Clinical utilities of quantitative ultrasound in osteoporosis associated with inflammatory rheumatic diseases
Introduction
Osteoporosis is characterized by reduced bone mass and microarchitectural deterioration of bone tissue, with consequent bone fragility and susceptibility to fractures (1). There are different defining criteria of osteoporosis on the basic of adult or pediatric populations. For adults, the WHO’s operational definition for osteoporosis specifies bone density of 2.5 standard deviations (SD) (T-scores) or more below the mean for young healthy adult women in dual emission X-ray absorptiometry (DXA), and osteopenia is defined as a T-score between −1 and −2.5 (2). In the pediatric population, the International Society of Clinical Densitometry determined low bone mineral density as a Z-score (not T-score) below –2.0 in children. T-score is not used for this definition as adolescent’s bone density varies with age (3), and so it is not appropriate for growing children (4).
Osteoporosis is one of the most ubiquitous skeletal diseases, with 10 million individuals currently diagnosed in the United States (5) and 2 million having osteoporotic fractures for each year (6). Osteoporotic fractures are closely associated with increased mortality. About 20–30% of patients with osteoporotic fracture die due to long-term immobilization and postoperative complications within 6 months (7). Therefore, the ability to diagnose osteoporosis before fractures occur, and timely treatment of osteoporosis are important.
From a pathological point of view, in osteoporosis, radiological imaging plays a role in the identification of early bone weakening and evaluation of patterns of bone alterations (8). From a clinical point of view, radiological imaging is used for fracture risk prediction, screening for the osteoporosis, deciding on the choice of the treatment, and monitoring disease progression and therapeutic response (9).
Prevalence of osteoporosis in inflammatory rheumatic diseases
The prevalence of osteoporosis varies broadly, depending on the particular types of rheumatic diseases as shown in Table 1. Even when the same WHO defining criteria for osteoporosis in terms of DXA was applied to a specific disease, inconsistent prevalence estimates are detected across the studies as a result of several factors such as characteristics of study population, age and sex of participants, disease activity, disease duration, treatment received, concomitant glucocorticoid therapy and site of BMD measurement (10,11,31). In the cross-sectional study, the low bone mineral density (BMD) in lumbar spine and hip was reported in 45% of adult who had a history of juvenile idiopathic arthritis (JIA) in childhood (32). So, the full remission of the disease in young adults cannot completely normalize BMD at all skeletal sites (33), posing them at risk of developing premature osteoporosis and associated fractures later in life. Osteoporosis, therefore, imposes a large burden on patients with inflammatory rheumatic diseases.
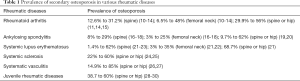
Full table
Secondary osteoporosis is an important co-morbidity in rheumatic disorders due to several mechanisms including the effects of inflammation on bone modeling, which increase osteoclast activation and subsequent bone resorption mediated by proinflammatory cytokines (34), physical inactivity attributed to painful joints and muscle weakness, increasing the osteoporosis risk, and the adverse skeletal effects related to the therapies administered to treat these diseases, e.g. glucocorticoids (GC) (Figure 1) (35,36). Substantial evidence shows that cross-talk between inflammatory cells and bone cells leads to production of a wide spectrum of cytokines such as interleukin-6 (IL-6), tumor necrosis factor (TNF), C-reactive protein (CRP), receptor activator of nuclear factor kappa-β ligand (RANKL) at sites of inflammation, that stimulate local and generalized bone resorption, and that inhibit in RA, or stimulate in AS, bone formation (37,38).
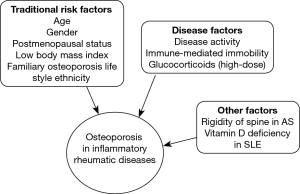
Bone density
Bone composition includes mineral, mainly calcium hydroxyapatite, embedded in type I collagen and specialized proteins forming the bone matrix. Calcium absorbs much more radiation than protein or soft tissue. The amount of X-ray energy that is absorbed by calcium in a section reflects the bone mineral content (BMC), which is divided by the area or volume of the bone to get estimated bone densitometry (BMD).
In laboratory studies, there is a high correlation (r=0.62) between BMD and bone breaking strength (39). Other determinants of bone strength include size, macroscopic structure (long bones with greater cross-sectional areas are more resistant to bending forces), microscopic structure (loss of normal trabecular architecture), and the composition of bone proteins (abnormal collagen) (40).
Bone evaluation to quantify BMD can be performed by various methods, including dual-emission X-ray absorptiometry (DXA), quantitative ultrasound (QUS) and quantitative computed tomography (QCT).
Quantitative methods
DXA
Bone densitometry utilizing DXA is the most widely used quantitative technique in clinical practice, and remains the gold-standard test for the osteoporosis diagnosis and quantification. Of all modalities, it has the most data on predicting fractures in post-menopausal women in longitudinal population-based studies (41) .
Lumbar spine is the primary site for BMD measurement, either providing total spine (from L1 to L4) or individual vertebral T-scores (9). Vertebral bodies largely comprise trabecular bone, possess a high ratio of remodeling surface to bone volume, and so are more sensitive to treatment or disease changes than cortical bone in other sites. The other most common sites of measurements are the hip region including femoral neck, trochanter, Ward’s area, intertrochanteric region, and total hip respectively, with the BMD of proximal femur being the best predictor of hip fracture (25) while lumbar spine bone mineral density does best in monitoring treatment effects (26).
However, spine BMD can be overestimated in degenerative arthritis, aortic calcification, etc. as standard anteroposterior spine BMD includes mineral in the posterior elements and facet joints as well as the abdominal aorta. Caution should, therefore, be exercised in interpreting spine BMD after about age 65 years (42).
As it is assessed in 2 dimensions, the section size of the bone has an impact on it: if a large and a small bone have the same mineral density, the larger will appear to have a higher BMD (Figure 2). Care should therefore be taken in interpreting longitudinal measurements of BMD in pediatric population such as in JIA due to potential size effects (4).
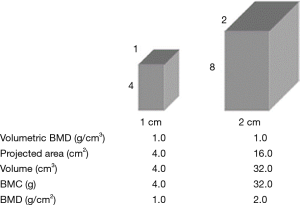
Another serious limitation of DXA is the inability of DXA to evaluate bone quality and microarchitecture of the trabeculae, which correspond to up to 50% of the mechanical strength of bone, representing a relationship of 0.43 between bone density and bone strength (44). This association explains why the fracture risk (the ultimate complication of osteoporosis) may be greater than the DXA would suggest, highlighting the importance of the fragile bone microarchitecture. In addition, this method incurs some major disadvantages, such as little information about cortical bone properties, the necessity of radiation exposure, limited accessibility, the need of some trained personnel, overestimations of bone density by marginal osteophyte (45) and vascular calcifications projecting on lumbar spine (46), and high cost.
