Exploring the asynchronous changes of articular cartilage and osteochondral junction in knee osteoarthritis based on T2 mapping and ultrashort echo time imaging: a pilot study
Introduction
Knee osteoarthritis (KOA) is the most common chronic degenerative joint disease, predominantly affecting middle-aged and older adults, with a global age-standardised prevalence of about 4.3% in 2020 and an expected increase of 74.9% by 2050 (1,2). The pathological features of this disease are primarily progressive articular cartilage (AC) degeneration, accompanied by subchondral bone (SB) sclerosis, ultimately leading to joint destruction, instability, and disability (3). Consequently, understanding the pathogenesis of KOA, particularly the initiating factors, is crucial for early diagnosis, clinical prevention, and effective management of disease progression.
Magnetic resonance imaging (MRI) enables noninvasive diagnosis of KOA at an earlier stage and has become the preferred imaging diagnostic method (4). Apart from utilizing conventional MRI sequences for a qualitative evaluation of degraded AC and adjacent soft tissues, diverse quantitative techniques, such as T2 mapping, T2* mapping, and T1ρ relaxation time measurements, have been extensively employed in the investigation of AC and the osteochondral junction (OCJ) (5-7). T2 mapping technology exhibits high sensitivity to cartilage matrix lesions, enabling the detection of cartilage abnormalities and reflecting early alterations in extracellular matrix water and collagen content before the occurrence of morphological changes in the cartilage (8,9). Ultrashort echo time (UTE) imaging technology has demonstrated effectiveness in visualizing the OCJ with a short transverse relaxation time and can be integrated with T2* mapping for quantitative analysis (7,10). A normal OCJ appears as a thin, linear structure with a high signal intensity on UTE images. To investigate the origin of high-intensity signals observed in the cartilage region on UTE images, Bae et al. (10) conducted a study in which human patellar cartilage samples were categorized into distinct groups and subjected to UTE imaging; histological validation was also performed. The findings revealed that only areas containing deep layers of uncalcified cartilage or regions with calcified cartilage exhibited linear high-signal intensities on the corresponding UTE images. This indicates that UTE is an imaging modality that can be used to evaluate the OCJ. UTE studies of ex vivo samples from humans and animals have demonstrated that a bright OCJ signal line is visible in normal joints, with T2* values gradually increasing from the OCJ towards the superficial cartilage. Additionally, a significant positive correlation has been observed between T2* values and the cartilage degeneration MRI Osteoarthritis Knee Score (MOAKS) (11-13).
Numerous studies have investigated the pathogenesis and early progression of KOA, yet controversy persists regarding the precise asynchronous alterations occurring among the AC, calcified cartilage, and SB. Generally, it is believed that there is a complex interaction between the AC and SB during the occurrence and progression of early KOA (3,14). All along, most researchers have concurred that the earliest pathological changes in the early stages of KOA are degradation of the surface cartilage matrix, metabolic disorders of chondrocytes, and loss of proteoglycans. As the disease advances, these pathological changes propagate from the superficial to deeper layers, encompassing the calcified cartilage and SB regions. Consequently, the surface of the SB becomes unprotected, rendering it susceptible to direct abnormal stress, ultimately leading to the aberrant reconstruction of the SB (15,16). However, some researchers argue that alterations in the OCJ may precede those in the AC (17-19). The OCJ, as the main functional barrier between the non-calcified cartilage layer and the SB, is susceptible to damage due to aging, SB lesions, and other factors. Following damage to OCJ, a cascade of intricate biochemical molecular crosstalk mechanisms becomes activated, encompassing numerous signal transduction pathways such as TGF-β/Smad, Wnt/β-Catenin, and mitogen-activated protein kinase. The activation of these signaling pathways collectively contributes to AC degeneration, extracellular matrix degradation, and decreased chondrocyte load-bearing capacity by increasing the porosity of SB and blood vessels, disrupting chondrocyte gene expression and protein synthesis regulation, or inducing chondrocyte phenotypic alterations (18,19).
Previous studies have demonstrated that early cartilage degeneration is associated with increased T2 values and decreased T2* values. Specifically, superficial cartilage exhibits significantly higher T2 values compared to deep cartilage (20,21). Notably, T2 values vary across different regions of the knee joint, potentially influenced by factors such as age, sex, and body mass index (BMI) (22). Nevertheless, there is a paucity of studies utilizing these quantitative MRI techniques to assess the sequential changes occurring in the AC and OCJ in patients with KOA.
The primary objective of this study was to investigate the sequential order of pathophysiological changes in the AC and OCJ in early osteoarthritis, based on quantitative MRI-derived T2 values of AC and UTE-T2* values of the OCJ in the knee joint. The secondary objectives were to explore the influence of age and sex on quantitative MRI metrics in healthy knee joints, as well as the correlation between cartilage T2 values and OCJ T2* values. We present this article in accordance with the STROBE reporting checklist (available at https://qims.amegroups.com/article/view/10.21037/qims-24-1492/rc).
Methods
Subjects
The study was conducted in accordance with the Declaration of Helsinki and its subsequent amendments. The study was approved by the local Institutional Review Board of Tianjin Hospital (Medical Ethics Review No. 159 in 2023), and written informed consent was obtained from all individual participants. This was a prospective, observational study conducted at Tianjin University Tianjin Hospital from July 2023 to March 2024.
The patients with early KOA and healthy volunteers without knee pain symptoms in the past 6 months were included in the study. All participants were between 20 and 65 years with a BMI <30 kg/m2. Among them, knees with early OA were defined as having knee pain symptoms and had undergone standing radiographs of the knees in the past 3 months, showing Kellgren-Lawrence (KL) (23) grades 1 to 2. The exclusion criteria for patients with early KOA were as follows: (I) a history of knee trauma or surgery; (II) suffering from rheumatoid arthritis, hematological malignancies, bone metastases, or metabolic bone diseases; (III) Lequesne index (24) >4; and (IV) poor MRI image quality. Lequesne index serves as a calculation method for the severity of KOA, with higher scores indicating more severe disease progression and functional impairment. Specifically, a score ranging from 1 to 4 is categorized as mild. The exclusion criteria for healthy knee joints were as follows: (I) the presence of any evidence of any knee joint abnormalities on MRI; and (II) poor MRI image quality.
