Functional dynamic radiography with computer analysis—for physiological chest imaging and kinematic joint imaging
Introduction
My colleagues and I have developed a screening examination that provides kinetic data through the use of a dynamic X-ray flat-panel detector (FPD) (Figure 1) in our laboratory. This new examination is not based on conventional X-ray fluoroscopy for diagnostic examinations but rather based on functional dynamic radiography (fDR) with the “addition of physiological and functional data that are subjected to computer analysis,” which is similar to systems generally used in initial examinations. Conventional screening examinations (initial examinations) require subjects to “hold their breath” and “keep still,” which results in only a limited amount of diagnostic data being obtained. However, considering major developments in image processing and the digital radiography system, we believe that it is possible to develop an innovative screening system that can provide new diagnostic information. Hereby I provide an overview of the development of this screening system from the initial stages as well as the results of currently ongoing clinical trials. In addition, I provide a summary of reports by other researchers that are related to this research.
Devices and image acquisition techniques utilized
The following systems were used for X-ray imaging: (I) A Canon FPD imaging system (CXDI series) was modified so that it could take sequential images at either 7.5 frames/s (fps) for 5 s or 3 fps for 10 s. The matrix size of the images was 2,688×2,688, and the grayscale was 12 bit; (II) A Konica Minolta Prototype FPD system was used to take sequential images at a frame rate of 15 fps for 10 s; the matrix size of the image was 1,024×1,024, and the grayscale was 16 bit.
The imaging parameters were set so that the radiation exposure to subjects when obtaining dynamic chest radiographs would not exceed that of conventional chest X-rays (front + lateral). The entrance surface dose for the detector is approximately 1.9 mGy, which is comparable to the dose limit recommended by the International Atomic Energy Agency (IAEA) for both front (0.4 mGy) and lateral (1.5 mGy) projections (1,2). In addition, the imaging parameters of the joints of extremities were set so that exposure would not be two times higher than that what is required for conventional X-ray imaging at those sites. For instance, for hip joints, the entrance surface dose for the detector is approximately 5.11 mGy, which is less than two times the dose (8 mGy) required for conventional X-ray imaging and the dose limit (10 mGy) recommended by the IAEA (1,3). This study was conducted with the approval of the Ethics Review Board of Kanazawa University. All subjects were provided with full explanations of the study in both written form and orally, and their written consent was obtained.
Morphological analysis of the function of the joints of extremities
When examining the joints of extremities, it is extremely important to assess motor function. However, nearly all current medical imaging makes use of initial diagnostic imaging based on morphological data using static X-ray images (front, lateral, under stress). Although diagnostic examinations utilize CT and MRI to perform differential diagnoses using 3D anatomical data of soft tissues such as cartilages, tendons, and articular disks, in many cases, subjects are instructed to keep still during the imaging process.
With the introduction of the FPD system, it would not be an exaggeration to say that X-ray imaging has entered the age of the X-ray digital camera and is not unlike a consumer digital camera in convenience and usability. Specifically, recording and performing functional analysis of “movements” of great interest using dynamic imaging as well as recording and assessing 3D “morphologies” that are difficult to visualize (e.g., tomosynthesis) are no longer technically difficult. Below are examples of kinetic examinations and analytical methods performed using dynamic FPD.
Initially, we developed a kinetic X-ray imaging technique and a functional analysis method to quantitatively assess the movement of the temporomandibular joint (TMJ) (Figure 2) (4,5). This joint was chosen because of the high prevalence of TMJ disorders, particularly among young individuals, and the lack of quantitative evaluation in diagnosis and treatment in such cases (6). This system is useful in TMJ screening examinations and diagnostic observations, and preoperative informed consent because it is easy to understand anomalies on dynamic radiographs. Although other studies have reported attempts to assess the TMJ using tracking systems with optical markers and MRI because these are considered as diagnostic examinations, they are expensive, complex to use, and require a long examination time; as a result, they have not been successfully used in clinical practice (7,8).