In addition, DXA has several other limitations in measuring osteoporosis related with inflammatory arthritis as a result of the negative impact of inflammatory cytokines on bone health (36,47) and the required use of glucocorticoid in these diseases (48,49). In rheumatoid arthritis patients, treated with GC, the risk of vertebral fracture has been reported as having increased 6-fold compared with controls, whereas the reduction of lumbar spine BMD was only 0.79 SD (50). A recent meta-analysis on glucocorticoid-induced osteoporosis revealed that fractures occur at a much higher rate than expected on the basis of BMD, and that BMD changes during GC therapy may predict, only to a moderate extent, the increase in fracture risk (49).
QCT
QCT produces volumetric 3D measurements by using low-dose scan protocols on a standard CT scanner for the spine (axial QCT) or by working on dedicated extremity scanner for peripheral quantitative computed tomography (pQCT). Axial QCT measures trabecular bone in spinal vertebrae and usually scans between T11 and L4 in 2D single slice QCT of the spine or includes only two vertebrae, often L1 and L2, in the case of spiral multi-detector CT to reduce radiation dose (51) (Figure 3). Fractured vertebrae should not be analyzed as the inclusion of the endplate will overestimate the BMD. An oval region of interest with as much of the vertebral trabecular bone as possible, without the inclusion of the cortical rim or basi-vertebral vein, is selected for providing the trabecular BMD in mg/cm3 of individual vertebrae scanned. The result is compared with a phantom as bone mineral reference standard to calibrate each scan, and usually expressed in absolute values or as Z-scores and T-scores.
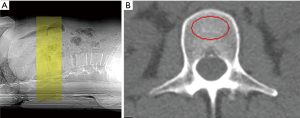
QCT has some limitations such as the effect of marrow changes on trabecular measurements (myelofibrosis, hematopoietic disorders, etc), expensiveness (51), high radiation dose (90 to 3,000 µSv) compared with DXA (1–6 µSv) (53,54), lack of standardization of examinations among the CT devices produced by different manufacturers (55) and the partial volume effect (underestimation of cortical BMD when the thickness of the cortical bone shell is less than 2 mm), which happens when a voxel in the image represents more than one tissue (56).
QUS
The use of QUS to investigate osteoporosis in cancellous heel bone was first introduced in 1984 (57), and involves placing ultrasound transducers on either side of the calcaneus; one acts as a wave transmitter and the other acts as the receiver (58). The transmission of ultrasound of frequency range between 200 and 1.5 MHz through bone tissue reflects its density and its structure (59). The majority of QUS research in the literature has focused on the calcaneal site as it has a high metabolic activity and demineralization pattern similar to the spine (60), although some other sites such as patella, tibia, phalanges and radius have also been studied.
The two main parameters measured in QUS are: broadband ultrasound attenuation (BUA) in decibel per megahertz and speed of sound (SOS) in meters per second. From these measures, a number of other measures can be derived including stiffness index, QUS index, amplitude-dependent speed of sound (AD-SoS), and bone transmission time (BTT) (61) (Figure 4).
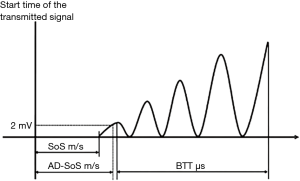
BUA represents a measure of the ultrasound variation of attenuation with the incident frequency of sound wave, primarily by absorption in cortical bone and scattering in the cancellous bone (63), and is expressed on a logarithmic scale over the range 0.1–1 MHz. SOS measures the distance the ultrasound signal travels per unit of time, independent of ultrasound wave attenuation (64). BUA and SOS parameters are usually measured by QUS devices used for heel, radius, tibia and patella (Figure 5) (62).

QUS index and stiffness are composite parameters derived from BUA and SOS, or velocity of sound (VOS), and described as a percentage of the result from young adults or the percentage of weight-matched references according to the manufacturer (65). QUS measures can be recorded in absolute values, or in T-score and Z-score compared to normative reference data (9). AD-SoS measures the interval from the starting time of the transmitted signal until the predetermined minimum amplitude of 2 mV is reached for the first time by the ultrasound signal received. BTT reflects the bone properties independent of the confounding effect of soft tissue and expressed in microsecond. AD-SoS and BTT are primarily quantified by the phalangeal QUS device (62).
In vitro studies, SOS is closely associated with bone mineralization with a resulting high correlation (r=0.888) between SOS and BMD at the same measurement site (66-68). However, BUA seems to depend more on the structural characteristics of trabecular bone (porosity, etc.) (67). In the case of SOS, the coefficient of variations (precision values) tend to be better in cortical bone compared to trabecular bone due to increased speed of sound waves in cortical bone. Similarly, BUA precision also appears to be poorer than its corresponding SOS precision in the same devices (60).
QUS possesses the main advantages of detecting different bone properties such as bone density, bone microarchitecture and elasticity (69). They are smaller, portable and cheaper than central DXA scanners, need no specially trained personnel, and do not use ionizing radiation (70).
The aim of this narrative review is to examine in inflammatory rheumatic diseases whether (I) QUS could be used to correlate with BMD in terms of WHO criteria by DXA; (II) QUS could predict disease activity and its usefulness; and (III) QUS could predict fracture in these populations.
QUS in inflammatory rheumatic diseases
To examine these potential clinical utilities of QUS in inflammatory rheumatic diseases, the current narrative review was based on electronic database search, Medline and Embase via Ovid, covering a period from their respective inception until 30th September 2017. These databases were looked up individually for all possible terms (MeSH and key word), and the combination of terms to meet differences in their search engines. The terms used with Boolean operators “OR” for three search strategies were (I) osteoporosis and bone density; (II) QUS, ultrasound and ultrasonography; (III) rheumatic disease, inflammatory joint disease, inflammatory arthritis, rheumatoid arthritis, juvenile idiopathic arthritis, ankylosing spondylitis, systemic lupus erythematosus, psoriatic arthritis (PA), systemic sclerosis, polymyositis, dermatomyositis, Sjogren’s syndrome and vasculitis. Then the Boolean operator “AND” was used among the three results. Only original articles were included while excluding animal studies, review articles, case repots, publications focusing on surgery, sample size less than 25, and non-English papers. After screening titles and abstracts (n=230), and then full texts (n=83) for exclusion criteria, 34 articles were included in this narrative review. Of these, 13 papers examined the correlation of QUS with BMD; 22 papers evaluated the association of QUS with disease activity; 3 papers assessed the fracture prediction of QUS, as shown in the flow diagram (Figure 6).