To ensure that the included knees were in the early stages of OA, the cartilage signal and morphology were evaluated using the Whole-Organ Magnetic Resonance Imaging Score (WORMS) (25) post-MRI. Knees with a WORMS cartilage score of >2 were excluded. The KL grading and WORMS cartilage scoring were performed by two experienced radiologists (Reader A and Reader B with 9 and 12 years of musculoskeletal imaging diagnosis experience, respectively) based on the conventional MRI images. In cases of disagreement, a consensus was reached. Figure 1 outlines the flowchart of the exclusion criteria.
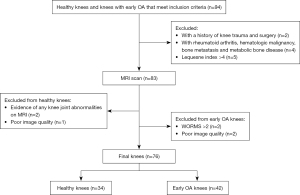
To investigate the potential influence of age and sex on the quantitative MRI values of the AC and OCJ, we stratified healthy knees into two sex groups and three distinct age cohorts: group 1: 20–35 years; group 2: 36–50 years; and group 3: 51–65 years.
Data acquisition
All MRI scans were performed using a 3.0T scanner (Ingenia CX, Philips Healthcare, Best, The Netherlands). The subjects were positioned in a supine posture with a knee flexion angle of 30° and feet first. We used an eight-channel knee joint surface flexible coil and aligned its positioning line to the inferior margin of the patella. All scanning sequences, except for three-dimensional water-selective (3D WATS) and UTE imaging, were conducted using turbo spin echo (TSE). These include T1-weighted imaging (T1WI), T2-weighted imaging with spectral attenuation with inversion recovery (T2WI SPAIR), proton density-weighted imaging with SPAIR (PDWI SPAIR), and T2 mapping. To accentuate the OCJ with a short T2 relaxation time and minimize the interference from tissues exhibiting a long transverse relaxation time during UTE imaging, we used dual-echo UTE (dUTE) imaging, where a subtraction image is obtained by subtracting the free-decay signal of the second echo (with an echo time of 2.9 ms) from the free-decay signal of the first echo (with an echo time of 0.2 ms) (Figure 2). Moreover, short hard-pulse excitation and radial sampling were employed to shorten the scan time. Table 1 details the specific scanning parameters utilized for each sequence.
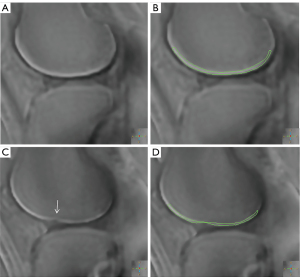
Table 1
Sequence | 3D WATS | T2 mapping | UTE-T2* mapping | T1WI | T2WI | PDWI |
---|---|---|---|---|---|---|
Orientation | Sagittal | Sagittal | Sagittal | Sagittal | Axial | Sagittal |
Fat suppression | No | No | SPAIR | No | SPAIR | SPAIR |
TR (ms) | 20 | 2,000 | 12 | 450 | 2,000 | 2,000 |
TE (ms) | 5.1 | 13; 26; 39; 52; 65; 78 | 0.2; 2.9; 5.6; 8.3 | 9 | 65 | 30 |
FOV (mm) | 150×150 | 160×160 | 200×200 | 170×170 | 153×153 | 170×170 |
Reconstruction matrix | 512×512 | 560×560 | 224×224 | 480×480 | 432×432 | 480×480 |
Voxel size (mm) | 0.3×0.3 | 0.3×0.3 | 0.9×0.9 | 0.35×0.35 | 0.34×0.34 | 0.35×0.35 |
Phase encoding steps | 304 | 311 | 132 | 214 | 212 | 202 |
Slice thickness (mm) | 3 | 3 | 1.5 | 4.5 | 4 | 4.8 |
Slice gap (mm) | 1.5 | 1.5 | 0.6 | 0.4 | 0.5 | 0.4 |
Number of slices | 50 | 50 | 100 | 14 | 14 | 14 |
Flip angle (°) | 15 | 90 | 3 | 90 | 90 | 90 |
NEX | 1 | 1 | 1 | 1 | 1 | 1 |
Acquisition time | 1 min 25 s | 3 min 38 s | 5 min 22 s | 1 min 1 s | 1 min 17 s | 1 min 12 s |
3D WATS, three-dimensional water-selective; FOV, field of view; MRI, magnetic resonance imaging; NEX, number of excitations; PD, proton density; SPAIR, spectral attenuation with inversion recovery; TE, echo time; TR, repetition time; UTE, ultrashort echo time; WI, weighted imaging.
After all the above qualitative and quantitative MRI images were obtained, they were transferred to the image and information management software (IntelliSpacePorta, version 9.0, Philips Medical Systems Nederland B.V., The Netherlands) for quantitative values measurement. Conventional sequences were used to score the cartilage according to WORMS to exclude non-early stage patients. Given that AC and the OCJ exhibit high signal intensity on 3D WATS and UTE subtraction images, respectively, in this study, 3D WATS and UTE subtraction images were utilized to determine the location, extent, and thickness of AC and the OCJ. The thickness of the high signal intensity regions was taken as the thickness of either the AC or the OCJ. Additionally, T2 mapping and UTE-T2* mapping images were employed to measure the T2 values of AC and the T2* values of the OCJ, respectively. To mitigate the impact of regional variations in knee joint cartilage on quantitative MRI metrics, we performed manual segmentation of each knee joint into six distinct regions of interest (ROIs): the medial femoral condyle (MFC), lateral femoral condyle (LFC), medial tibial plateau (MTP), lateral tibial plateau (LTP), patellar region, and trochlear region. During the manual segmentation of ROIs, the anterior and posterior boundaries of the tibiofemoral joint cartilage and the OCJ were aligned with the anterior and posterior margins of the tibial plateau, respectively. The ROI for the femoral trochlea region was defined as the remaining unmarked anterior portion of the femur. For the patellar region, the ROI included the AC and OCJ adjacent to the patella at the patellofemoral joint. Given the thin nature of the OCJ, in instances where the boundary between the OCJ and the SB was indistinct, the ROI was either appropriately reduced in scope or excluded from segmentation to ensure precision and reliability.