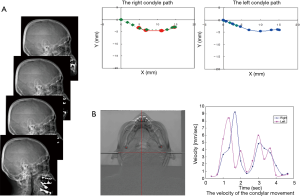
Sakuda et al. developed a sequential X-ray imaging technique and a functional analysis technique for the quantitative assessment of shoulder joint movement (Figure 3) (9). During arm elevation, shoulder joint movement displays a characteristic rhythm. Specifically, the angle between the humerus and the scapula (glenohumeral angle) and the angle between the scapula and the axis of the thorax (scapulothoracic angle) are normally in a fixed 2:1 ratio regardless of the angle of arm elevation (scapulohumeral rhythm: G/S). Changes in this rhythm are useful in discovering abnormalities in the shoulder joint and assessing severity. Therefore, this method is effective in assisting therapeutic strategy determination and monitoring therapeutic progress. The total exposure dose was almost equivalent to that of the two projections in conventional shoulder imaging (0.4 mGy) (9).
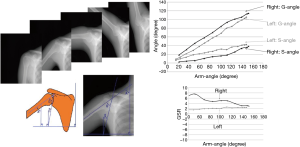
There are many reasons for performing orthopedic assessments of shoulder kinetics, and the use of various modalities has been reported. For example, in their study on an X-ray TV system, Inman et al. reported that the field of view (FOV) is narrow and that the analytical accuracy is limited due to poor image quality (10). In their attempt to perform highly accurate assessments using MRI, Roberts et al. reported that because the procedure is time consuming and expensive, it is not clinically practical (11). Although sonographic imaging techniques are simple, because the FOV is narrow, the ability to assess large joint kinetics is limited (12). There have been multiple reports on methods of measuring kinetics of joint from the surface of the body using videometers and goniometers; however, these reports are limited by their inability to directly assess and analyze intra-articular structures (13-15).
Kawashima et al. developed a sequential X-ray imaging technique and a functional analysis technique to quantitatively assess wrist movement (Figure 4) (16). This study elucidated that changes in the angle between the radiocarpal joint and the midcarpal joint during palmar-dorsiflexion of the wrist have a certain rhythm between these two joints. Their technique can be used not only for quantitative assessments as part of a screening examination but also for pre- and postoperative assessments of recovery. In dynamic imaging, the exposure dose was approximately 0.77 mGy, which is almost 3.5 times the dose for lateral radiography of the wrist joint. Because of advances in FPD technology, the exposure dose has decreased by half (16).
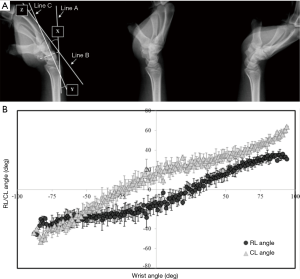
The importance of kinetic analysis of the wrist has been reported in numerous studies, and in recent years, the usefulness of 3D kinetic analysis using CT has been recognized (17,18). However, considering subjects’ exposure to X-rays and the cost, it is difficult to utilize this method as a screening examination in spite of the excellent diagnostic examination. The ideal methodology would be to use sequential X-ray images to first conduct an overview of the kinetics and, if any abnormalities are found, to conduct diagnostic examinations using CT and MRI.
In the field of orthopedics, sequential X-ray imaging has already been shown to be extremely useful in the kinetic analysis of the lumbar and hip joints (3,19). Considering the aging of society, these motor function examinations are expected to be useful in the early assessment of locomotive syndrome, prevention of overload during recreational sports, and other types of simple orthopedic kinetic screening examinations. Furthermore, there is the potential for use in the examination of trauma patients in the fields of emergency medicine and disaster medicine.