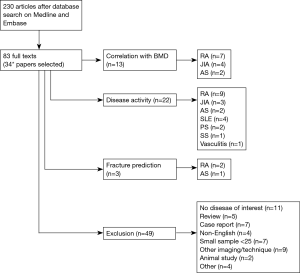
Rheumatoid arthritis (RA)
QUS association with BMD
In a sample of 51 RA patients, SOS of proximal phalanges was significantly correlated with lumbar spine, femoral neck and hand BMD, using DXA, (r=0.49, 0.51 and 0.72 respectively) (71). Calcaneal ultrasound demonstrated significant correlation of BUA with bone mineral content (BMC) and BMD (r=0.6572, 0.6081, respectively), and of SOS with BMC and BMD (r=0.4704, 0.4723) (72), suggesting the potential utility of QUS to evaluate BMD where DXA is unavailable.
In two separate case-control studies with 115 RA and 210 RA patients respectively, calcaneal QUS could discriminate between RA patients and controls better than DXA based on standardized response mean (73) and AUC (AUC =0.67–0.68 for QUS, and AUC =0.60–0.65 for DXA) (74), and between patients with and without vertebral deformities (74). The heel scan in 46 RA patients revealed a sensitivity of 90%, a specificity of 44% for a diagnosis of osteoporosis compared with DXA, a positive predictive value of 31% and a negative predictive value of 94%, suggesting the potential utility of heel ultrasound as a primary screening device (75). However, in the case of corticosteroid-induced osteoporosis, QUS did not appear to discriminate effects of corticosteroids on bone better than DXA in 76 RA patients (76).
In an interventional trial with 30 RA patients, AD-SoS values increased by 1.3% after 6 months of anti-TNF-α treatment while BMD increased by 0.2% at the lumbar spine and 0.1% at the hip. On the contrary, the AD-SoS levels decreased by 4.6% during the same period in the untreated RA group while BMD decreased by 0.8% and 0.6% (at lumbar spine and the hip, respectively). This study might support that QUS could capture the effect of anti-TNF therapy on generalized and periarticular osteoporosis in RA patients (77). Long-term studies are required to answer the clinical importance/utility of this finding.
Relation of QUS to underlying disease characteristics
In 60 patients with RA, a significant reduction was detected in the Z scores with increased disease duration (−1.52 vs. −2.12, P=0.004), and the Z score for AD-SoS was lower in those with disease duration of less than 2 years (−1.71) than in those with disease duration of 2–4 years (−1.01) (78). A similar positive association between the severity of QUS osteoporosis at the calcaneus and symptom duration was reported in a larger sample (n=256) (79). There existed an association between disease activity as determined by swollen joint and a combined swollen and tender joint and SOS, supporting the presence of a potentially adverse effect of clinically active disease on the bone (80).
The pronounced bone loss at the proximal phalanges of digits II–V was documented in patients with disease duration of 18–72 months (early RA) at the subchondral regions on measurements with SOS (81,82). However, discrepant results were published by Dragon et al. who determined failure of QUS parameters in 32 patients with early peripheral polyarthritis (median disease duration =4 months) to classify these patients into RA or another rheumatic disease (83).
In a number of studies, RA patients using the finger ultrasound technique also failed to show a correlation between Z scores and disease activity markers such as ESR or CRP (71,78,84). Finger ultrasound was moderately correlated with measures of hand function, with correlation coefficients of 0.37 and 0.39 for health assessment questionnaires and grip strength (71).
QUS with fracture prediction
In a study (n=825) which included a subset of RA patients (17%), QUS identified a higher number of women with increased fracture risk than the FRAX tool whose association was also relatively low, suggesting the incorporation of QUS parameters as an upgraded model of FRAX (85). Another study also reported an increased risk of fracture in patients with lower values of QUS (86).
Juvenile idiopathic arthritis
QUS association with BMD
A significant positive correlation (r=0.54, P<0.001) was detected between the lumbar DEXA and radius SOS (n=40) (87). Spine and total body BMD measured by DXA correlated significantly with tibia SOS (spine: r=0.57, P<0.007; total body: r=0.68, P<0.001) in another study (88). Calcaneal BUA measurements were lower in the juvenile rheumatic patients compared with a control group (P<0.001) and significantly correlated (r=0.83) with lumbar spine BMD in a mixed sample of 29 RA, 13 SLE and 11 dermatomyositis patients, suggesting a probable application of QUS technique in estimating bone density in children (89).
A low bone mass and quality were detected in 151 patients with JIA compared to controls, and the normal bone condition was not obtained over time especially in children with polyarticular or systemic onset despite the current more effective drugs, potentially posing a high risk of osteoporosis in early adulthood (90).
QUS with underlying disease characteristics
One study documented improvement of bone QUS parameters in JIA after 1-year etanercept therapy which might be due to suppression of the underlying disease activity (91). A longitudinal study (n=166) followed up until puberty determined a significant negative association between AD-SoS, and systemic corticosteroids exposure or number of intra-articular corticosteroids injections, a positive association among TNF-alpha-blocking agents and AD-SoS, and no improvements in their QUS z-scores with respect to baseline (92). QUS parameters had a significant negative correlation with disease duration [(r=0.57 (BUA), r=0.67 (VOS)] and cumulative dose of prednisone [r=0.48 (BUA), r=0.50 (VOS)] in children with polyarticular JIA (93).
QUS with fracture prediction
There is a lack of studies examining the fracture prediction of QUS in this disease population.
Ankylosing spondylitis
QUS association with BMD
Weak to good correlations (r=0.22 to 0.53) were found between lumbar spine, femoral neck and total body BMD, and the different calcaneal QUS variables in 71 early AS patients (94). There was a significant correlation of calcaneal SOS with hip BMD (r=0.43) in 23 women with AS (95).
QUS with underlying disease characteristics
Calcaneal QUS parameters did not reveal significant association with variables of disease activities including ESR, serum CRP levels and BASDAI (94,95).