T2 and UTE-T2* values were directly obtained using the T2 imaging and T2* imaging software on the Philips workstation where T2 and T2* maps are independently generated from multiple echo images through single-exponential pixel-by-pixel fitting of T2 and T2*. The representative fitting curves are shown in Figure 3. This process followed image registration to ensure anatomical correspondence between images acquired from different sequences. Initially, the AC was outlined on the 3D WATS image and subsequently copied to the corresponding position on the T2 maps for the quantification of T2 values. Similarly, the OCJ was sketched on the UTE subtraction image and replicated onto UTE-T2* maps to assess the T2* values (Figure 4). During ROI delineation, joint effusion and meniscus were avoided, and the entire cartilage or OCJ in the image was included as much as possible. The ROI was manually segmented on the slice showing the thickest cartilage, as well as the preceding and subsequent two slices. The average values of the cartilage in the corresponding regions were then obtained for statistical analysis to minimize measurement errors in the data. Furthermore, the segmentation of the ROI and measurement of quantitative MRI values were performed independently by two experienced musculoskeletal radiologists (Reader A and Reader B) to analyze inter-observer consistency.
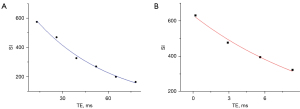
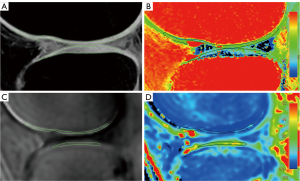
Statistical analysis
All statistical analyses were conducted using SPSS (version 22.0; IBM Corp., Armonk, NY, USA) and R v. 4.4.1 (www.r-project.org). Continuous variables were expressed as means ± standard deviation or medians (25th quartile, 75th quartile). The chi-square test was used to determine significant differences in gender and side distribution between the healthy group and the KOA group.
The intraclass correlation coefficient (ICC) was used to assess the interobserver consistency for AC T2 values and OCJ T2* values. Strength of agreement was categorized as follows: poor (ICC: <0.50), moderate (ICC: 0.50–0.74), good (ICC: 0.75–0.9), and excellent (ICC: greater than 0.9) (26).
Before performing the analysis of variance and correlation, the Shapiro-Wilk test was conducted for each dataset. Differences between the healthy group and the KOA group were analyzed through analysis of covariance controlling for age. Furthermore, to assess the diagnostic efficiency of the quantitative MRI values in identifying cartilage and OCJ lesions in different regions of the knee, receiver operating characteristic (ROC) curve analysis and DeLong test were conducted.
The T2 and T2* values of all three age groups were compared using Kruskal-Wallis and Dunn tests. The Mann-Whitney U test was used to assess whether there were statistical differences between the two sex groups. Spearman’s correlation analysis was conducted to evaluate the correlation between all measured T2 and T2* values as well as the correlation between quantitative MRI values and the Lequesne index in all patients. P values <0.05 were considered statistically significant.
Results
Ultimately, 34 normal knee joints from 27 healthy volunteers and 42 knee joints from 34 patients with early OA were included in the study. Table 2 presents the baseline characteristics of the study participants.
Table 2
Variables | Healthy group | KOA group | Statistic | P value |
---|---|---|---|---|
Number | 34 [20–35 years: 13; 36–50 years: 11; 51–65 years: 10] | 42 | – | – |
Race/ethnicity | East Asian/Han Chinese | East Asian/Han Chinese | – | – |
Age (years) | 38.2±2.2 | 44.6±1.9 | t=−2.15 | 0.03 |
Males:females | 15:19 | 23:19 | ꭓ2=0.85 | 0.35 |
Left knees:right knees | 17:17 | 20:22 | ꭓ2=0.04 | 0.83 |
The ages of the healthy group and the KOA group are presented as mean ± standard deviation. KOA, knee osteoarthritis.
Reliability assessment of T2 value and T2* value
The inter-observer agreement was excellent for both AC T2 value with an ICC of 0.94 [95% confidence interval (CI): 0.81–0.98] and OCJ T2* value with an ICC of 0.94 (95% CI: 0.72–0.96).
Comparison of T2 and T2* value between early KOA and healthy group
The AC T2 values were significantly higher in the early KOA group for MFC (P<0.001), MTP (P=0.04), patellar region (P<0.001), and trochlear region (P=0.01) relative to those in the healthy control groups. However, there was no significant difference between T2 values in LFC (P=0.59) and LTP (P=0.10) as shown in Figure 5. The T2 values for each region in the healthy group and the early KOA group are presented in Table 3.