Analysis of the physiology and function of the chest using density data
Frontal (anteroposterior) chest X-ray imaging is the most important screening examination among all X-ray examinations. This is because cardiopulmonary images provide diagnostic data that allow an overview of a patient’s general condition. When dynamically imaging the chest, at first, sequential changes caused by respiratory movements, such as movements of the diaphragm, bony thorax, intrapulmonary structures, and cardiac walls, can be analyzed and assessed morphologically. Imaging is done using nearly the same method as conventional frontal chest X-rays, and images a subject’s respiratory process in the order of maximum inspiration to maximum expiration and again to maximum inspiration are obtained. Based on these X-ray images, the degree of diaphragmatic elevation and right–left symmetry, among other parameters, can be analyzed, and as a result, useful diagnostic data can be obtained (20).
Tanaka et al. elucidated that the most important discovery during kinetic chest X-ray examinations is the fact that changes in X-ray translucency (density of black and white) at sites within the lung field during respiration are related to the status of lung ventilation in those sites (2,21-23). When examining density data in the lung field (sequential changes in pixel values), for example, the case shown in Figure 5, synchronized coronal CT images clearly show bilateral bullous emphysema in the same locations and areas of loss can be seen as changes in the density of sequential X-ray images (lower two-stage sequential images). In normal cases, such areas of loss and sites with irregular density changes are not observed. In other words, in intrapulmonary sites where there are no abnormalities in ventilation, density changes are not seen. In normal intrapulmonary sites, X-ray translucency increases during the inspiratory phase and images become darker and translucency decreases during the expiratory phase and images become lighter. This is because the density per unit volume of intrapulmonary structures, such as blood vessels and bronchi, at any given site decreases during the inspiratory phase and increases during the expiratory phase.
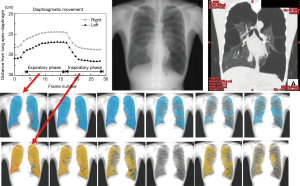
For dynamic chest radiography, spatial and temporal resolutions and exposure dose have to be balanced using optimal system settings. The imaging rate should be greater than 7.5 frames per second (fps) for an accurate evaluation of cardiac function. For respiratory function, an imaging rate lower than 7.5 fps is acceptable, considering the exposure dose. The entrance surface dose for the detector was approximately 1.9 mGy for 14 s imaging performed at 120 kV, 50 mA, 2.5 ms, 15 fps, and 2.0 m source-image receptor distance. Digital image parameters were 2,688×2,688 matrix, 160 micron pixels, and 4,096 gray level (2).
The pulse component, which was originally thought to have a negative influence on the analysis of respiration kinetics, is now known to be related to heart rate, and it was found that relative blood flow distribution could be visualized, as shown in Figure 6. Specifically, it is now known that minute changes in X-ray translucency that occur when the blood that has entered the heart and lung sites is expelled from those sites is reflected in translucency data for target sites.
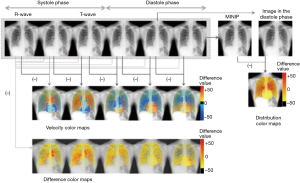
Currently, we are investigating examination and analysis techniques for chest respiratory and circulatory dynamics in animal (adult pigs) experiments, and we are using analytical results to investigate the accuracy and reproducibility of these techniques. We plan to release our results in the near future. Currently, such fDR with computer analysis has been developed only in our laboratory. Few other facilities have initiated clinical trials in Japan since June 2017 using dynamic X-ray imaging systems from Konica-Minolta, Inc.
Conclusions
We have developed an X-ray dynamic imaging technique and a computer image analysis technique as a general initial examination (screening examination) that is able to easily assess respiratory and circulatory physiology and dynamic function. As a result of initial tests on a total of over 100 subjects, we found that additional diagnostic information, such as air trapping in emphysema, anomalistic movement of lung texture in fibrosis, pleural adhesions, and diaphragm kinetics, which could not be obtained using conventional screening examination techniques that rely on still images, could be easily obtained with a relatively high degree of accuracy (2,23). In particular, these results suggest that fDR is useful in monitoring respiratory and circulatory functions in intensive care units during postoperative examinations and emergency examinations using a portable dynamic radiography system (2).
