100:798-802. [Crossref] [PubMed]
- Kim HS, Steinbok P, Wickenheiser D. Predictors of poor outcome after selective dorsal rhizotomy in treatment of spastic cerebral palsy. Childs Nerv Syst 2006;22:60-6. [Crossref] [PubMed]
- Grunt S, Fieggen AG, Vermuelen RJ, Becher JG, Langerak NG. Selection criteria for selective dorsal rhizotomy in children with spastic cerebral palsy: a systematic review of the literature. Dev Med Child Neurol 2014;56:302-12. [Crossref] [PubMed]
- Langerak NG, Lamberts RP, Fieggen AG, Peter JC, Peacock WJ, Vaughan CL. Selective dorsal rhizotomy: long-term experience from Cape Town. Childs Nerv Syst 2007;23:1003-6. [Crossref] [PubMed]
- Cole GF, Farmer SE, Roberts A, Stewart C, Patrick JH. Selective dorsal rhizotomy for children with cerebral palsy: the Oswestry experience. Arch Dis Child 2007;92:781-5. [Crossref] [PubMed]
- MacWilliams BA, Johnson BA, Shuckra AL, D'Astous JL. Functional decline in children undergoing selective dorsal rhizotomy after age 10. Dev Med Child Neurol 2011;53:717-23. [Crossref] [PubMed]
- Park TS, Johnston JM. Surgical techniques of selective dorsal rhizotomy for spastic cerebral palsy. Technical note. Neurosurg Focus 2006;21:e7. [Crossref] [PubMed]
- Novak I, McIntyre S, Morgan C, Campbell L, Dark L, Morton N, Stumbles E, Wilson SA, Goldsmith S. A systematic review of interventions for cerebral palsy: state of the evidence. Dev Med Child Neurol 2013;55:885-910. [Crossref] [PubMed]
- Russell DJ, Avery LM, Rosenbaum PL, Raina PS, Walter SD, Palisano RJ. Improved scaling of the Gross Motor Function Measure for children with cerebral palsy: evidence of reliability and validity. Phys Ther 2000;80:873-85. [PubMed]
- Reimers J. The stability of the hip in children: a radiological study of results from muscle surgery in cerebral palsy. Acta Orthop Scand Suppl 1980;184:1-100. [Crossref] [PubMed]
- Soo B, Howard JJ, Boyd RN, Reid SM, Lanigan A, Wolfe R, Reddihough D, Graham HK. Hip displacement in cerebral palsy. J Bone Joint Surg Am 2006;88:121-9. [PubMed]
- Scrutton D, Baird G. Surveillance measures of the hips of children with bilateral cerebral palsy. Arch Dis Child 1997;76:381-4. [Crossref] [PubMed]
- Farmer JP, Sabbagh AJ. Selective dorsal rhizotomies in the treatment of spasticity related to cerebral palsy. Childs Nerv Syst 2007;23:991-1002. [Crossref] [PubMed]
- Bolster EA, van Schie PE, Becher JG, van Ouwerkerk WJ, Strijers RL, Vermeulen RJ. Long-term effect of selective dorsal rhizotomy on gross motor function in ambulant children with spastic bilateral cerebral palsy, compared with reference centiles. Dev Med Child Neurol 2013;55:610-6. [Crossref] [PubMed]
- Grunt S, Becher JG, Vermuelen RJ. Long-term outcome and adverse effects of selective dorsal rhizotomy in children with cerebral palsy: a systematic review. Dev Med Child Neurol 2011;53:490-8. [Crossref] [PubMed]
- Langerak NG, Lamberts RP, Fieggen AG, Peter JC, van der Merwe L, Peacock WJ, Vaughan CL. A prospective gait analysis study in patients with diplegic cerebral palsy 20 years after selective dorsal rhizotomy. J Neurosurg Pediatr 2008;1:180-6. [Crossref] [PubMed]
- Li Z, Zhu J, Liu X. Deformity of lumbar spine after selective dorsal rhizotomy for spastic cerebral palsy. Microsurgery 2008;28:10-2. [Crossref] [PubMed]
- Steinbok P, Hicdonmez T, Sawatzky B, Beauchamp R, Wickenheiser D. Spinal deformities after selective dorsal rhizotomy for spastic cerebral palsy. J Neurosurg 2005;102:363-73. [PubMed]