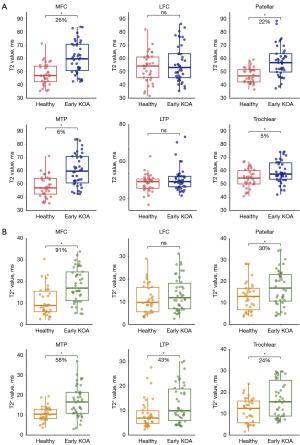
Table 3
Anatomical location | T2 (ms) | T2* (ms) |
---|---|---|
Medial femoral condyle | ||
Healthy | 47.0 (41.9–54.5) | 8.8 (5.8–15.9) |
KOA | 59.7 (50.2–70.9) | 16.9 (10.7–25.0) |
P | <0.001 | <0.001 |
Lateral femoral condyle | ||
Healthy | 54.2 (45.0–61.2) | 9.6 (5.4–16.5) |
KOA | 53.1 (45.1–64.9) | 11.7 (6.7–18.8) |
P | 0.59 | 0.16 |
Patellar | ||
Healthy | 46.8 (42.1–51.5) | 12.9 (6.3–16.8) |
KOA | 57.3 (49.7–64.4) | 16.8 (10.7–23.3) |
P | <0.001 | 0.03 |
Medial tibial plateau | ||
Healthy | 35.8 (32.6–41.5) | 10.4 (8.1–12.9) |
KOA | 38.9 (34.9–49.7) | 16.5 (10.7–21.5) |
P | 0.04 | <0.001 |
Lateral tibial plateau | ||
Healthy | 33.5 (27.9–35.7) | 6.9 (4.5–10.4) |
KOA | 33.5 (29.1–37.8) | 9.9 (5.6–19.1) |
P | 0.1 | 0.01 |
Trochlear | ||
Healthy | 54.4 (49.5–60.5) | 12.4 (5.5–15.9) |
KOA | 57.4 (53.4–66.2) | 15.5 (8.8–26.2) |
P | 0.01 | 0.01 |
Data are presented as median (interquartile range). AC, articular cartilage; KOA, knee osteoarthritis; OCJ, osteochondral junction.
For the OCJ T2* values, the early KOA group in each of the six regions measured exhibited some degree of elevation compared to the healthy group as shown in Figure 4. However, the difference of OCJ T2* value in LFC (P=0.16) was not statistically significant. The median T2* value of KOA group in MFC (P<0.001) showed the greatest increase, followed by MTP (P<0.001), LTP (P=0.01), patellar (P=0.03) and trochlear (P=0.01). The UTE-T2* values for each region in the healthy group and the early KOA group are presented in Table 3.
ROC curve analysis of T2 mapping and UTE-T2* mapping for early degeneration of cartilage
Figure 6 shows the ROC curve for diagnosing cartilage degeneration by T2 mapping and OCJ lesion by UTE-T2* mapping in early KOA. In the patellar region specifically, the area under the curve (AUC) for diagnosing early KOA using AC T2 values (AUC =0.78, P<0.001) was higher than that using OCJ UTE-T2* values (AUC =0.64, P=0.04). This difference was confirmed to be statistically significant by the Delong test (P=0.04). In contrast, no significant difference in AUC was observed between the two imaging modalities in the remaining five regions at the 0.05 significance level.
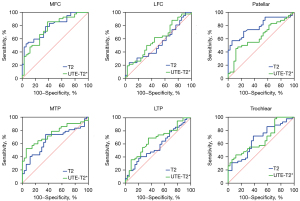
Age and sex differences in AC T2 value and OCJ T2* value
Figures 7,8 show a comparison of the quantitative MR values between different age groups in six distinct regions of healthy knees. Apart from the statistically significant difference in T2 values observed between group 1 and group 2 in the trochlear region, there were no discernible variations in T2 values among the various age groups in the remaining regions. Specifically, in the trochlear region, the T2 value of group 2 (57.1, 51.1–63.2 ms) was significantly higher than that of group 1 (50.9, 46.2–54.4 ms, P=0.02).
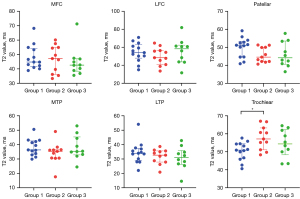

For UTE-T2* mapping, the OCJ T2* value of group 1 (11.2, 7.2–17.9 ms) was higher than those of group 2 (4.7, 3.8–6.8 ms, P=0.007) in LTP. However, no significant differences in T2* values were observed among different age groups in other regions (Figure 8).
Table 4 shows a sex-specific comparison of T2 and T2* values in healthy knees. The cartilage T2 values were significantly higher in females than in males only in MFC (52.5, 46.7–54.9 ms vs. 40.9, 37.8–46.0 ms, P=0.003) and MTP (36.3, 35.7–43.7 ms vs. 34.0, 31.0–37.3 ms, P=0.02). No significant differences were seen in the T2 values and T2* values between the sexes in any other ROIs.
Table 4
Anatomical location | T2 (ms) | T2* (ms) |
---|---|---|
Medial femoral condyle | ||
Female | 52.5 (46.7–54.9) | 8.5 (5.8–13.5) |
Male | 40.9 (37.8–46.0) | 9.1 (6.2–17.4) |
P | 0.003 | 0.41 |
Lateral femoral condyle | ||
Female | 52.3 (44.6–58.5) | 10.9 (8.6–17.3) |
Male | 56.0 (44.7–61.3) | 8.0 (4.8–10.9) |
P | 0.49 | 0.08 |
Patellar | ||
Female | 50.9 (43.9–51.9) | 12.7 (6.2–17.2) |
Male | 44.5 (41.3–48.8) | 14.6 (8.4–16.7) |
P | 0.09 | 0.83 |
Medial tibial plateau | ||
Female | 36.3 (35.7–43.7) | 10.5 (7.6–13.0) |
Male | 34.0 (31.0–37.3) | 10.3 (8.8–12.3) |
P | 0.02 | 0.68 |
Lateral tibial plateau | ||
Female | 33.5 (30.7–35.5) | 6.5 (4.6–11.1) |
Male | 30.1 (27.9–36.7) | 7.4 (4.5–8.8) |
P | 0.83 | 0.86 |
Trochlear | ||
Female | 55.1 (49.8–61.1) | 10.0 (7.3–15.3) |
Male | 54.3 (50.5–57.1) | 14.0 (5.1–16.3) |
P | 0.78 | 0.86 |
Data are presented as median (interquartile range). AC, articular cartilage; KOA, knee osteoarthritis; OCJ, osteochondral junction.
Correlation analysis
Spearman’s correlation analysis showed a weak positive correlation between AC T2 values and OCJ T2* values in the 912 regional ROIs (r=0.30, P<0.001).
