Quantitative assessment of biomechanical changes in oral lesions at different cancerous stages using optical coherence elastography
Introduction
Oral cancer is the 6th most common cancer worldwide, and due to its extremely malignant nature, it has a 5-year mortality rate of 50% (1,2). Oral squamous cell carcinoma (OSCC) is the most common type of oral cancer, and generally develops from precursor lesions of oral potentially malignant disorders (OPMDs) (3). Most patients experience a long phase of OPMDs before being diagnosed with cancer, and may not present with obvious symptoms that could aid in early diagnosis (4,5).
Biopsy and histopathologic evaluation are cornerstones in the diagnosis of OPMDs and OSCC (6). Nevertheless, the invasive nature and lengthy detection procedure of biopsy limit its application in long-term monitoring and early diagnosis (7). Imaging examinations such as magnetic resonance imaging or ultrasonography are minimally invasive and can enable early cancer detection; however, these techniques have a number of shortcomings, such as high costs, limited resolution, and tissue contrast that may be insufficient for the accurate identification of oral lesions (8-12). Photographic medical imaging is a cost-effective and rapid oral screening approach, and artificial intelligence systems have achieved detection rates of 82–89% (13,14). However, three key barriers hinder the clinical adoption of such systems: operator-dependent variability (>15% diagnostic inconsistency due to lighting/angular differences), a lack of standardized quantitative biomarkers, and demanding protocol standardization requirements (13,14). Many emerging techniques have been proposed to address these shortcomings, such as fluorescence imaging and confocal microscopy, but these techniques usually pose challenges such as the need for exogenous contrast materials, complicated handling, and concerns related to user safety (15-17).
At present, several potential challenges arise during the screening and early diagnosis of OPMDs and OSCC. Optical coherence tomography (OCT) is an imaging technology that has several advantages, such as a high resolution (2–10 µm) and imaging depth (1–2 mm), good sensitivity, non-radiation damaging properties, and real-time detection (18-20). OCT has shown significant potential in oral cancer animal models for detecting disease-induced tissue alterations; however, it primarily provides structural contrast (21), and several notable limitations persist when applying OCT in clinical practice. For example, the typical penetration depth of OCT systems in oral tissues is limited to 1–2 mm, which is insufficient for evaluating deep tumor margins in advanced cases (e.g., lesions invading the submucosal or muscular layers) (22,23). Additionally, signal attenuation caused by highly keratinized regions (e.g., the dorsal tongue surface), saliva, and blood contamination reduces diagnostic accuracy by 15–20% (24,25). Our research team sought to improve the application of OCT by enhancing its contrast through the examination of the mechanical characteristics of tissues. As an elastography technique that can enable the evaluation of the biomechanical characteristics of tissues, optical coherence elastography (OCE) can be coupled to record qualitative or quantitative measurements of tissue stiffness (26,27).
In the present study, we established a shaker-based OCE (shaker-OCE) system for quantitative measurements of mechanical properties of tissues. We aimed to monitor the variability in the biomechanical course of OSCC during lesion development using OCE, and then to explore the correlation between changes in soft tissue elasticity and the degree of tissue degeneration in an oral cancer model. We present this article in accordance with the ARRIVE reporting checklist (available at https://qims.amegroups.com/article/view/10.21037/qims-24-2359/rc).
Methods
Animal model and protocol
Oral cancer was induced by spiking the drinking water of Sprague-Dawley (SD) rats with 4-nitroquinoline-1-oxide (4NQO). 4NQO, a water-soluble carcinogen, selectively targets oral mucosa, particularly in the tongue (28). 4NQO induces carcinogenesis through a relatively natural process, allowing for a more realistic simulation of the occurrence and development of human cancers (2,29). Therefore, it serves as a relatively ideal animal model. This is a relatively ideal animal model. The resulting changes in the course of carcinogenesis and morphological traits involving the gradual progression from hyperplasia and dysplasia to squamous cell carcinoma (SCC) contribute to the development of human OSCC (29,30). Experiments were performed under a project license (No. NCULAE-20240827001) granted by the Animal Ethics Committee of Nanchang University and in compliance with institutional guidelines for the care and use of animals.
In total, 45 male SD rats (5 weeks old) weighing about 200 g were purchased from GemPharmatech Co., Ltd. (Nanjing, China) [No. SCXK(SU) 2023-0009] for this study. Of the 45 rats, 35 were provided drinking water spiked with 100 µg/mL of water-soluble 4NQO (Sigma-Aldrich, St. Louis, MO, USA), and 10 control rats were not exposed to 4NQO. OCE imaging was performed at weeks 10, 12, 16, 24, and 32 following the initiation of the 4NQO treatment. At every scheduled time slot, two control and seven experimental rats were imaged (Figure 1A). The rats were anesthetized by an intraperitoneal injection of 40 mg/kg phenobarbital before being scanned. Their cheeks were cut open, and the tongue tip was carefully protruded and secured. Optical and mechanical properties were measured for each rat at three locations along the length of the tongue, and the average value of the measurements was employed to characterize the sample (Figure 1B,1C). The rats were sacrificed once the images were acquired, and their tongue tissues were excised, stained, and preserved for histological examination.