QUS with fracture prediction
In a study including 50 AS patients, increased calcaneal QUS, with a cut-off level T <−1.0 provided 70% sensitivity, 68% specificity, 35% positive predictive value and 90% negative predictive value, with femoral neck BMD. It might suggest the applicability of QUS to exclude severe osteoporosis (96). In the same study, it was reported that the sensitivity of QUS T <−1.0 to find the fractures was 80%, and the sensitivity of femoral neck DXA T score <−2.5 was 60% (96).
Systemic lupus erythematosus
There is no study for QUS correlation with BMD and fracture prediction in the disease, which met our criteria.
QUS with underlying disease characteristics
In a case-control study in a mixed sample of SLE and RA patients (n=88), SOS but not BUA and DXA measurements reflected disease activities assessed by erythrocyte sedimentation rate (ESR) and CRP, suggesting that SOS might be more sensitive to alteration of bone secondary to the inflammation process and may reflect short-term bone status (97). In another study (n=43), young adults with SLE showed lower values of AD-SoS than controls, and had a low bone mass without catch-up growth over time, compared to healthy subjects, leading to a reduced final peak bone mass (98). Juvenile onset SLE patients had a reduced AD-SoS and QUS z-score (P<0.005) (99,100).
Psoriatic arthritis
No study exists for QUS correlation with BMD and fracture prediction in this disease.
QUS with underlying disease characteristics
Among psoriatic arthritis patients, reduced QUS parameters in at least one skeletal region were observed in 67% of premenopausal women, 100% of postmenopausal women, and 80% of the men. This was not related to the indices of inflammation or disease duration (101).
One study examined the responsiveness of QUS in a mixed sample of RA and PA (n=163), using clodronate (100 mg IM/week) with significant changes of QUS stiffness over 48 months (102).
Systemic sclerosis
Studies are lacking for QUS correlation with BMD and fracture prediction in this disease.
QUS with underlying disease characteristics
In a cross-sectional study with 55 patients with systematic sclerosis, reduced BMD and SI was more marked in the diffuse form and in those with internal organ involvement. The QUS stiffness index was not related to inflammation indices, disease duration, or to the immunological pattern (103).
Vasculitis
No study exists for QUS correlation with BMD and fracture prediction in this disease.
QUS with underlying disease characteristics
Although significant reduction of AD-SoS was detected in RA patients, compared to the vasculitis and control groups, no significant difference existed between the latter groups despite the substantial glucocorticoid dose in vasculitis group. This finding might suggest that phalangeal QUS measurements are particularly suited to the study of bone destruction induced by immobilization or local inflammation rather than that induced by the detrimental effect of corticosteroid treatment (82).
Limitations of QUS
The major limitation of QUS in inflammatory rheumatic disease is the proliferation of various types of QUS scanners based on different ultrasound principles and applied to a variety of anatomical sites (104), utilizing different ultrasound mechanisms such as trabecular transverse transmission mostly measured at the heel, cortical transverse transmission used at the phalanges, cortical axial transmission applicable to the phalanges, radius and tibia (62,105). Therefore, it is inappropriate to directly compare measurements acquired with different QUS devices which are technologically different. The international consensus definition of osteoporosis and/or osteopenia using QUS variables is also still lacking.
In addition, most of the studies are focused on RA and JIA, and majority of these studies are not based on large population samples (n<100 patients in most studies). Although the limited literature in these diseases demonstrated that QUS provided a substantial correlation with BMD, additional information in fracture prediction models and considerable diagnostic accuracy against BMD, further evidence is still required to be proposed as a diagnostic tool or screening instrument or combined fracture risk prediction model in these diseases (106). Regarding disease activities, QUS lacked significant correlation in most inflammatory diseases. Future studies based on large samples are required to demonstrate capability of QUS fracture prediction for these diseases, the ultimate goal of imaging in osteoporosis.
Conclusions
Bone mineral loss is a prevalent finding in inflammatory rheumatic diseases. Although QUS may have some complementary benefits to fracture risk prediction models, current literature does not support the substitution of QUS for DXA in the diagnosis and monitoring of osteoporosis in the rheumatic diseases. Most of the QUS studies are focused only on RA and JIA. In the case of RA, QUS has moderate to substantial correlation with DXA, a weak association with function and good discriminative validity between disease and control; however, the literature is conflicting in the use of early disease process, and corticosteroid-induced osteoporosis. In JIA, QUS parameters seem to improve after treatment with biologicals. To determine the utility of QUS in improving outcomes for osteoporotic rheumatic patients, future research is still required for evaluation of QUS clinimetrics as well as the cost-effectiveness of screening strategies that incorporate QUS in current fracture risk assessment tools.
Acknowledgements
None.
Footnote
Conflicts of Interest: The authors have no conflicts of interest to declare.
References
- Consensus development conference. diagnosis, prophylaxis, and treatment of osteoporosis. Am J Med 1993;94:646-50. [Crossref] [PubMed]
- Assessment of fracture risk and its application to screening for postmenopausal osteoporosis. Report of a WHO Study Group. World Health Organ Tech Rep Ser 1994;843:1-129. [PubMed]
- Gordon CM, Baim S, Bianchi ML, Bishop NJ, Hans DB, Kalkwarf H, Langman C, Leonard MB, Plotkin H, Rauch F, Zemel BS. Special report on the 2007 Pediatric Position Development Conference of the International Society for Clinical Densitometry. South Med J 2008;101:740-3. [Crossref] [PubMed]
- Binkley TL, Berry R, Specker BL. Methods for measurement of pediatric bone. Rev Endocr Metab Disord 2008;9:95-106. [Crossref] [PubMed]
- Ekman EF. The role of the orthopaedic surgeon in minimizing mortality and morbidity associated with fragility fractures. J Am Acad Orthop Surg 2010;18:278-85. [Crossref] [PubMed]
- Bukata SV, DiGiovanni BF, Friedman SM, Hoyen H, Kates A, Kates SL, Mears SC, Mendelson DA, Serna FH, Sieber FE, Tyler WK. A Guide to Improving the Care of Patients With Fragility Fractures. Geriatr Orthop Surg Rehabil 2011;2:5-37. [Crossref] [PubMed]
- Kanis JA, Oden A, Johnell O, De Laet C, Jonsson B, Oglesby AK. The components of excess mortality after hip fracture. Bone 2003;32:468-73. [Crossref] [PubMed]
- Guglielmi G, Muscarella S, Bazzocchi A. Integrated Imaging Approach to Osteoporosis: State-of-the-Art Review and Update. Radiographics 2011;31:1343-64. [Crossref] [PubMed]
- Guglielmi G, Nasuto M, Avery LY, Cheng X. Bone densitometry: Current status and future trends. Journal of Gerontology and Geriatrics 2016;64:97-103.