Only the cartilage T2 values of the MFC (r=0.33, P=0.03) and trochlear regions (r=0.33, P=0.03) exhibited a positive correlation, albeit a weak one, with the Lequesne index. In LFC (P=0.65), patellar (P=0.25), MTP (P=0.88) and LTP (P=0.65), the Spearman’s correlation coefficient between AC T2 values and Lequesne index was 0.67, 0.18, 0.24 and 0.07, respectively.
For the correlation between OCJ T2* values and Lequesne index, no significant correlation was found irrespective of whether they were examined in MFC (r=0.02, P=0.88), LFC (r=0.19, P=0.20), patellar (r=0.02, P=0.94), MTP (r=0.07, P=0.63), LTP (r=0.25, P=0.10), or trochlear regions (r=−0.17, P=0.26).
Discussion
This study quantitatively and non-invasively evaluated early-stage AC and OCJ lesions in patients with KOA using T2 and UTE-T2* mapping techniques, respectively. In the early stage of KOA, compared with normal knees, both the T2 values of the AC and the UTE-T2* values of the OCJ increased in the MFC, MTP, patellar, and trochlear regions, while no changes were observed in the LFC. Additionally, there was an approximate 43% increase in the LTP OCJ T2* value, whereas no statistically significant difference was observed in the T2 value of the lateral tibial cartilage compared to the healthy group. This preliminary study indicates the presence of pathophysiological alterations in both AC and the OCJ during the early stages of KOA.
Early diagnosis and prevention of KOA requires careful monitoring of changes in the AC and OCJ, particularly alterations in the UTE-T2* values of the OCJ at the medial tibiofemoral joint. Although no changes in OCJ UTE-T2* values were detected in the LFC in the early stage of KOA, the OCJ plays a crucial role in transporting nutrients from the SB to the avascular cartilage, thereby maintaining homeostasis and function of the entire knee joint. Changes in cartilage composition and structure commence during the early stages of OA, highlighting the critical importance of early diagnosis and intervention in KOA.
Osteoarthritis is influenced by both biochemical signals and biomechanical factors. Any alterations in the tissues of the OCJ can compromise its barrier and transport functions, ultimately contributing to the initiation and progression of OA. In case of microstructural damage in SB, prostaglandins and growth factors released from osteoblasts can affect the AC (27). Bellido et al.’s research indicates that increased SB resorption in individuals with osteoporosis and OA may exacerbate cartilage damage (28). Variations in SB density or stiffness can predispose the overlying AC to degeneration (29). The study conducted by Davis et al. (18) using animal models of medial tibial OA to assess the early mechanical changes, suggests that stiffening of the deep zone of the AC and a decrease in SB stiffness may precede cartilage degeneration in OA progression.
T2 values can reflect subtle changes in the water content and orientation of collagen fibers within the knee cartilage, which serve as signals of cartilage degradation and correlate with the severity of osteoarthritis (9,30). Previous studies have demonstrated that the median T2 value of AC in the healthy knee is approximately 50 ms and increases with the degree of cartilage degeneration (31,32). Consistent with the findings of Soellner et al. (32) who compared the T2 relaxation time of knee AC with intraoperative results, our findings also revealed a 5% to 26% increase in the AC T2 values in specific regions during the early stages of KOA. Furthermore, the T2 mapping technique exhibited good diagnostic efficiency in distinguishing between normal and early degenerative hyaline AC.
The T2* relaxation time provides significant insight into local magnetic field inhomogeneity, potentially offering a more sensitive measure of tissue composition changes than did the T2 relaxation time (33,34). Therefore, UTE-T2* mapping of deep cartilage has great potential for early diagnosis and prediction of future KOA. Chu et al. (35) measured the T2* values of the deep cartilage in the central MFC and the anterior MFC of normal knees using three- Dimensional Double-Echo Steady-State (DESS) UTE technique, with results of 9.9±0.3 and 11.9±0.3 ms, respectively. Williams et al. (36) conducted in vivo studies and reported that the T2* value of the deep cartilage of the patella was 15.1±0.4 ms, and they also found an increase in the UTE-T2* values of the deep cartilage in the medial femoral and medial tibial in patients who underwent anterior cruciate ligament reconstruction within two years, exhibiting evidence of cartilage degeneration. Notably, this elevation was also observed in patients without medial cartilage or meniscal degeneration.
However, the UTE-T2* values are largely dependent on the choice of scanning parameters, particularly the first TE. For instance, Titchenal et al. (37) measured the UTE-T2* values of normal deep knee cartilage to be within the range of 10–20 ms using a 3D UTE sequence with 8 echoes and a first TE of 0.032 ms at a 3T magnetic field. In contrast, in a similar study by Chu et al. (35), they observed UTE-T2* values of deep knee cartilage below 10 ms when employing a UTE sequence with 11 echoes and a first TE of 0.6 ms.
The feasibility study results of Mackay et al. (7) in assessing the OCJ using UTE imaging are similar to ours, they observed that knees with pain symptoms exhibited an increased mean T2 value in the medial tibial cartilage, a reduction in the high-signal-intensity lines in the medial and lateral OCJ regions, and the peak signal intensity ratio in the medial tibia was significantly lower than that in asymptomatic volunteers, while no such difference was noted in the lateral tibia. This observation suggests that UTE-T2* mapping exhibits sensitivity to compositional alterations within the OCJ.
Although no significant alterations were noted in the T2 values of the AC in LFC and LTP, nor in the T2* values of the lateral femoral OCJ in the present study, there was a tendency towards an increase. Moreover, a positive correlation was found between the AC T2 value and the Lequesne index in MFC. This may potentially be ascribed to the comparatively infrequent manifestation of lateral compartment narrowing in tibiofemoral osteoarthritis as compared to the medial compartment (38).
The elevations of AC T2 values and OCJ T2* values in the KOA group of could be attributed either to normal physiological changes associated with aging or to biochemical alterations due to mild cartilage degeneration. However, when examining the influence of age on quantitative MRI indices, we did not find a significant increase in T2 or T2* values with advancing age. Therefore, the significant elevations in cartilage T2 values and OCJ T2* values observed in this study are likely due to the disruption of collagen fibers and an increase in mobile water protons in the early stages of cartilage and OCJ degeneration (39). Furthermore, the lack of observed differences in quantitative MRI indices among different age groups may be attributed to the inclusion of normal volunteers with cartilage pathology rather than normal cartilage, after strictly excluding risk factors for KOA, and who exhibit no symptoms or radiological signs of KOA. Despite these findings, there are still some inconsistencies in the literature regarding age-related changes in MRI indices (40).