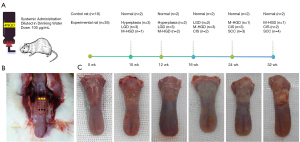
System setup
As Figure 2 shows, the shaker-OCE system comprises a swept-source OCT unit and an excitation unit. The swept-source OCT unit employs a scanning laser with the following properties: a center wavelength of 1,310 nm; an A-line rate of 50 kHz; and an average power of 20 mW. The optical fiber coupler divides the output light at a ratio of 90:10, such that 10% of the light enters the reference arm, and 90% enters the sample arm. After entering a 50:50 optical coupler, the interference signals from the sample and reference arms are reflected back and captured by a balanced photodetector (PDB480C-AC, Thorlabs, Newton, NJ, USA). The signal from the photodetector is collected via a waveform digitizer acquisition card (Alazar Technologies Inc., Quebec, Canada). The excitation unit is made up of a shaker exciter (Miniature Shaker Model 4810, Duluth, GA, USA), a power amplifier (PAS-00023-25, Spanawave, Roseville, CA, USA), and a function generator (AFG31102, Tektronix, Beaverton, OR, USA).

System synchronization
Figure 3 depicts the M-B scanning protocol used by the system. While acquiring elastic data from the sample, a single A-scan was conducted at position P0 to form an A-line, which requires 20 µs. This process is repeated 1,000 times to create an M-scan, resulting in a duration of 20 µs × 1,000 = 20 ms for one complete M-scan. Subsequently, a two-dimensional (2D) galvanometer is rotated to generate a scanning beam probe at position P1, followed by the execution of an identical M-scan. After performing 1,000 M-scans along the acquisition direction, a complete B-scan is ultimately obtained. One B-scan takes 20 ms × 1,000 = 20 s. Figure 3B displays a logical diagram of timing synchronization in the process of elasticity imaging, wherein the shaker excitation functions between 101st and 120th A-lines for a duration of 400 µs.
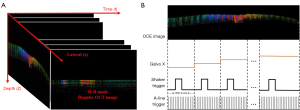
Data-processing process
As per the below equations, the axial phase shifts Δφ of two adjacent A-lines are determined once the raw data of the interfering signals from the sample tissue are processed by the system using a phase-resolved Doppler OCT algorithm (31):
where Fm and F*m+1 are conjugate complex numbers, and Im() and Re() indicate real and imaginary parts of the complex signals, respectively, at the acquisition point and the next acquisition point. Using the Doppler phase shift (32), the axial displacement of the tongue tissue induced by the excitation is then calculated using the following equation:
where is the laser’s central wavelength and n is the sample’s refractive index. The vibrational displacement can be used to reconstruct the x-t 2D image of Doppler OCT, where x is the propagation distance of the shear wave, and t is the propagation time of the shear wave in the acquisition direction. The ratio x-t can be used to calculate the velocity of the shear wave (33) and thus the shear modulus according to the following equation:
The parameters ρ, Vs, and µ correspond to the density of the tongue (assumed to be 1,000 kg/m3) (34), shear wave velocity, and shear modulus, respectively. Herein, Young’s modulus was employed to quantify biomechanical properties. The correlation between the shear modulus (µ) and Young’s modulus (E) (35) is expressed as follows:
The Poisson’s ratio of the biological tissue is estimated to be υ=0.5 (34,36). Therefore, Young’s modulus of the biological tissue can be expressed as follows:
Histopathology
The tissue samples were embedded in paraffin blocks and cut into 4-µm sections, such that the region lined up with the OCE B scanning position (the histology section). The tissue sections were treated with hematoxylin and eosin (HE) staining, Masson’s trichrome staining, and Gomori’s aldehyde-fuchsin (AF) staining for the immunohistochemical analysis. The experimenter was blinded to the experimental samples, and the reviewer was unaware of the group assignments during the histological analysis.
The HE staining of the tissue sections was performed for pathological gradation. OSCC staging was performed as per the Cancer Staging Manual (5th edition) of American Joint Committee on Cancer (37) as follows: (I) normal; (II) hyperplasia; (III) low-grade dysplasia (LGD); (IV) moderate-high-grade dysplasia (M-HGD); (V) carcinoma in situ (CIS); and (VI) SCC. The degree of collagen fiber expression per unit area was analyzed by Masson’s trichrome staining, while the dispersion of elastic fibers per unit area was observed using Gomori’s AF staining. Cancer-associated fibroblasts (CAFs) were detected through the immunohistochemical staining of alpha-smooth muscle actin (α-SMA). A semi-quantitative method was employed to determine the number of positive cells and to analyze the intensity of the α-SMA staining (38). Based on the method of Tuxhorn et al. (39), the immunostaining results were evaluated based on the intensity and percentage of α-SMA-positive cells. The percentage of the α-SMA-positive cells in the tumor stroma was scored as follows: 0, no positive cells; 1, 1–33% positive cells; 2, 34–66% positive cells; and 3, 67–100% positive cells. While staining was scored as follows: 0, no staining; 1, weak staining; 2, moderate staining; and 3, strong staining. The immunoreactivity score (IRS) was then calculated as follows: IRS = positive cell score × staining intensity score. The IRS was classified as follows: zero (0 points), low (1–2 points), moderate (3–4 points), and high (6–9 points) (40). Five images were randomly selected under a 10× field of a Zeiss high-magnification optical microscope (Vectra 3 PerkinElmer), and the images were analyzed using ImageJ 1.46 (National Institute of Health, Bethesda, MD, USA).
Statistical analysis
All the statistical analyses were performed using SPSS 27 software (SPSS, Inc., Chicago, IL, USA). The correlation between collagen or elastic fibers and the pathological grading of cancer was determined by Spearman correlation analysis. Changes in α-SMA expression in lesions at different stages were evaluated using cross-tabulation and Chi-squared test (also χ2 test). One-way analysis of variance and Tamhane’s T2 post-hoc multiple comparisons were used to determine differences in the shear modulus and Young’s modulus at different lesion stages. A (two-sided) P value <0.05 indicated statistical significance.