Identifying these techniques as a type of general initial examination (screening examination) using kinetic radiography will allow physicians to obtain more diagnostic data than conventional screening examinations using a method that is far simpler than that used in diagnostic examinations (Figure 7), i.e., these techniques are cost-effective and requires only two times the dose. For example, kinetic and functional data obtained using fDR can provide beneficial diagnostic data that can be used to supplement 3D anatomical data obtained via CT and tissue anatomy data obtained via MRI. Morphological data obtained via X-ray static images are unable to predominate CT 3D data on their own. When performing chest examinations, fDR provides site-specific pulmonary ventilation data that cannot be provided by spirometry, which is the “gold standard” for pulmonary ventilation assessments. Thus, fDR may be an alternative to nuclear medicine, which provides spatial ventilation and blood flow data.
In addition, fDR allows a great deal of temporal and spatial freedom when performing general imaging. In other words, fDR has a high tolerance for adjusting patient positioning and X-ray projection, which have to be strictly controlled when utilizing static imaging. We believe that there is a high degree of latent potential for the development of self-imaging and autonomous imaging using radiography navigation systems.
Acknowledgements
The contents of this review article include the results of the study with physicians and radiological technologists in the Kanazawa University Department of Radiology, Department of Pulmonary Medicine and Surgery, Department of Dental Surgery, and Department of Orthopedic Surgery as well as members of my research laboratory (Rie Tanaka, PhD and all alumni and alumnae). I would like to express my appreciation to all of them.
I would also like to express my deep appreciation to Professor Tohru Tani, MD, PhD of Shiga University of Medical Science, who kindly provided encouragement from the beginning of this study and is currently assisting the animal experiments as well as the physicians in the Departments of Surgery, Radiology, and Emergency and Intensive Medicine.
Finally, I would like to express my appreciation to Canon, Inc., who provided the kinetic X-ray device and technical support at the start of this study, as well as Konica-Minolta, Inc. and its technicians for their cooperation in a joint study with us on the precision of the technique and this device that is currently under way and for their assistance with its release as a clinical device.
Funding: This study was partially funded by the Ministry of Education, Culture, Sports, Science, and Technology Grants-in-aid for Scientific Research (Project ID #23602006, #26460726) and by the Fukushima Medical and Assistive Device (medical devices for emergencies and disasters; Project term, 2014–2017) developmental project grant. Support was provided by the C&C Foundation for the release of these study results.
Footnote
Conflicts of Interest: The author has no conflicts of interest to declare.
References
- IAEA, 1996. International Basic safety Standard for Protection against Ionizing Radiation and for the Safety of Radiation Source. Safety Series No.115.
- Tanaka R. Dynamic chest radiography: flat-panel detector (FPD) based functional X-ray imaging. Radiol Phys Technol 2016;9:139-53. [Crossref] [PubMed]
- Kawashima H, Kajino Y, Kabata T, Tsuchiya H, Sanada S, Ichikawa K. Kinematic radiography of the hip joint after hip resurfacing arthroplasty. Radiol Phys Technol 2016;9:254-60. [Crossref] [PubMed]
- Bandai N, Sanada S, Ueki K, Funabasama S, Matsui T, Tsuduki S. Kinetic radiography and functional analysis of the temporomandibular joint (TMJ). Nihon Hoshasen Gijutsu Gakkai Zasshi 2003;59:416-22. [Crossref] [PubMed]