Our study also did not reveal a significant impact of sex on OCJ T2* values in normal knees, whereas in females, the cartilage T2 values of medial MTP and MFC were significantly higher than those in males. This is consistent with the higher prevalence of KOA among women (41). This finding underscores the importance of understanding the impact of sex on cartilage T2 values. Regarding the influence of age on quantitative metrics, no clear pattern was observed in this study, which may be attributed to the relatively small sample size.
During the progression of OA, there exists a complex biochemical crosstalk between the cartilage and SB, resulting in mutual influences between the two (42). This finding is consistent with the observed significant correlation between the AC T2 values and the T2* values of the OCJ in this study.
In contrast to previous studies (9,11,32), this research employed 3D WATS rather than T2 mapping technology to acquire images for determining the specific location and extent of the AC. The 3D WATS sequence, based on water-fat separation technology, selectively excites water signals, effectively suppressing the interference of fat signals on cartilage imaging. Additionally, this sequence employs three-dimensional volumetric acquisition technology, significantly reducing partial volume effects and thereby enhancing the signal-to-noise ratio (SNR) and contrast of the images. It enables the acquisition of high-resolution, high-SNR full-thickness cartilage images in a single scan. In contrast, conventional T2 mapping sequences typically utilize two-dimensional imaging techniques and lower spatial resolution (43,44). Furthermore, a study by Zhang et al. (43) has demonstrated that MR cartilage images based on the 3D WATS sequence, combined with automated cartilage segmentation algorithms (such as the NNU-NET model), exhibit high segmentation accuracy (mean dice scores >0.90) in different knee compartments. Therefore, this study selected the 3D WATS images for ROI segmentation to ensure the accuracy and reliability of cartilage quantitative analysis.
Due to the thinness of the OCJ (45) and the prolonged scanning time required to achieve high spatial resolution imaging in quantitative sequences, UTE MRI has primarily been used for the assessment of full-thickness AC in the knee, with rare instances of independent evaluation of the OCJ. In this study, the shortest echo time, 0.2 ms, was employed when performing UTE imaging of the OCJ and a subtraction technique was used to suppress longer T2 signals. By acquiring two echo signals, this method was less susceptible to the effects of magnetic field inhomogeneity, enabling clear visualization of the OCJ. In combination with quantitative imaging, the scan duration for UTE was 5 min 22 s. Additionally, the SPAIR sequence was used to suppress fat signals, effectively eliminating chemical shift artifacts arising from the SB marrow.
However, this study has some limitations. First, as the subjects in our study comprised patients with early-stage KOA and healthy individuals, we did not conduct histopathological validation of the results. Second, due to technological constraints, T2 mapping imaging may be influenced by the magic angle effect, leading to a certain degree of elevation in the T2 values of the hyaline cartilage. Finally, as this study was a preliminary exploration, our observations were limited to the early stages of KOA, and we did not conduct longitudinal follow-ups to determine the ultimate outcomes for these patients. Consequently, in future research, we will enlarge the sample size, broaden the scope of cartilage assessment and incorporate additional quantitative MRI techniques to determine the changes in quantitative MRI biomarkers of cartilage and OCJ in KOA. Concurrently, we will also endeavor to enroll a greater number of young participants to establish MRI normal values and variances for healthy young adults, and to mitigate the impact of age-related factors. Furthermore, it is equally important and necessary to conduct longitudinal studies across all stages of KOA progression, including the early, middle, and late stages, in order to observe the changes in quantitative MRI values of AC and the OCJ.
Conclusions
Early KOA can be identified by T2 mapping imaging of AC and UTE-T2* mapping of the OCJ. The T2 and UTE-T2* values, respectively, provide quantitative and non-invasive measures of the degeneration in the AC and the OCJ during the early stages of KOA. Notably, quantitative MRI biomarkers demonstrate alterations in both the AC and the OCJ in early KOA, with a positive correlation observed between the AC T2 values and the OCJ T2* values.
Acknowledgments
The authors are grateful the supports from Tianjin Medical University, Tianjin University Tianjin Hospital and Philips Healthcare. We would also like to thank the friends who helped us with the creation and revision of the article, as well as Editage for their professional language polishing work on this paper.
Footnote
Reporting Checklist: The authors have completed the STROBE reporting checklist. Available at https://qims.amegroups.com/article/view/10.21037/qims-24-1492/rc
Funding: None.
Conflicts of Interest: All authors have completed the ICMJE uniform disclosure form (available at https://qims.amegroups.com/article/view/10.21037/qims-24-1492/coif). Z.S. is an employee of Philips healthcare company; however, the corporation had no influence on the design, conduct, or interpretation of the results of this study. The other authors have no conflicts of interest to declare.
Ethical Statement: The authors are accountable for all aspects of the work in ensuring that questions related to the accuracy or integrity of any part of the work are appropriately investigated and resolved. The study was conducted in accordance with the Declaration of Helsinki and its subsequent amendments. The study was approved by the local Institutional Review Board of Tianjin Hospital (Medical Ethics Review No. 159 in 2023), and written informed consent was taken from all individual participants.
Open Access Statement: This is an Open Access article distributed in accordance with the Creative Commons Attribution-NonCommercial-NoDerivs 4.0 International License (CC BY-NC-ND 4.0), which permits the non-commercial replication and distribution of the article with the strict proviso that no changes or edits are made and the original work is properly cited (including links to both the formal publication through the relevant DOI and the license). See: https://creativecommons.org/licenses/by-nc-nd/4.0/.