Results
Animal experiments and histological analysis results
The experimental results of 45 specimens at different time points are displayed in Figure 1. In total, the results showed that 10 samples were normal (Figure 4A, A1), five were hyperplastic (Figure 4A, A2), eight were LGDs (Figure 4A, A3), eight were M-HGDs (Figure 4A, A4), seven were CISs (Figure 4A, A5), and seven were SCCs (Figure 4A, A6).

Expression of collagen fibers at various stages of cancer progression
In the healthy tongue (Figure 4B, B1), the collagen fibers were bluish, thin, and long, and displayed a linear pattern through the lamina propria (LP), while the core connective tissue of the tongue papilla displayed very few collagen fibers. The collagen fibers of the hyperplastic (Figure 4B, B2) and LGD (Figure 4B, B3) tissues were not significantly different from those of the normal tissue. However, in the M-HGD (Figure 4B, B4) and CIS (Figure 4B, B5) samples, the collagen fibers were more slender in the core connective tissue, and the collagen fiber bundles in the LP of the mucous membrane were thick and densely arranged. The SCC samples (Figure 4B, B6) had a significantly higher number of collagen fibers than the other sample types that showed thick and dense deposition in the LP and tight fiber distribution. The Spearman correlation test results revealed a positive correlation between the expression of collagen fibers and tumor progression (r=0.353, P<0.05).
Expression of elastic fibers at various stages of cancer progression
In the healthy tongue tissue (Figure 4C, C1), the LP was enriched in AF-positive elastic fibers. The elastic fibers of the hyperplastic (Figure 4C, C2) and LGD (Figure 4C, C3) tissues had morphological characteristics similar to those of the normal tongue mucosa. In both the M-HGD (Figure 4C, C4) and CIS (Figure 4C, C5) tissue samples, the AF-positive elastic fibers of the LP were disrupted and shortened, and exhibited a diluted distribution. The LP of the SCC tissue sample (Figure 4C, C6) rarely showed AF-positive elastic fibers. The Spearman correlation test results revealed that the expression of the elastic fibers progressively declined as the malignancy of the tongue cancer progressed (r=−0.776, P<0.05).
Expression of α-SMA at various stages of cancer progression
As detailed in Table 1, no cancerous fibroblasts were observed in the stroma of the normal (Figure 4D, D1), hyperplasia (Figure 4D, D2), and LGD (Figure 4D, D3) specimens (which had scores of 0). Among the eight M-HGD (Figure 4D, D4) samples, six showed no cancerous fibroblasts (scores of 0) and two samples exhibited low α-SMA expression. Among the seven CIS (Figure 4D, D5) specimens, two showed no cancerous fibroblasts (scores of 0), four samples had low α-SMA expression, and one sample showed moderate α-SMA expression. All the SCC (Figure 4D, D6) samples exhibited varying degrees of α-SMA-positive staining. Specifically, one sample had a low score, five had moderate scores, and one had a high score. Based on the results of the χ2 test, the α-SMA scores of the CIS and SCC samples were significantly higher than those of the normal, simple hyperplasia, LGD, and M-HGD samples (P<0.05).
Table 1
Samples | Presence of α-SMA | |||
---|---|---|---|---|
Negative | Low | Middle | High | |
Normal (n=10) | 10† | 0† | 0† | 0† |
Hyperplasia (n=5) | 5† | 0† | 0† | 0† |
LGD (n=8) | 8† | 0† | 0† | 0† |
M-HGD (n=8) | 7† | 1†,‡ | 0† | 0† |
CIS (n=7) | 2† | 4‡ | 1†,‡ | 0† |
SCC (n=7) | 0† | 1†,‡ | 5‡ | 1† |
Different letters (†, ‡) in the same vertical column indicate a statistically significant difference in relation to other groups (P<0.05). α-SMA, alpha-smooth muscle actin; CIS, carcinoma in situ; LGD, low-grade dysplasia; M-HGD, moderate-high-grade dysplasia; SCC, squamous cell carcinoma.
OCT/OCE evaluation
OCT images of the normal oral epithelium tissue (Figure 5A, A1) showed clear mucosal layer contours with a visible keratinized layer (KL), epithelial layer (EP), LP, and basement membrane (BM). The BM was continuous and intact. Figure 5A, A2 shows the corresponding histopathologic image. The OCT images showed no significant differences between normal and hyperplastic tissues, and sometimes the KL seemed more luminous and reflective (Figure 5B, B1); Figure 5B, B2 shows the corresponding histopathological images. The LGD samples (Figure 5C, C1) displayed a wide layer that had a strong signal, thicker epithelium, clearly distinguishable epithelial and LP layers, and a visible BM; Figure 5C, C2 shows the corresponding histopathological images. OCT images of the M-HGD samples (Figure 5D, D1) were consistent with the histopathology findings (Figure 5D, D2), highlighting the significant thickening of the epithelium. The individual mucosal layers were sometimes indistinct, and the border between the EP and LP was no longer visible. Compared to the BM in the normal mucosa, during the development of SCC, the BM undergoes significant changes. In the OCT image of early CIS tissue (Figure 5E, E1), the BM could not be clearly identified due to severe epithelial dysplasia; Figure 5E, E2 shows the corresponding histopathology images. For the SCC samples (Figure 5F, F1), the epithelium showed varying degrees of thickness, and sometimes tended to be irregular, with areas of erosion and extensive downward growth and invasion into the sub-EP; Figure 5F, F2 shows the corresponding histopathological images.