- Johnson MB, Goldstein L, Thomas SS, Piatt J, Aiona M, Sussman M. Spinal deformity after selective dorsal rhizotomy in ambulatory patients with cerebral palsy. J Pediatr Orthop 2004;24:529-36. [Crossref] [PubMed]
- Park TS, Edwards C, Liu JL, Walter DM, Dobbs MB. Beneficial effects of childhood selective dorsal rhizotomy in adulthood. Cureus 2017;9:e1077. [PubMed]
- McLaughlin J, Bjornson K, Temkin N, Steinbok P, Wright V, Reiner A, Roberts T, Drake J, O'Donnell M, Rosenbaum P, Barber J, Ferrel A. Selective dorsal rhizotomy: meta-analysis of three randomized controlled trials. Dev Med Child Neurol 2002;44:17-25. [Crossref] [PubMed]
- Wright FV, Shell EM, Drake JM, Wedge JH, Naumann S. Evaluation of selective dorsal rhizotomy for the reduction of spasticity in cerebral palsy: a randomized controlled trial. Dev Med Child Neurol 1998;40:239-47. [Crossref] [PubMed]
- Steinbok P, Reiner AM, Beauchamp R, Armstrong RW, Cochrane DD, Kestle J. A randomized clinical trial to compare selective posterior rhizotomy plus physiotherapy with physiotherapy alone in children with spastic diplegic cerebral palsy. Dev Med Child Neurol 1997;39:178-84. [Crossref] [PubMed]
- McLaughlin JF, Bjornson KF, Astley SJ, Graubert C, Hays RM, Roberts TS, Price R, Temkin N. Selective dorsal rhizotomy: efficacy and safety in an investigator-masked randomized clinical trial. Dev Med Child Neurol 1998;40:220-32. [Crossref] [PubMed]
- Wong AM, Pei YC, Lui TN, Chen CL, Wang CM, Chung CY. Comparison between botulinum toxin type A injection and selective posterior rhizotomy in improving gait performance in children with cerebral palsy. J Neurosurg 2005;102:385-9. [PubMed]
- Daunter AK, Kratz AL, Hurvitz EA. Long-term impact of childhood selective dorsal rhizotomy on pain, fatigue, and function: a case-control study. Dev Med Child Neurol 2017;59:1089-95. [Crossref] [PubMed]
- Nordmark E, Josenby AL, Lagergren J, Andersson G, Strömblad LG, Westbom L. Long-term outcomes five years after selective dorsal rhizotomy. BMC Pediatr 2008;8:54. [Crossref] [PubMed]
- Josenby AL, Wagner P, Jarnlo GB, Westbom L, Nordmark E. Functional performance in self-care and mobility after selective dorsal rhizotomy: a 10-year practice-based follow-up study. Dev Med Child Neurol 2015;57:286-93. [Crossref] [PubMed]
- Dudley RW, Parolin M, Gagnon B, Saluja R, Yap R, Montpetit K, Ruck J, Poulin C, Cantin MA, Benaroch TE, Farmer JP. Long-term functional benefits of selective dorsal rhizotomy for spastic cerebral palsy. J Neurosurg Pediatr 2013;12:142-50. [Crossref] [PubMed]
- Watt JM, Robertson CM, Grace MG. Early prognosis for ambulation of neonatal intensive care survivors with cerebral palsy. Dev Med Child Neurol 1989;31:766-73. [Crossref] [PubMed]
- Chicoine MR, Park TS, Kaufman BA. Selective dorsal rhizotomy and rates of orthopaedic surgery in children with spastic cerebral palsy. J Neurosurg 1997;86:34-9. [Crossref] [PubMed]
- Hurvitz EA, Marciniak CM, Daunter AK, Haapala HJ, Stibb SM, McCormick SF, Muraszko KM, Gaebler-Spira D. Functional outcomes of childhood dorsal rhizotomy in adults and adolescents with cerebral palsy. J Neurosurg Pediatr 2013;11:380-8. [Crossref] [PubMed]
- Graham D, Aquilina K, Cawker S, Paget S, Wimalasundera N. Single-level selective dorsal rhizotomy for spastic cerebral palsy. J Spine Surg 2016;2:195-201. [Crossref] [PubMed]
- Cawker S, Aquilina K. Selective dorsal rhizotomy (the perspective of the neurosurgeon and the physiotherapist). Paediatrics and Child Health 2016;9:395-9. [Crossref]