- Haugeberg G, Uhlig T, Falch JA, Halse JI, Kvien TK. Bone mineral density and frequency of osteoporosis in female patients with rheumatoid arthritis: results from 394 patients in the Oslo County Rheumatoid Arthritis register. Arthritis Rheum 2000;43:522-30. [Crossref] [PubMed]
- Lee JH, Sung YK, Choi CB, Cho SK, Bang SY, Choe JY, Hong SJ, Jun JB, Kim TH, Lee J, Lee HS, Yoo DH, Yoon BY, Bae SC. The frequency of and risk factors for osteoporosis in Korean patients with rheumatoid arthritis. BMC Musculoskelet Disord 2016;17:98. [Crossref] [PubMed]
- Sinigaglia L, Nervetti A, Mela Q, Bianchi G, Del Puente A, Di Munno O, Frediani B, Cantatore F, Pellerito R, Bartolone S, La Montagna G, Adami S. A multicenter cross sectional study on bone mineral density in rheumatoid arthritis. Italian Study Group on Bone Mass in Rheumatoid Arthritis. J Rheumatol 2000;27:2582-9. [PubMed]
- Lodder MC, de Jong Z, Kostense PJ, Molenaar ET, Staal K, Voskuyl AE, Hazes JM, Dijkmans BA, Lems WF. Bone mineral density in patients with rheumatoid arthritis: relation between disease severity and low bone mineral density. Ann Rheum Dis 2004;63:1576-80. [Crossref] [PubMed]
- Forsblad D'Elia H, Larsen A, Waltbrand E, Kvist G, Mellstrom D, Saxne T, Ohlsson C, Nordborg E, Carlsten H. Radiographic joint destruction in postmenopausal rheumatoid arthritis is strongly associated with generalised osteoporosis. Ann Rheum Dis 2003;62:617-23. [Crossref] [PubMed]
- Hauser B, Riches PL, Wilson JF, Horne AE, Ralston SH. Prevalence and clinical prediction of osteoporosis in a contemporary cohort of patients with rheumatoid arthritis. Rheumatology (Oxford) 2014;53:1759-66. [Crossref] [PubMed]
- El Maghraoui A, Borderie D, Cherruau B, Edouard R, Dougados M, Roux C. Osteoporosis, body composition, and bone turnover in ankylosing spondylitis. J Rheumatol 1999;26:2205-9. [PubMed]
- Vasdev V, Bhakuni D, Garg MK, Narayanan K, Jain R, Chadha D. Bone mineral density in young males with ankylosing spondylitis. Int J Rheum Dis 2011;14:68-73. [Crossref] [PubMed]
- van der Weijden MA, Claushuis TA, Nazari T, Lems WF, Dijkmans BA, van der Horst-Bruinsma IE. High prevalence of low bone mineral density in patients within 10 years of onset of ankylosing spondylitis: a systematic review. Clin Rheumatol 2012;31:1529-35. [Crossref] [PubMed]
- Bessant R, Keat A. How should clinicians manage osteoporosis in ankylosing spondylitis? J Rheumatol 2002;29:1511-9. [PubMed]
- Wang DM, Zeng QY, Chen SB, Gong Y, Hou ZD, Xiao ZY. Prevalence and risk factors of osteoporosis in patients with ankylosing spondylitis: a 5-year follow-up study of 504 cases. Clin Exp Rheumatol 2015;33:465-70. [PubMed]
- Boyanov M, Robeva R, Popivanov P. Bone mineral density changes in women with systemic lupus erythematosus. Clin Rheumatol 2003;22:318-23. [Crossref] [PubMed]
- Mok CC, Mak A, Ma KM. Bone mineral density in postmenopausal Chinese patients with systemic lupus erythematosus. Lupus 2005;14:106-12. [Crossref] [PubMed]
- Uaratanawong S, Deesomchoke U, Lertmaharit S, Uaratanawong S. Bone mineral density in premenopausal women with systemic lupus erythematosus. J Rheumatol 2003;30:2365-8. [PubMed]
- Atteritano M, Sorbara S, Bagnato G, Miceli G, Sangari D, Morgante S, Visalli E, Bagnato G. Bone Mineral Density, Bone Turnover Markers and Fractures in Patients with Systemic Sclerosis: A Case Control Study. PLoS One 2013;8:e66991. [Crossref] [PubMed]
- Ibn Yacoub Y, Amine B, Laatiris A, Wafki F, Znat F, Hajjaj-Hassouni N. Bone density in Moroccan women with systemic scleroderma and its relationships with disease-related parameters and vitamin D status. Rheumatol Int 2012;32:3143-8. [Crossref] [PubMed]
- Andersson R, Rundgren A, Rosengren K, Bengtsson BA, Malmvall BE, Mellstrom D. Osteoporosis after long-term corticosteroid treatment of giant cell arteritis. J Intern Med 1990;227:391-5. [Crossref] [PubMed]
- Hatz HJ, Helmke K. Polymyalgia rheumatica and giant cell arteritis; diagnosis and side effects of low-dose long-term glucocorticoid therapy. Z Rheumatol 1992;51:213-21. [PubMed]
- Lilleby V, Lien G, Frey Froslie K, Haugen M, Flato B, Forre O. Frequency of osteopenia in children and young adults with childhood-onset systemic lupus erythematosus. Arthritis Rheum 2005;52:2051-9. [Crossref] [PubMed]
- Pereira RM, Corrente JE, Chahade WH, Yoshinari NH. Evaluation by dual X-ray absorptiometry (DXA) of bone mineral density in children with juvenile chronic arthritis. Clin Exp Rheumatol 1998;16:495-501. [PubMed]