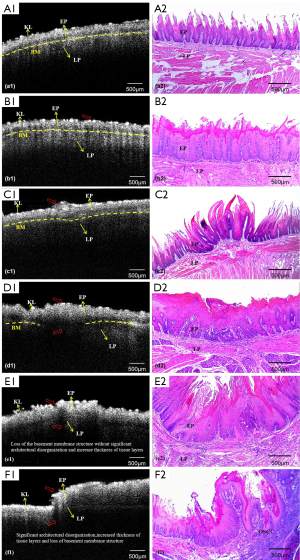
The M-B mode protocol was employed to successfully identify elastic waves inside the tissue. The images illustrated the 2D shear wave propagation in the normal tongue tissue (Figure 6A) and cancerous tongue tissue (Figure 6B) at six different time points. As the propagation distance increased, the amplitude gradually decreased. Notably, there were significant distinct differences between the healthy and cancerous tissues in terms of the speed of propagation and the amplitude decay rate of the elastic wave. We reconstructed the three-dimensional (3D) images to visualize the propagation of the shear wave across the entire transverse scanning range. The vibration amplitude of the elastic wave of the normal tissue (Figure 6C) exceeded that of the elastic wave of the cancerous tissue (Figure 6D). We produced spatio-temporal Doppler OCT images through the 2D reconstruction of 3D elastic waves along the x-t plane. The 2D OCT structural maps of tongue lesion tissues at various cancerous stages and during normal development were correlated with spatiotemporal Doppler OCT images, which are displayed in Figure 7. The slope became flatter during the progression of the lesion.
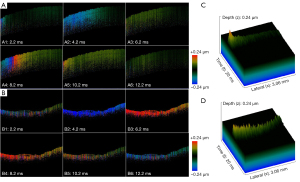
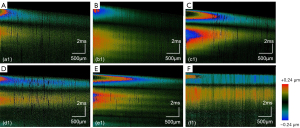
The velocity of the shear wave can be calculated from the slope of the spatiotemporal image and Eq. [3], while the corresponding Young’s modulus can be derived from Eqs. [4] and [6]. Table 2 sets out the experimental results. Figure 8A depicts a plot of the average phase velocity of the tongue tissue during lesion progression to illustrate the trend. As the lesion progressed, the velocity of the phases increased by 118.95%. The shear modulus and Young’s modulus results at different stages of the lesion are illustrated and compared in box plots shown in Figure 8B. It is evident that the shear modulus and Young’s modulus gradually increased along with the progression of the lesion. The M-HGD, CIS, and SCC samples had statistically significantly higher Young’s modulus values than the LGD, hyperplasia, and normal tissue samples (P<0.05).
Table 2
Group | Shear velocity (m/s) | Elasticity | |
---|---|---|---|
Shear modulus (kPa) | Young’s modulus (kPa) | ||
Normal | 2.85±0.18 | 8.32±1.04 | 24.94±3.11 |
Hyperplasia | 3.08±0.12 | 9.68±0.77 | 29.03±2.32 |
LGD | 3.51±0.12 | 12.62±0.81 | 37.87±2.44 |
M-HGD | 4.05±0.10 | 16.84±0.94 | 50.51±2.83 |
CIS | 4.60±0.43 | 22.43±3.87 | 67.29±11.61 |
SCC | 6.24±0.48 | 40.02±5.44 | 120.06±16.32 |
Data are presented as mean ± standard deviation. CIS, carcinoma in situ; LGD, low-grade dysplasia; M-HGD, moderate-high-grade dysplasia; SCC, squamous cell carcinoma.
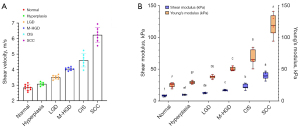
Discussion
In the present study, we first employed a shaker-OCE to identify variations in elasticity between tongue tissues of healthy rats and rats with 4NQO-induced SCC lesions at different stages. This technique improved the identification and differentiation of benign or malignant oral lesions by employing a two-pronged approach using OCE, based on elasticity and structural metrics. In addition, we showed that the 4NQO-induced OSCC model exhibited significant similarities to naturally occurring OSCC in humans, which supports the clinical value of the results presented in this study (29,41).
Previous studies have suggested that the integrity of the BM and the thickness of the epithelial tissue could serve as indicators for distinguishing between benign and malignant oral lesions (42-44). However, in the case of malignant lesions, changes in the integrity of the BM or thickness of epithelial cells can be similar, which poses challenges in determining if these variations occur in response to a local stimulus or as a part of the carcinogenesis process (4,45,46). Further, the normal thickness of the epithelium varies slightly in different areas of an individual’s oral cavity (46,47).
The stiffness of the soft tissue is important in determining if tissue can retain the normal structure and function (48,49). The elastography analysis revealed changes in the stiffness of the tissue. OCE has been recently used to study characteristics of malignant tumors, specifically breast and prostate tumors. However, this was the first study to employ OCE to measure changes in the elasticity of the tissue during the progression of OSCC disease. In this study, the Young’s modulus indicating the stiffness of the tongue SCC (TSCC) and healthy samples was 120.06±16.32 and 24.94±3.11 kPa, respectively (P<0.05). Consistent with other studies, these findings highlight that the stiffness of TSCC tissue is much higher than that of normal tissue and premalignant tumors (50,51).