- Bandai N, Sanada S, Ueki K, Funabasama S, Tsuduki S, Matsui T. Morphological analysis for kinetic X-ray images of the temporomandibular joint. Nihon Hoshasen Gijutsu Gakkai Zasshi 2003;59:951-7. [Crossref] [PubMed]
- Goldstein BH. Temporomandibular disorders: a review of current understanding. Oral Surg Oral Med Oral Pathol Oral Radiol Endod 1999;88:379-85. [Crossref] [PubMed]
- Theusner J, Plesh O, Curtis DA, Hutton JE. Axiographic tracings of temporomandibular joint movements. J Prosthet Dent 1993;69:209-15. [Crossref] [PubMed]
- Nebbe B, Major PW, Prasad NG, Hatcher D. Quantitative assessment of temporomandibular joint disk status. Oral Surg Oral Med Oral Pathol Oral Radiol Endod 1998;85:598-607. [Crossref] [PubMed]
- Sakuda K, Sanada S, Tanaka R, Kitaoka K, Hayashi N, Matsuura Y. Functional shoulder radiography with use of a dynamic flat panel detector. Radiol Phys Technol 2014;7:254-61. [Crossref] [PubMed]
- Inman VT, Saunders JB, Abbott LC. Observations of the function of the shoulder joint. Clin Orthop Relat Res 1996.3-12. [Crossref] [PubMed]
- Roberts CS, Davila JN, Hushek SG, Tillett ED, Corrigan TM. Magnetic resonance imaging analysis of the subacromial space in the impingement sign positions. J Shoulder Elbow Surg 2002;11:595-9. [Crossref] [PubMed]
- Desmeules F, Minville L, Riederer B, Côté CH, Frémont P. Acromio-humeral distance variation measure by ultrasonography and its association with the outcome of rehabilitation for shoulder impingement syndrome. Clin J Sport Med 2004;14:197-205. [Crossref] [PubMed]
- Bagg SD, Forrest WJ. A biomechanical analysis of scapular rotation during arm abduction in the scapular plane. Am J Phys Med Rehabil 1988;67:238-45. [PubMed]
- Johnson MP, McClure PW, Karduna AR. New method to assess scapular upward rotation in subjects with shoulder pathology. J Orthop Sports Phys Ther 2001;31:81-9. [Crossref] [PubMed]
- Roux L, Hanneton S, Roby-Brami A. Shoulder movements during the initial phase of learning manual wheelchair propulsion in able-bodied subjects. Clin Biomech (Bristol, Avon) 2006;21 Suppl 1:S45-51. [Crossref] [PubMed]
- Kawashima H, Tada K, Suganuma S, Tsuchiya H, Sanada S. Wrist rhythm during wrist joint motion evaluated by dynamic radiography. Hand Surg 2014;19:343-7. [Crossref] [PubMed]
- Wolfe SW, Neu C, Crisco JJ. In vivo scaphoid, lunate, and capitate kinematics in flexion and in extension J Hand Surg Am 2000;25:860-9. [Crossref] [PubMed]
- Moojen TM, Snel JG, Ritt MJ, Venema HW, Kauer JM, Bos KE. In vivo analysis of carpal kinematics and comparative review of the literature. J Hand Surg Am 2003;28:81-7. [Crossref] [PubMed]
- Shimada K, Kawashima H, Yoshioka K, Sanada S. Motion Analysis of Lumbar Spine and Hip Joint on Sequential Radiographs Acquired with a Dynamic Flat-panel Detector (FPD) System. Nihon Hoshasen Gijutsu Gakkai Zasshi 2015;71:1174-9. [Crossref] [PubMed]
- Tanaka R, Sanada S, Suzuki M, Matsui T, Uoyama Y. New method of screening chest radiography with computer analysis of respiratory kinetics. Nihon Hoshasen Gijutsu Gakkai Zasshi 2002;58:665-9. [Crossref] [PubMed]
- Tanaka R, Sanada S, Suzuki M, Matsui T, Uoyama Y. New screening chest radiography with computer analysis of pulmonary marking movement and change in regional density. Nihon Hoshasen Gijutsu Gakkai Zasshi 2002;58:1489-96. [Crossref] [PubMed]
- Sanada S, Tanaka R, Kobayashi T, Suzuki M, Inoue H. Screening chest X-ray examination with kinetic analysis using flat-panel detector. Nihon Igaku Hoshasen Gakkai Zasshi 2003;63:579-81. [PubMed]
- Tanaka R, Sanada S, Kobayashi T, Matsui T, Inoue H, Yoshihisa N. Breathing chest radiography using a dynamic flat-panel detector (FPD) combined with computer analysis. Medical Physics 2004;31:2254-62. [Crossref] [PubMed]