References
- Hunter DJ, Schofield D, Callander E. The individual and socioeconomic impact of osteoarthritis. Nat Rev Rheumatol 2014;10:437-41. [Crossref] [PubMed]
- Global, regional, and national burden of osteoarthritis, 1990-2020 and projections to 2050: a systematic analysis for the Global Burden of Disease Study 2021. Lancet Rheumatol 2023;5:e508-22. [Crossref] [PubMed]
- Yunus MHM, Nordin A, Kamal H. Pathophysiological Perspective of Osteoarthritis. Medicina (Kaunas) 2020.
- Oei EHG, Hirvasniemi J, van Zadelhoff TA, van der Heijden RA. Osteoarthritis year in review 2021: imaging. Osteoarthritis Cartilage 2022;30:226-36. [Crossref] [PubMed]
- Xie D, Tanaka M, Pedoia V, Li AK, Facchetti L, Neumann J, Lartey R, Souza RB, Link TM, Benjamin Ma C, Li X. Baseline cartilage T1ρ and T2 predicted patellofemoral joint cartilage lesion progression and patient-reported outcomes after ACL reconstruction. J Orthop Res 2023;41:1310-9. [Crossref] [PubMed]
- Wang H, Li Z, Li Q, Sommer S, Chen T, Sun Y, Wei H, Yan F, Lu Y. Comparing the Effect of Mechanical Loading on Deep and Superficial Cartilage Using Quantitative UTE MRI. J Magn Reson Imaging 2024;59:2048-57. [Crossref] [PubMed]
- Mackay JW, Low SB, Houston GC, Toms AP. Ultrashort TE evaluation of the osteochondral junction in vivo: a feasibility study. Br J Radiol 2016;89:20150493. [Crossref] [PubMed]
- Wu Z, Zaylor W, Sommer S, Xie D, Zhong X, Liu K, Kim J, Beveridge JE, Zhang X, Li X. Assessment of ultrashort echo time (UTE) T(2)* mapping at 3T for the whole knee: repeatability, the effects of fat suppression, and knee position. Quant Imaging Med Surg 2023;13:7893-909. [Crossref] [PubMed]
- Pan J, Pialat JB, Joseph T, Kuo D, Joseph GB, Nevitt MC, Link TM. Knee cartilage T2 characteristics and evolution in relation to morphologic abnormalities detected at 3-T MR imaging: a longitudinal study of the normal control cohort from the Osteoarthritis Initiative. Radiology 2011;261:507-15. [Crossref] [PubMed]
- Bae WC, Dwek JR, Znamirowski R, Statum SM, Hermida JC, D’Lima DD, Sah RL, Du J, Chung CB. Ultrashort echo time MR imaging of osteochondral junction of the knee at 3 T: identification of anatomic structures contributing to signal intensity. Radiology 2010;254:837-45. [Crossref] [PubMed]
- Mahar R, Batool S, Badar F, Xia Y. Quantitative measurement of T2, T1ρ and T1 relaxation times in articular cartilage and cartilage-bone interface by SE and UTE imaging at microscopic resolution. J Magn Reson 2018;297:76-85. [Crossref] [PubMed]
- Lombardi AF, Wei Z, Moazamian D, Jerban S, Jang H, Le N, Du J, Chung CB, Chang EY, Ma YJ. Quantitative Ultrashort Echo Time (UTE) imaging of Osteochondral Junction. IProc Intl Soc Mag Reson Med 2022. doi:
10.58530/2022/4940 .10.58530/2022/4940 - Shao H, Chang EY, Pauli C, Zanganeh S, Bae W, Chung CB, Tang G, Du J. UTE bi-component analysis of T2* relaxation in articular cartilage. Osteoarthritis Cartilage 2016;24:364-73. [Crossref] [PubMed]
- Intema F, Hazewinkel HA, Gouwens D, Bijlsma JW, Weinans H, Lafeber FP, Mastbergen SC. In early OA, thinning of the subchondral plate is directly related to cartilage damage: results from a canine ACLT-meniscectomy model. Osteoarthritis Cartilage 2010;18:691-8. [Crossref] [PubMed]
- Arokoski JP, Jurvelin JS, Väätäinen U, Helminen HJ. Normal and pathological adaptations of articular cartilage to joint loading. Scand J Med Sci Sports 2000;10:186-98. [Crossref] [PubMed]
- Hollander AP, Pidoux I, Reiner A, Rorabeck C, Bourne R, Poole AR. Damage to type II collagen in aging and osteoarthritis starts at the articular surface, originates around chondrocytes, and extends into the cartilage with progressive degeneration. J Clin Invest 1995;96:2859-69. [Crossref] [PubMed]
- Mahjoub M, Berenbaum F, Houard X. Why subchondral bone in osteoarthritis? The importance of the cartilage bone interface in osteoarthritis. Osteoporos Int 2012;23:S841-6. [Crossref] [PubMed]
- Davis S, Zekonyte J, Karali A, Roldo M, Blunn G. Early Degenerative Changes in a Spontaneous Osteoarthritis Model Assessed by Nanoindentation. Bioengineering (Basel) 2023.