Several factors, such as the quantity and state of the extracellular matrix (ECM) in the tumor microenvironment and the infiltration of inflammatory cells, influence the mechanical properties of TSCC (52). The stiffness of tumor cells is lower than that of the surrounding normal cells (9,53,54). Nevertheless, the stiffness of the ECM components is higher than that of tumor cells. The deposition of stromal components and the subsequent structural alterations ultimately result in the characteristic increase in the elastic modulus of the tongue cancer tissue (55,56).
The ECM is a vital component of the tumor microenvironment, and its remodeling is critical for tissue hardening and tumor progression (57). One key indicator of the potential of a cell to undergo invasion and become carcinogenic is ECM stiffness (58,59). As predominant structural proteins in ECM components, collagen fibers are involved in cancer fibrosis (60). In line with the findings of Shibata et al. (50), our histological analysis results revealed a positive correlation between the increase in collagen fibers and tissue stiffness. Although r fell within the weak-to-moderate correlation range, this was consistent with the complex interaction mechanisms among biological variables. The expression of collagen fibers may be regulated by multiple factors, which limits the strength of its correlation with the progression of tongue cancer (61,62). These factors include the enhanced activity of matrix-degrading enzymes leading to collagen degradation, as well as the variability and distribution characteristics of sample data (60,63).
Further, we observed more variations in the elasticity of the diseased tissue, probably because each lesion sample progressed differently and caused different degrees of alterations in the distribution and accumulation of collagen. We also noted a negative correlation between elastic fiber content and tissue stiffness. In line with the observations of Lakiotaki et al. (64), our results showed a noteworthy decline in the accumulation of elastic fibers surrounding and adjacent to the malignant tissue. One might speculate that the breakage of the elastic fibers in the LP accelerates the process of carcinogenesis by facilitating the deeper entry of cancer cells into the tissue.
In comparison to the normal and OPMD tissues, the OSCC tissues had a noticeably higher expression of α-SMA, a commonly used marker for CAFs (65). CAFs, which are characterized by a firm and retractile texture, are part of the stroma of various invasive and metastatic malignant tumors (58). The CAF-mediated production of collagen and stimulation of lysyl oxidase synthesis contribute to increased stiffness, collagen cross-connections, and ECM reorganization (50,51). In addition, CAFs are known to secrete matrix metalloproteinases (MMPs) through cell-cell interactions. The absence of any elastic fibers in the cancerous areas of the tissue may be attributed to their breakdown and destruction by MMPs (64).
The present study had several limitations. First, in addition to collagen fibers and elastic fibers, the tumor ECM components also include fibronectin, laminin, and other components (49,51), which were not studied. Second, every lesion examined in this study was a well-differentiated tumor. Bordoloi et al. (60) used polarized light microscopy to record changes in collagen fibers of different histological grades of OSCC. Consequently, we anticipated that the OCE results of highly differentiated TSCC would differ from low differentiation results. Third, as this was an in vivo investigation, the possible motion artifacts and anatomical constraints of the equipment were not considered. Fourth, the results could be affected by the mechanical compression of the shaker-OCE system’s probe against the mucosa (26).
Conclusions
We found that the biomechanical properties of the tongue tissue with various degrees of lesions can be precisely measured using the shaker-OCE technique. We identified a range of elasticity values of the tongue tissue that are correlated with different stages of tongue cancer, and could thus provide a strong basis for evaluating changes during the progression of tongue cancer. OCE is a promising diagnostic tool that could serve as a key technology for early screening of TSCC.
Acknowledgments
None.
Footnote
Reporting Checklist: The authors have completed the ARRIVE reporting checklist. Available at https://qims.amegroups.com/article/view/10.21037/qims-24-2359/rc.
Funding: This work was supported by
Conflicts of Interest: All authors have completed the ICMJE uniform disclosure form (available at https://qims.amegroups.com/article/view/10.21037/qims-24-2359/coif). The authors have no conflicts of interest to declare.
Ethical Statement: The authors are accountable for all aspects of the work in ensuring that questions related to the accuracy or integrity of any part of the work are appropriately investigated and resolved. Experiments were performed under a project license (No. NCULAE-20240827001) granted by the Animal Ethics Committee of Nanchang University and in compliance with institutional guidelines for the care and use of animals.
Open Access Statement: This is an Open Access article distributed in accordance with the Creative Commons Attribution-NonCommercial-NoDerivs 4.0 International License (CC BY-NC-ND 4.0), which permits the non-commercial replication and distribution of the article with the strict proviso that no changes or edits are made and the original work is properly cited (including links to both the formal publication through the relevant DOI and the license). See: https://creativecommons.org/licenses/by-nc-nd/4.0/.