- Regio P, Bonfa E, Takayama L, Pereira R. The influence of lean mass in trabecular and cortical bone in juvenile onset systemic lupus erythematosus. Lupus 2008;17:787-92. [Crossref] [PubMed]
- di Munno O, Mazzantini M, Sinigaglia L, Bianchi G, Minisola G, Muratore M, la Corte R, di Matteo L, Canesi B, Caminiti M, Broggini M, Adami S. Effect of low dose methotrexate on bone density in women with rheumatoid arthritis: results from a multicenter cross-sectional study. J Rheumatol 2004;31:1305-9. [PubMed]
- Zak M, Hassager C, Lovell DJ, Nielsen S, Henderson CJ, Pedersen FK. Assessment of bone mineral density in adults with a history of juvenile chronic arthritis: a cross-sectional long-term followup study. Arthritis Rheum 1999;42:790-8. [Crossref] [PubMed]
- French AR, Mason T, Nelson AM, Crowson CS, O'Fallon WM, Khosla S, Gabriel SE. Osteopenia in adults with a history of juvenile rheumatoid arthritis. A population based study. J Rheumatol 2002;29:1065-70. [PubMed]
- Redlich K, Smolen JS. Inflammatory bone loss: pathogenesis and therapeutic intervention. Nat Rev Drug Discov 2012;11:234. [Crossref] [PubMed]
- Mandl P, Kainberger F, Friberg Hitz M. Imaging in osteoporosis in rheumatic diseases. Best Pract Res Clin Rheumatol 2016;30:751-65. [Crossref] [PubMed]
- Boling EP. Secondary Osteoporosis: Underlying Disease and the Risk for Glucocorticoid-Induced Osteoporosis. Clinical Therapeutics 2004;26:1-14. [Crossref] [PubMed]
- Bultink IEM, Vis M, van der Horst-Bruinsma IE, Lems WF. Inflammatory rheumatic disorders and bone. Curr Rheumatol Rep 2012;14:224-30. [Crossref] [PubMed]
- Gao LX, Jin HT, Xue XM, Wang J, Liu DG. Osteoporosis in rheumatic diseases. World J Rheumatol 2015;5:23-35. [Crossref]
- Toth P, Horvath C, Ferencz V, Toth B, Varadi A, Szenci O, Bodo G. Bone mineral density (BMD) and computer tomographic measurements of the equine proximal phalanx in correlation with breaking strength. Pol J Vet Sci 2013;16:3-8. [Crossref] [PubMed]
- Cummings SR, Bates D, Black DM. Clinical use of bone densitometry: scientific review. JAMA 2002;288:1889-97. [Crossref] [PubMed]
- Szulc P, Munoz F, Duboeuf F, Marchand F, Delmas PD. Bone mineral density predicts osteoporotic fractures in elderly men: the MINOS study. Osteoporos Int 2005;16:1184-92. [Crossref] [PubMed]
- Steiger P, Cummings SR, Black DM, Spencer NE, Genant HK. Age-related decrements in bone mineral density in women over 65. J Bone Miner Res 1992;7:625-32. [Crossref] [PubMed]
- Carter DR, Bouxsein ML, Marcus R. New approaches for interpreting projected bone densitometry data. J Bone Miner Res 1992;7:137-45. [Crossref] [PubMed]
- Frost ML, Blake GM, Fogelman I. Does the Combination of Quantitative Ultrasound and Dual-Energy X-Ray Absorptiometry Improve Fracture Discrimination? Osteoporos Int 2001;12:471-7. [Crossref] [PubMed]
- Oei L, Koromani F, Rivadeneira F, Zillikens MC, Oei EH. Quantitative imaging methods in osteoporosis. Quant Imaging Med Surg 2016;6:680-98. [Crossref] [PubMed]
- Setiawati R, Di Chio F, Rahardjo P, Nasuto M, Dimpudus FJ, Guglielmi G. Quantitative Assessment of Abdominal Aortic Calcifications Using Lateral Lumbar Radiograph, Dual-Energy X-ray Absorptiometry, and Quantitative Computed Tomography of the Spine. J Clin Densitom 2016;19:242-9. [Crossref] [PubMed]
- Geusens P, Lems WF. Osteoimmunology and osteoporosis. Arthritis Res Ther 2011;13:242. [Crossref] [PubMed]
- De Nijs RN. Glucocorticoid-induced osteoporosis: a review on pathophysiology and treatment options. Minerva Med 2008;99:23-43. [PubMed]
- van Staa TP, Leufkens HG, Cooper C. The epidemiology of corticosteroid-induced osteoporosis: a meta-analysis. Osteoporos Int 2002;13:777-87. [Crossref] [PubMed]
- Peel NF, Moore DJ, Barrington NA, Bax DE, Eastell R. Risk of vertebral fracture and relationship to bone mineral density in steroid treated rheumatoid arthritis. Ann Rheum Dis 1995;54:801-6. [Crossref] [PubMed]
- Engelke K, Adams JE, Armbrecht G, Augat P, Bogado CE, Bouxsein ML, Felsenberg D, Ito M, Prevrhal S, Hans DB, Lewiecki EM. Clinical use of quantitative computed tomography and peripheral quantitative computed tomography in the management of osteoporosis in adults: the 2007 ISCD Official Positions. J Clin Densitom 2008;11:123-62. [Crossref] [PubMed]
- Brett AD, Brown JK. Quantitative computed tomography and opportunistic bone density screening by dual use of computed tomography scans. J Korean Neurosurg Soc 2015;3:178-84.