- Radin EL, Rose RM. Role of subchondral bone in the initiation and progression of cartilage damage. Clin Orthop Relat Res 1986;34-40. [Crossref] [PubMed]
- Williams A, Qian Y, Bear D, Chu CR. Assessing degeneration of human articular cartilage with ultra-short echo time (UTE) T2* mapping. Osteoarthritis Cartilage 2010;18:539-46. [Crossref] [PubMed]
- Khandelwal R, Kharat A, Botchu R, Koganti D, Shah VP. High resolution T2* mapping in assessment of knee articular cartilage on 3T MRI. J Clin Orthop Trauma 2022;27:101823. [Crossref] [PubMed]
- Tsai PH, Wong CC, Chan WP, Lu TW. The value of MR T2* measurements in normal and osteoarthritic knee cartilage: effects of age, sex, and location. Eur Radiol 2019;29:4514-22. [Crossref] [PubMed]
- Kellgren JH, Lawrence JS. Radiological assessment of osteo-arthrosis. Ann Rheum Dis 1957;16:494-502. [Crossref] [PubMed]
- Lequesne MG. The algofunctional indices for hip and knee osteoarthritis. J Rheumatol 1997;24:779-81. [PubMed]
- Peterfy CG, Guermazi A, Zaim S, Tirman PF, Miaux Y, White D, Kothari M, Lu Y, Fye K, Zhao S, Genant HK. Whole-Organ Magnetic Resonance Imaging Score (WORMS) of the knee in osteoarthritis. Osteoarthritis Cartilage 2004;12:177-90. [Crossref] [PubMed]
- Shrout PE, Fleiss JL. Intraclass correlations: uses in assessing rater reliability. Psychol Bull 1979;86:420-8. [Crossref] [PubMed]
- Li G, Yin J, Gao J, Cheng TS, Pavlos NJ, Zhang C, Zheng MH. Subchondral bone in osteoarthritis: insight into risk factors and microstructural changes. Arthritis Res Ther 2013;15:223. [Crossref] [PubMed]
- Bellido M, Lugo L, Roman-Blas JA, Castañeda S, Caeiro JR, Dapia S, Calvo E, Largo R, Herrero-Beaumont G. Subchondral bone microstructural damage by increased remodelling aggravates experimental osteoarthritis preceded by osteoporosis. Arthritis Res Ther 2010;12:R152. [Crossref] [PubMed]
- Burr DB, Gallant MA. Bone remodelling in osteoarthritis. Nat Rev Rheumatol 2012;8:665-73. [Crossref] [PubMed]
- Emanuel KS, Kellner LJ, Peters MJM, Haartmans MJJ, Hooijmans MT, Emans PJ. The relation between the biochemical composition of knee articular cartilage and quantitative MRI: a systematic review and meta-analysis. Osteoarthritis Cartilage 2022;30:650-62. [Crossref] [PubMed]
- Bae WC, Biswas R, Chen K, Chang EY, Chung CB. UTE MRI of the Osteochondral Junction. Curr Radiol Rep 2014;2:35. [Crossref] [PubMed]
- Soellner ST, Goldmann A, Muelheims D, Welsch GH, Pachowsky ML. Intraoperative validation of quantitative T2 mapping in patients with articular cartilage lesions of the knee. Osteoarthritis Cartilage 2017;25:1841-9. [Crossref] [PubMed]
- Mamisch TC, Hughes T, Mosher TJ, Mueller C, Trattnig S, Boesch C, Welsch GH. T2 star relaxation times for assessment of articular cartilage at 3 T: a feasibility study. Skeletal Radiol 2012;41:287-92. [Crossref] [PubMed]
- Andreisek G, Weiger M. T2* mapping of articular cartilage: current status of research and first clinical applications. Invest Radiol 2014;49:57-62. [Crossref] [PubMed]
- Chu CR, Williams AA, West RV, Qian Y, Fu FH, Do BH, Bruno S. Quantitative Magnetic Resonance Imaging UTE-T2* Mapping of Cartilage and Meniscus Healing After Anatomic Anterior Cruciate Ligament Reconstruction. Am J Sports Med 2014;42:1847-56. [Crossref] [PubMed]
- Williams AA, Titchenal MR, Do BH, Guha A, Chu CR. MRI UTE-T2* shows high incidence of cartilage subsurface matrix changes 2 years after ACL reconstruction. J Orthop Res 2019;37:370-7. [Crossref] [PubMed]
- Titchenal MR, Williams AA, Chehab EF, Asay JL, Dragoo JL, Gold GE, McAdams TR, Andriacchi TP, Chu CR. Cartilage Subsurface Changes to Magnetic Resonance Imaging UTE-T2* 2 Years After Anterior Cruciate Ligament Reconstruction Correlate With Walking Mechanics Associated With Knee Osteoarthritis. Am J Sports Med 2018;46:565-72. [Crossref] [PubMed]
- Wise BL, Niu J, Yang M, Lane NE, Harvey W, Felson DT, Hietpas J, Nevitt M, Sharma L, Torner J, Lewis CE, Zhang Y. Patterns of compartment involvement in tibiofemoral osteoarthritis in men and women and in whites and African Americans. Arthritis Care Res (Hoboken) 2012;64:847-52. [Crossref] [PubMed]
- Matzat SJ, van Tiel J, Gold GE, Oei EH. Quantitative MRI techniques of cartilage composition. Quant Imaging Med Surg 2013;3:162-74. [PubMed]
- Wirth W, Maschek S, Eckstein F. Sex- and age-dependence of region- and layer-specific knee cartilage composition (spin-spin-relaxation time) in healthy reference subjects. Ann Anat 2017;210:1-8. [Crossref] [PubMed]
- Li D, Li S, Chen Q, Xie X. The Prevalence of Symptomatic Knee Osteoarthritis in Relation to Age, Sex, Area, Region, and Body Mass Index in China: A Systematic Review and Meta-Analysis. Front Med (Lausanne) 2020;7:304. [Crossref] [PubMed]
- Zhou X, Cao H, Yuan Y, Wu W. Biochemical Signals Mediate the Crosstalk between Cartilage and Bone in Osteoarthritis. Biomed Res Int 2020;2020:5720360. [Crossref] [PubMed]
- Zhang Q, Geng J, Zhang M, Kan T, Wang L, Ai S, Wei H, Zhang L, Liu C. Cartilage morphometry and magnetic susceptibility measurement for knee osteoarthritis with automatic cartilage segmentation. Quant Imaging Med Surg 2023;13:3508-21. [Crossref] [PubMed]
- Huang F, Wu W, Guo D, Luo Y. Application value of 3D-WATS magnetic resonance imaging in cartilage injury of knee joint. Journal of Army Medical University 2021;43:871-5.
- Shepherd DE, Seedhom BB. Thickness of human articular cartilage in joints of the lower limb. Ann Rheum Dis 1999;58:27-34. [Crossref] [PubMed]