References
- Noorlag R, Klein Nulent TJW, Delwel VEJ, Pameijer FA, Willems SM, de Bree R, van Es RJJ. Assessment of tumour depth in early tongue cancer: Accuracy of MRI and intraoral ultrasound. Oral Oncol 2020;110:104895. [Crossref] [PubMed]
- Li J, Liang F, Yu D, Qing H, Yang Y. Development of a 4-nitroquinoline-1-oxide model of lymph node metastasis in oral squamous cell carcinoma. Oral Oncol 2013;49:299-305. [Crossref] [PubMed]
- Vered M, Allon I, Buchner A, Dayan D. Stromal myofibroblasts and malignant transformation in a 4NQO rat tongue carcinogenesis model. Oral Oncol 2007;43:999-1006. [Crossref] [PubMed]
- Chen PH, Wu CH, Chen YF, Yeh YC, Lin BH, Chang KW, Lai PY, Hou MC, Lu CL, Kuo WC. Combination of structural and vascular optical coherence tomography for differentiating oral lesions of mice in different carcinogenesis stages. Biomed Opt Express 2018;9:1461-76. [Crossref] [PubMed]
- Romano A, Di Stasio D, Petruzzi M, Fiori F, Lajolo C, Santarelli A, Lucchese A, Serpico R, Contaldo M. Noninvasive Imaging Methods to Improve the Diagnosis of Oral Carcinoma and Its Precursors: State of the Art and Proposal of a Three-Step Diagnostic Process. Cancers (Basel) 2021;13:2864. [Crossref] [PubMed]
- Rahman TY, Mahanta LB, Das AK, Sarma JD. Histopathological imaging database for oral cancer analysis. Data Brief 2020;29:105114. [Crossref] [PubMed]
- Lajolo C, Gioco G, Rupe C, Patini R, Rizzo I, Romeo U, Contaldo M, Cordaro M. Patient perception after oral biopsies: an observational outpatient study. Clin Oral Investig 2021;25:5687-97. [Crossref] [PubMed]
- Li C, Zhang C, Li N, Li J. Compression Real-time Elastography for Evaluation of Salivary Gland Lesions: A Meta-analysis. J Ultrasound Med 2016;35:999-1007. [Crossref] [PubMed]
- Kwon S, Yang W, Moon D, Kim KS. Comparison of Cancer Cell Elasticity by Cell Type. J Cancer 2020;11:5403-12. [Crossref] [PubMed]
- Li GY, Cao Y. Mechanics of ultrasound elastography. Proc Math Phys Eng Sci 2017;473:20160841. [Crossref] [PubMed]
- Guo J, Savic LJ, Hillebrandt KH, Sack I. MR Elastography in Cancer. Invest Radiol 2023;58:578-86. [Crossref] [PubMed]
- Cui XW, Li KN, Yi AJ, Wang B, Wei Q, Wu GG, Dietrich CF. Ultrasound elastography. Endosc Ultrasound 2022;11:252-74. [Crossref] [PubMed]
- Parola M, Galatolo FA, La Mantia G, Cimino MGCA, Campisi G, Di Fede O. Towards explainable oral cancer recognition: Screening on imperfect images via Informed Deep Learning and Case-Based Reasoning. Comput Med Imaging Graph 2024;117:102433. [Crossref] [PubMed]
- Di Fede O, La Mantia G, Parola M, Maniscalco L, Matranga D, Tozzo P, Campisi G, Cimino MGCA. Automated Detection of Oral Malignant Lesions Using Deep Learning: Scoping Review and Meta-Analysis. Oral Dis 2025;31:1054-64. [Crossref] [PubMed]
- Kanchwala N, Kumar N, Gupta S, Lokhandwala H. Fluorescence spectroscopic study on malignant and premalignant oral mucosa of patients undergoing treatment: An observational prospective study. Int J Surg 2018;55:87-91. [Crossref] [PubMed]
- Gatesman Ammer A, Hayes KE, Martin KH, Zhang L, Spirou GA, Weed SA. Multi-photon imaging of tumor cell invasion in an orthotopic mouse model of oral squamous cell carcinoma. J Vis Exp 2011;2941. [Crossref] [PubMed]
- Jabbour JM, Bentley JL, Malik BH, Nemechek J, Warda J, Cuenca R, Cheng S, Jo JA, Maitland KC. Reflectance confocal endomicroscope with optical axial scanning for in vivo imaging of the oral mucosa. Biomed Opt Express 2014;5:3781-91. [Crossref] [PubMed]
- Putra RH, Yoda N, Astuti ER, Sasaki K. Potential imaging capability of optical coherence tomography as dental optical probe: A mini-review. Applied Sciences 2021;11:11025.
- Erdelyi RA, Duma VF, Sinescu C, Dobre GM, Bradu A, Podoleanu A. Optimization of X-ray Investigations in Dentistry Using Optical Coherence Tomography. Sensors (Basel) 2021;21:4554. [Crossref] [PubMed]
- Mandurah MM, Sadr A, Shimada Y, Kitasako Y, Nakashima S, Bakhsh TA, Tagami J, Sumi Y. Monitoring remineralization of enamel subsurface lesions by optical coherence tomography. J Biomed Opt 2013;18:046006. [Crossref] [PubMed]
- Chen PH, Lee HY, Chen YF, Yeh YC, Chang KW, Hou MC, Kuo WC. Detection of Oral Dysplastic and Early Cancerous Lesions by Polarization-Sensitive Optical Coherence Tomography. Cancers (Basel) 2020;12:2376. [Crossref] [PubMed]
- Yang Z, Shang J, Liu C, Zhang J, Liang Y. Identification of oral precancerous and cancerous tissue by swept source optical coherence tomography. Lasers Surg Med 2022;54:320-8. [Crossref] [PubMed]
- Sunny SP, Agarwal S, James BL, Heidari E, Muralidharan A, Yadav V, Pillai V, Shetty V, Chen Z, Hedne N, Wilder-Smith P, Suresh A, Kuriakose MA. Intra-operative point-of-procedure delineation of oral cancer margins using optical coherence tomography. Oral Oncol 2019;92:12-9. [Crossref] [PubMed]