- Kalender WA. Effective dose values in bone mineral measurements by photon absorptiometry and computed tomography. Osteoporos Int 1992;2:82-7. [Crossref] [PubMed]
- Link TM, Lang TF. Axial QCT: clinical applications and new developments. J Clin Densitom 2014;17:438-48. [Crossref] [PubMed]
- Węgierska M, Dura M, Blumfield E, Żuchowski P, Waszczak M, Jeka S. Osteoporosis diagnostics in patients with rheumatoid arthritis. Reumatologia 2016;54:29-34. [Crossref] [PubMed]
- Binkley TL, Specker BL. pQCT measurement of bone parameters in young children: validation of technique. J Clin Densitom 2000;3:9-14. [Crossref] [PubMed]
- Langton CM, Palmer SB, Porter RW. The measurement of broadband ultrasonic attenuation in cancellous bone. Eng Med 1984;13:89-91. [Crossref] [PubMed]
- Prins SH, Jorgensen HL, Jorgensen LV, Hassager C. The role of quantitative ultrasound in the assessment of bone: a review. Clin Physiol 1998;18:3-17. [Crossref] [PubMed]
- D’Elia G, Caracchini G, Cavalli L, Innocenti P. Bone fragility and imaging techniques. Clin Cases Miner Bone Metab 2009;6:234-46. [PubMed]
- Knapp KM. Quantitative ultrasound and bone health. Salud Publica Mex 2009;51 Suppl 1:S18-24. [Crossref] [PubMed]
- Guglielmi G, de Terlizzi F. Quantitative ultrasond in the assessment of osteoporosis. Eur J Radiol 2009;71:425-31. [Crossref] [PubMed]
- Baroncelli GI. Quantitative Ultrasound Methods to Assess Bone Mineral Status in Children: Technical Characteristics, Performance, and Clinical Application. Pediatr Res 2008;63:220. [Crossref] [PubMed]
- Hans D, Fuerst T, Duboeuf F. Quantitative ultrasound bone measurement. Eur Radiol 1997;7 Suppl 2:S43-50. [Crossref] [PubMed]
- Danese RD, Licata AA. Ultrasound of the skeleton: review of its clinical applications and pitfalls. Curr Rheumatol Rep 2001;3:245-8. [Crossref] [PubMed]
- Trimpou P, Bosaeus I, Bengtsson BA, Landin-Wilhelmsen K. High correlation between quantitative ultrasound and DXA during 7 years of follow-up. Eur J Radiol 2010;73:360-4. [Crossref] [PubMed]
- Hans D, Njeh CF, Genant HK, Meunier PJ. Quantitative ultrasound in bone status assessment. Rev Rhum Engl Ed 1998;65:489-98. [PubMed]
- Gluer CC, Wu CY, Jergas M, Goldstein SA, Genant HK. Three quantitative ultrasound parameters reflect bone structure. Calcif Tissue Int 1994;55:46-52. [Crossref] [PubMed]
- Toyras J, Nieminen MT, Kroger H, Jurvelin JS. Bone mineral density, ultrasound velocity, and broadband attenuation predict mechanical properties of trabecular bone differently. Bone 2002;31:503-7. [Crossref] [PubMed]
- Njeh CF, Kuo CW, Langton CM, Atrah HI, Boivin CM. Prediction of Human Femoral Bone Strength Using Ultrasound Velocity and BMD: An In Vitro Study. Osteoporos Int 1997;7:471-7. [Crossref] [PubMed]
- Wuster C, Heilmann P, Pereira-Lima J, Schlegel J, Anstatt K, Soballa T. Quantitative ultrasonometry (QUS) for the evaluation of osteoporosis risk: reference data for various measurement sites, limitations and application possibilities. Exp Clin Endocrinol Diabetes 1998;106:277-88. [Crossref] [PubMed]
- Njeh CF, Boivin CM, Gough A, Hans D, Srivastav SK, Bulmer N, Devlin J, Emery P. Evaluation of Finger Ultrasound in the Assessment of Bone Status with Application of Rheumatoid Arthritis. Osteoporos Int 1999;9:82-90. [Crossref] [PubMed]
- Leszczynski P, Lacki JK, Mackiewicz SH. Quantitative ultrasound densitometry (QUS) and dual X-ray densitometry (DXA) in patients with rheumatoid arthritis. Przegl Lek 2000;57:100-2. [PubMed]
- Haugeberg G, Orstavik RE, Uhlig T, Falch JA, Halse JI, Kvien TK. Comparison of ultrasound and X-ray absorptiometry bone measurements in a case control study of female rheumatoid arthritis patients and randomly selected subjects in the population. Osteoporos Int 2003;14:312-9. [Crossref] [PubMed]
- Orstavik RE, Haugeberg G, Uhlig T, Mowinckel P, Kvien TK, Falch JA, Halse JI. Quantitative ultrasound and bone mineral density: Discriminatory ability in patients with rheumatoid arthritis and controls with and without vertebral deformities. Ann Rheum Dis 2004;63:945-51. [Crossref] [PubMed]
- Cryer JR, Otter SJ, Bowen CJ. Use of quantitative ultrasound scans of the calcaneus to diagnose osteoporosis in patients with rheumatoid arthritis. J Am Podiatr Med Assoc 2007;97:108-14. [Crossref] [PubMed]
- Sambrook P, Raj A, Hunter D, Naganathan V, Mason R, Robinson B. Osteoporosis with low dose corticosteroids: contribution of underlying disease effects and discriminatory ability of ultrasound versus bone densitometry. J Rheumatol 2001;28:1063-7. [PubMed]
- Seriolo B, Paolino S, Sulli A, Ferretti V, Cutolo M. Bone Metabolism Changes During Anti-TNF-α Therapy in Patients with Active Rheumatoid Arthritis. Ann N Y Acad Sci 2006;1069:420-7. [Crossref] [PubMed]
- Birkett V, Ring EF, Elvins DM, Taylor G, Bhalla AK. A comparison of bone loss in early and late rheumatoid arthritis using quantitative phalangeal ultrasound. Clin Rheumatol 2003;22:203-7. [Crossref] [PubMed]
- Kavaja G, Xinxo S. Bone mineral density in patients with active rheumatoid arthritis, the relation between generalized osteoporosis and disease activity. Osteoporos Int 2010;21:S62.
- Pye SR, Marshall T, Gaffney K, Luben R, Khaw KT, Silman AJ, Symmons DP, O'Neill TW. Influence of inflammatory polyarthritis on quantitative heel ultrasound measurements. BMC Musculoskelet Disord 2012;13:133. [Crossref] [PubMed]
- Alenfeld FE, Diessel E, Brezger M, Sieper J, Felsenberg D, Braun J. Detailed analyses of periarticular osteoporosis in rheumatoid arthritis. Osteoporos Int 2000;11:400-7. [Crossref] [PubMed]
- Röben P, Barkmann R, Ullrich S, Gause A, Heller M, Glüer CC. Assessment of phalangeal bone loss in patients with rheumatoid arthritis by quantitative ultrasound. Ann Rheum Dis 2001;60:670-7. [Crossref] [PubMed]
- Daragon A, Krzanowska K, Vittecoq O, Menard JF, Hau I, Jouen-Beades F, Lesage C, Bertho JM, Tron F, Le Loet X. Prospective X-ray densitometry and ultrasonography study of the hand bones of patients with rheumatoid arthritis of recent onset. Joint Bone Spine 2001;68:34-42. [Crossref] [PubMed]
- Madsen OR, Suetta C, Egsmose C, Lorentzen JS, Sørensen OH. Bone status in rheumatoid arthritis assessed at peripheral sites by three different quantitative ultrasound devices. Clin Rheumatol 2004;23:324-9. [Crossref] [PubMed]
- Simoes E, Cardoso A, Cunha-Miranda L, Fernandes R, Cristovam T. Soul study - Sreening osteoporosis by FRAX(R) and ultrasound. Osteoporos Int 2010;21:S47.