- Jerjes W, Hamdoon Z, Yousif AA, Al-Rawi NH, Hopper C. Epithelial tissue thickness improves optical coherence tomography's ability in detecting oral cancer. Photodiagnosis Photodyn Ther 2019;28:69-74. [Crossref] [PubMed]
- Obade AY, Pandarathodiyil AK, Oo AL, Warnakulasuriya S, Ramanathan A. Application of optical coherence tomography to study the structural features of oral mucosa in biopsy tissues of oral dysplasia and carcinomas. Clin Oral Investig 2021;25:5411-9. [Crossref] [PubMed]
- Zhu J, Yu J, Qu Y, He Y, Li Y, Yang Q, Huo T, He X, Chen Z. Coaxial excitation longitudinal shear wave measurement for quantitative elasticity assessment using phase-resolved optical coherence elastography. Opt Lett 2018;43:2388-91. [Crossref] [PubMed]
- Sun C, Standish B, Vuong B, Wen XY, Yang V. Digital image correlation-based optical coherence elastography. J Biomed Opt 2013;18:121515. [Crossref] [PubMed]
- Ishida K, Tomita H, Nakashima T, Hirata A, Tanaka T, Shibata T, Hara A. Current mouse models of oral squamous cell carcinoma: Genetic and chemically induced models. Oral Oncol 2017;73:16-20. [Crossref] [PubMed]
- Zigmundo GCO, Schuch LF, Schmidt TR, Silveira FM, Martins MAT, Carrard VC, Martins MD, Wagner VP. 4-nitroquinoline-1-oxide (4NQO) induced oral carcinogenesis: A systematic literature review. Pathol Res Pract 2022;236:153970. [Crossref] [PubMed]
- Sagheer SH, Whitaker-Menezes D, Han JYS, Curry JM, Martinez-Outschoorn U, Philp NJ. 4NQO induced carcinogenesis: A mouse model for oral squamous cell carcinoma. Methods Cell Biol 2021;163:93-111. [Crossref] [PubMed]
- Li Y, Moon S, Chen JJ, Zhu Z, Chen Z. Ultrahigh-sensitive optical coherence elastography. Light Sci Appl 2020;9:58. [Crossref] [PubMed]
- Nguyen TM, Song S, Arnal B, Wong EY, Huang Z, Wang RK, O'Donnell M. Shear wave pulse compression for dynamic elastography using phase-sensitive optical coherence tomography. J Biomed Opt 2014;19:16013. [Crossref] [PubMed]
- Xu X, Zhu J, Chen Z. Dynamic and quantitative assessment of blood coagulation using optical coherence elastography. Sci Rep 2016;6:24294. [Crossref] [PubMed]
- Zhang Y, Luo J, Han X, Shi G, Zhang Q, He X. Quantitative Evaluation of Biomechanical Properties of the Tongue Using a Shaker-Based Optical Coherence Elastography System. Photonics 2023;10:1143.
- Wang S, Lopez AL 3rd, Morikawa Y, Tao G, Li J, Larina IV, Martin JF, Larin KV. Noncontact quantitative biomechanical characterization of cardiac muscle using shear wave imaging optical coherence tomography. Biomed Opt Express 2014;5:1980-92. [Crossref] [PubMed]
- Choi AP, Zheng YP. Estimation of Young's modulus and Poisson's ratio of soft tissue from indentation using two different-sized indentors: finite element analysis of the finite deformation effect. Med Biol Eng Comput 2005;43:258-64. [Crossref] [PubMed]
- Driemel O, Hertel K, Reichert TE, Kosmehl H. Current classification of precursor lesions of oral squamous cell carcinoma principles of the WHO classification 2005. Mund Kiefer Gesichtschir 2006;10:89-93. [Crossref] [PubMed]
- Meyerholz DK, Beck AP. Principles and approaches for reproducible scoring of tissue stains in research. Lab Invest 2018;98:844-55. [Crossref] [PubMed]
- Tuxhorn JA, Ayala GE, Smith MJ, Smith VC, Dang TD, Rowley DR. Reactive stroma in human prostate cancer: induction of myofibroblast phenotype and extracellular matrix remodeling. Clin Cancer Res 2002;8:2912-23.
- Etemad-Moghadam S, Khalili M, Tirgary F, Alaeddini M. Evaluation of myofibroblasts in oral epithelial dysplasia and squamous cell carcinoma. J Oral Pathol Med 2009;38:639-43. [Crossref] [PubMed]
- Bouaoud J, De Souza G, Darido C, Tortereau A, Elkabets M, Bertolus C, Saintigny P. The 4-NQO mouse model: An update on a well-established in vivo model of oral carcinogenesis. Methods Cell Biol 2021;163:197-229. [Crossref] [PubMed]
- Tsai MT, Lee HC, Lu CW, Wang YM, Lee CK, Yang CC, Chiang CP. Delineation of an oral cancer lesion with swept-source optical coherence tomography. J Biomed Opt 2008;13:044012. [Crossref] [PubMed]
- Tsai MT, Lee CK, Lee HC, Chen HM, Chiang CP, Wang YM, Yang CC. Differentiating oral lesions in different carcinogenesis stages with optical coherence tomography. J Biomed Opt 2009;14:044028. [Crossref] [PubMed]
- Wilder-Smith P, Lee K, Guo S, Zhang J, Osann K, Chen Z, Messadi D. In vivo diagnosis of oral dysplasia and malignancy using optical coherence tomography: preliminary studies in 50 patients. Lasers Surg Med 2009;41:353-7. [Crossref] [PubMed]
- Volgger V, Stepp H, Ihrler S, Kraft M, Leunig A, Patel PM, Susarla M, Jackson K, Betz CS. Evaluation of optical coherence tomography to discriminate lesions of the upper aerodigestive tract. Head Neck 2013;35:1558-66. [Crossref] [PubMed]
- Hamdoon Z, Jerjes W, Upile T, McKenzie G, Jay A, Hopper C. Optical coherence tomography in the assessment of suspicious oral lesions: an immediate ex vivo study. Photodiagnosis Photodyn Ther 2013;10:17-27. [Crossref] [PubMed]
- Panzarella V, Buttacavoli F, Gambino A, Capocasale G, Di Fede O, Mauceri R, Rodolico V, Campisi G. Site-Coded Oral Squamous Cell Carcinoma Evaluation by Optical Coherence Tomography (OCT): A Descriptive Pilot Study. Cancers (Basel) 2022;14:5916. [Crossref] [PubMed]
- Li C, Guan G, Ling Y, Hsu YT, Song S, Huang JT, Lang S, Wang RK, Huang Z, Nabi G. Detection and characterisation of biopsy tissue using quantitative optical coherence elastography (OCE) in men with suspected prostate cancer. Cancer Lett 2015;357:121-8. [Crossref] [PubMed]
- Gubarkova EV, Sovetsky AA, Matveev LA, Matveyev AL, Vorontsov DA, Plekhanov AA, Kuznetsov SS, Gamayunov SV, Vorontsov AY, Sirotkina MA, Gladkova ND, Zaitsev VY. Nonlinear Elasticity Assessment with Optical Coherence Elastography for High-Selectivity Differentiation of Breast Cancer Tissues. Materials (Basel) 2022;15:3308. [Crossref] [PubMed]
- Shibata M, Ishikawa A, Ishii J, Anzai E, Yagishita H, Izumo T, Sumino J, Katsurano M, Kim Y, Kanda H, Ushijima M, Yagihara K, Yoda T. Stiffness of tongue squamous cell carcinoma measured using strain elastography correlates with the amount of collagen fibers in the tumor. Oral Radiol 2022;38:278-87. [Crossref] [PubMed]
- Wang J, Jiang L. Application value of shear wave elastography in salivary gland tumors. Oral Radiol 2021;37:653-7. [Crossref] [PubMed]
- Bonollo F, Thalmann GN, Kruithof-de Julio M, Karkampouna S. The Role of Cancer-Associated Fibroblasts in Prostate Cancer Tumorigenesis. Cancers (Basel) 2020;12:1887. [Crossref] [PubMed]
- Kashani AS, Packirisamy M. Cancer cells optimize elasticity for efficient migration. R Soc Open Sci 2020;7:200747. [Crossref] [PubMed]
- Swaminathan V, Mythreye K, O'Brien ET, Berchuck A, Blobe GC, Superfine R. Mechanical stiffness grades metastatic potential in patient tumor cells and in cancer cell lines. Cancer Res 2011;71:5075-80. [Crossref] [PubMed]
- Karan B, Purbager A. Association of tumor strain ratio with prognostic factors in invasive breast cancer. Indian J Surg 2022;84:1239-44.
- Fang M, Yuan J, Peng C, Li Y. Collagen as a double-edged sword in tumor progression. Tumour Biol 2014;35:2871-82. [Crossref] [PubMed]
- Safaei S, Sajed R, Shariftabrizi A, Dorafshan S, Saeednejad Zanjani L, Dehghan Manshadi M, Madjd Z, Ghods R. Tumor matrix stiffness provides fertile soil for cancer stem cells. Cancer Cell Int 2023;23:143. [Crossref] [PubMed]
- Wright K, Ly T, Kriet M, Czirok A, Thomas SM. Cancer-Associated Fibroblasts: Master Tumor Microenvironment Modifiers. Cancers (Basel) 2023;15:1899. [Crossref] [PubMed]
- Mossinelli C, Tagliabue M, Ruju F, Cammarata G, Volpe S, Raimondi S, et al. The role of radiomics in tongue cancer: A new tool for prognosis prediction. Head Neck 2023;45:849-61. [Crossref] [PubMed]
- Bordoloi B, Siddiqui S, Jaiswal R, Tandon A, Jain A, Chaturvedi R. A quantitative and qualitative comparative analysis of collagen fibers to determine the role of connective tissue stroma in oral squamous cell carcinoma using special stains and polarized microscopy. J Oral Maxillofac Pathol 2020;24:398. [Crossref] [PubMed]
- Kardam P, Mehendiratta M, Rehani S, Kumra M, Sahay K, Jain K. Stromal fibers in oral squamous cell carcinoma: A possible new prognostic indicator? J Oral Maxillofac Pathol 2016;20:405-12. [Crossref] [PubMed]
- Fan H, Jiang W, Li H, Fang M, Xu Y, Zheng J. MMP-1/2 and TIMP-1/2 expression levels, and the levels of collagenous and elastic fibers correlate with disease progression in a hamster model of tongue cancer. Oncol Lett 2016;11:63-8. [Crossref] [PubMed]
- John RE, Murthy S. Morphological analysis of collagen and elastic fibers in oral squamous cell carcinoma using special stains and comparison with Broder's and Bryne's grading systems. Indian J Dent Res 2016;27:242-8. [Crossref] [PubMed]
- Lakiotaki E, Sakellariou S, Evangelou K, Liapis G, Patsouris E, Delladetsima I. Vascular and ductal elastotic changes in pancreatic cancer. APMIS 2016;124:181-7. [Crossref] [PubMed]
- Vered M, Grinstein-Koren O, Reiter S, Allon I, Dayan D. The effect of desalivation on the malignant transformation of the tongue epithelium and associated stromal myofibroblasts in a rat 4-nitroquinoline 1-oxide-induced carcinogenesis model. Int J Exp Pathol 2010;91:314-23. [Crossref] [PubMed]