- Filho JC, Pinheiro MM, de Moura Castro CH, Szejnfeld VL. Prevalence and risk factors associated with low-impact fractures in men with rheumatoid arthritis. Clin Rheumatol 2014;33:1389-95. [Crossref] [PubMed]
- Hartman C, Shamir R, Eshach-Adiv O, Iosilevsky G, Brik R. Assessment of osteoporosis by quantitative ultrasound versus dual energy X-ray absorptiometry in children with chronic rheumatic diseases. J Rheumatol 2004;31:981-5. [PubMed]
- Njeh CF, Shaw N, Gardner-Medwin JM, Boivin CM, Southwood TR. Use of quantitative ultrasound to assess bone status in children with juvenile idiopathic arthritis: a pilot study. J Clin Densitom 2000;3:251-60. [Crossref] [PubMed]
- Falcini F, Bindi G, Ermini M, Galluzzi F, Poggi G, Rossi S, Masi L, Cimaz R, Brandi ML. Comparison of quantitative calcaneal ultrasound and dual energy X-ray absorptiometry in the evaluation of osteoporotic risk in children with chronic rheumatic diseases. Calcif Tissue Int 2000;67:19-23. [Crossref] [PubMed]
- Fernanda F, Stefano S, Loredana C, Laura M, Serena C, Lorenzo C, Marco MC, Luisa BM. Longitudinal evaluation of bone mass in adolescents and young adults with juvenile idiopathic arthritis: The role of bone mass determinants in a large cohort of patients. Pediatric Rheumatology Conference: 18th Pediatric Rheumatology European Society, PReS Congress Bruges Belgium Conference Publication, 2011;9.
- Simonini G, Giani T, Stagi S, de Martino M, Falcini F. Bone status over 1 yr of etanercept treatment in juvenile idiopathic arthritis. Rheumatology 2005;44:777-80. [Crossref] [PubMed]
- Stagi S, Cavalli L, Masi L, Brandi ML, Cerinic MM, De Martino M, Falcini F. Bone mass and quality in juvenile idiopathic arthritis: Comparison of the role of bone mass determinants using DXA, PQCT and QUS. Horm Res Paediatr 2012;78:147.
- Kutilek S, Bayer M, Dolezalova P, Nemcova D. Quantitative ultrasonometry of the calcaneus in children with juvenile idiopathic arthritis. Rheumatology 2006;45:1273-5. [Crossref] [PubMed]
- Toussirot E, Michel F, Wendling D. Bone density, ultrasound measurements and body composition in early ankylosing spondylitis. Rheumatology 2001;40:882-8. [Crossref] [PubMed]
- Speden DJ, Calin AI, Ring FJ, Bhalla AK. Bone mineral density, calcaneal ultrasound, and bone turnover markers in women with ankylosing spondylitis. J Rheumatol 2002;29:516-21. [PubMed]
- Jansen TL, Aarts MH, Zanen S, Bruyn GA. Risk assessment for osteoporosis by quantitative ultrasound of the heel in ankylosing spondylitis. Clin Exp Rheumatol 2003;21:599-604. [PubMed]
- Lernbass I, Wutzl A, Grisar J, Schett G, Redlich K, Spitzauer S, Grampp S, Imhof H, Peterlik M, Pietschmann P. Quantitative ultrasound in the assessment of bone status of patients suffering from rheumatic diseases. Skeletal Radiol 2002;31:270-6. [Crossref] [PubMed]
- Stagi S, Cavalli L, Signorini C, Bertini F, Cerinic MM, Brandi ML, Falcini F. Bone mass and quality in patients with juvenile idiopathic arthritis: longitudinal evaluation of bone-mass determinants by using dual-energy x-ray absorptiometry, peripheral quantitative computed tomography, and quantitative ultrasonography. Arthritis Res Ther 2014;16:R83. [Crossref] [PubMed]
- Stagi S, Cavalli L, Bertini F, Matucci Cerinic M, Luisa Brandi M, Falcini F. Cross-sectional and longitudinal evaluation of bone mass and quality in children and young adults with juvenile onset systemic lupus erythematosus (JSLE): role of bone mass determinants analyzed by DXA, PQCT and QUS. Lupus 2014;23:57-68. [Crossref] [PubMed]
- Stagi S, Cavalli L, Bertini F, Signorini C, Matucci Cerinic M, de Martino M, Brandi ML, Falcini F. Comparison of bone mass and quality determinants in adolescents and young adults with juvenile systemic lupus erythematosus (JSLE) and juvenile idiopathic arthritis (JIA). Lupus 2014;23:1392-406. [Crossref] [PubMed]
- Frediani B, Allegri A, Falsetti P, Storri L, Bisogno S, Baldi F, Filipponi P, Marcolongo R. Bone mineral density in patients with psoriatic arthritis. J Rheumatol 2001;28:138-43. [PubMed]
- Frediani B, Falsetti P, Baldi F, Acciai C, Filippou G, Marcolongo R. Effects of 4-year treatment with once-weekly clodronate on prevention of corticosteroid-induced bone loss and fractures in patients with arthritis: Evaluation with dual-energy X-ray absorptiometry and quantitative ultrasound. Bone 2003;33:575-81. [Crossref] [PubMed]
- Frediani B, Baldi F, Falsetti P, Acciai C, Filippou G, Spreafico A, Siagri C, Chellini F, Capperucci C, Filipponi P, Galeazzi M, Marcolongo R. Clinical determinants of bone mass and bone ultrasonometry in patients with systemic sclerosis. Clin Exp Rheumatol 2004;22:313-8. [PubMed]
- Moayyeri A, Adams JE, Adler RA, Krieg MA, Hans D, Compston J, Lewiecki EM. Quantitative ultrasound of the heel and fracture risk assessment: an updated meta-analysis. Osteoporos Int 2012;23:143-53. [Crossref] [PubMed]
- Krieg MA, Barkmann R, Gonnelli S, Stewart A, Bauer DC, Del Rio Barquero L, Kaufman JJ, Lorenc R, Miller PD, Olszynski WP, Poiana C, Schott AM, Lewiecki EM, Hans D. Quantitative ultrasound in the management of osteoporosis: the 2007 ISCD Official Positions. J Clin Densitom 2008;11:163-87. [Crossref] [PubMed]
- Leib ES, Lewiecki EM, Binkley N, Hamdy RC. Official positions of the International Society for Clinical Densitometry. J Clin Densitom 2004;7:1-6. [Crossref] [PubMed]