Prediction of fusion cage subsidence after transforaminal lumbar interbody fusion based on MRI endplate bone quality
Introduction
Transforaminal lumbar interbody fusion (TLIF) is a significant surgical method for treating degenerative lumbar diseases. The surgical method involves the placement of a cage through the intervertebral foramen, which has the advantages of minimal nerve stimulation and a high fusion rate, and is therefore widely used. Cage subsidence is one of the common postoperative complications of TLIF surgery. This may lead to the loss of intervertebral height and failure of internal fixation, and severely affect the patient’s quality of life. Therefore, reducing the incidence of cage subsidence can effectively decrease surgery-related complications and benefit the patients.
Bone quality is a critical determinant of successful vertebral fusion, particularly in the lumbar region where the quality of the vertebrae is paramount. Bone mineral density (BMD) measured by dual-energy X-ray absorptiometry (DXA) is considered the gold standard for measuring bone strength (1). The accuracy of DXA measurements may be compromised by the presence of sclerotic bone resulting from degenerative changes in the lumbar spine. Consequently, DXA may not accurately represent the true bone quality of the lumbar spine in the context of degeneration (2-4). Vertebral bone quality (VBQ) scoring based on magnetic resonance imaging (MRI) has become an alternative method to DXA for measuring BMD. This method has been increasingly applied in the lumbar spine, surpassing DXA (5-9). However, the subsidence of the cage is mainly related to the quality of the endplate; therefore, the scoring of endplate bone quality (EBQ) based on MRI is currently regarded as a potentially effective method for predicting the settlement of fusion devices (10,11). This method assesses fat infiltration in the trabecular bone beneath the cartilage of the lumbar endplate by selectively measuring the region of interest (ROI) in lumbar spine MRI images. It offers the advantages of low radiation exposure and high repeatability.
At present, few studies have investigated the prediction of postoperative interbody fusion device subsidence following TLIF using EBQ scores. In the current study, we examined the clinical data of patients with degenerative lumbar diseases treated with TLIF at the Affiliated Hospital of Hebei University and evaluated the correlation between EBQ scores and cage subsidence. Our aim was to provide evidence that can assist clinicians in making informed surgical decisions. We present this article in accordance with the STROBE reporting checklist (available at https://qims.amegroups.com/article/view/10.21037/qims-20204-2533/rc).
Methods
Patient population
A retrospective analysis of clinical data was performed for 102 patients who underwent TLIF surgery at the Affiliated Hospital of Hebei University between October 2022 and October 2023.
Inclusion criteria
(I) Patients with single-segment disc herniation who still experience severe lower back pain, radiating leg pain, and abnormal leg sensations, among other symptoms of disc herniation, even after undergoing standard conservative treatment. (II) Comprehensive clinical and radiological data available. (III) Underwent single-level TLIF with bilateral pedicle screw fixation and the placement of a single polyetheretherketone (PEEK) fusion device. (IV) Postoperative follow-up period >1 year. (V) Complete clinical and radiological data at the last follow-up, including lumbar spine anteroposterior and lateral X-rays and lumbar computed tomography (CT) scans.
Exclusion criteria
(I) Patients with a history of prior lumbar spine surgery. (II) Individuals with congenital or idiopathic spinal deformities. (III) Occurrence of endplate damage during surgery or subsidence beneath the fusion device observed in immediate postoperative X-ray results. (IV) Osteoporosis characterized by a BMD T-score of ≤−2.5. (V) Patients who experience acute or delayed deep infections following the surgery.
Ethical statement
This study was conducted in accordance with the Declaration of Helsinki and its subsequent amendments. The study was approved by the Ethics Committee of the Affiliated Hospital of Hebei University (No. HDFY-LL-2022-090). Written informed consent was provided by all participants.
Patient data
Patient information, including gender, age, body mass index (BMI), affected segments, bone density, duration of operation, intraoperative blood loss, and duration of postoperative follow-up, were collected.
Radiological parameters are assessed using Picture Archiving and Communication Systems (PACS) software to evaluate lumbar spine X-rays at preoperative, immediate postoperative, 3-month postoperative, and final follow-up stages, in both anterior-posterior and lateral views. The lumbar sagittal plane parameters recorded included the following: lumbar lordosis (LL), defined as the angle between the upper endplates of the first lumbar vertebra and the first sacral vertebra; segmental lordosis (SL), defined as the angle between the upper and lower endplates of the fused segments; and disc height (DH), calculated as the average of the anterior and posterior heights of the intervertebral space of the affected segment. These measurements were taken from lumbar spine X-rays (Figure 1).
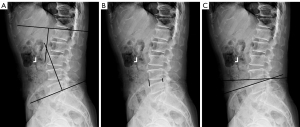
All parameters are independently measured by two spinal surgeons (D.L. and S.S.). The repeatability tests demonstrated that the parameters LL, SL, and DH exhibited high reproducibility both between and within observers, with intraclass correlation coefficient (ICC) values all exceeding 0.80. The average value was then calculated from two measurements to ensure accuracy and consistency.
Surgical procedure
To more accurately describe the surgical procedure, the example of L4/5 is provided. All patients underwent open TLIF surgery. After general anesthesia, the patient was placed in a prone position on the operating table, and a posterior median approach was used. The dissection was extended laterally from the spinous processes to the outer edge of the facet joints. Pedicle screws were inserted into the L4 and L5 vertebrae. A bone chisel was used to remove the inferior articular process of L4 and a small portion of the lamina, exposing and excising the superior articular process of L5. The same method was applied to the opposite side. The nerve roots and dural sac were protected, the L4/5 intervertebral disc was exposed, and after cutting the annulus fibrosus, a rongeur was used to remove the disc material. An endplate scraper was utilized to remove the cartilaginous endplate while preserving the bony endplate. Autologous bone chips (sourced from the excised superior and inferior articular processes and part of the lamina) were implanted into the intervertebral space. A suitably sized bullet-shaped fusion device was inserted into the intervertebral space. All patients underwent interbody fusion only, without posterolateral fusion. Appropriate length connecting rods were selected for fixation. After placing a drainage tube, the incision was closed layer by layer.
VBQ score calculation
VBQ was quantified using T1-weighted MRI by delineating the ROI within the central marrow of the vertebral bodies from L1 to L4. Additionally, the ROI was positioned on the sagittal section corresponding to the L3 level of cerebrospinal fluid (CSF), as depicted in Figure 2. The mean signal intensity (SI) for each vertebral ROI and the CSF was documented. The VBQ index was then computed using the ratio of the median SI for the vertebral bodies to the SI of the CSF, as specified by the applied formula.
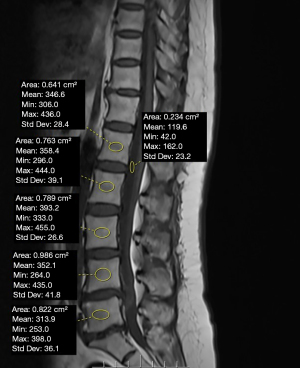
In instances where the mid-sagittal plane exhibited anomalies—such as hemangiomas, venous plexuses, or scoliosis-induced alterations, a parasagittal section was employed to more accurately assess bone quality. Vertebral bodies that were entirely compromised by abnormalities were omitted from the analysis. Should the CSF at the L3 spinal canal level be entirely occluded, the CSF ROI was alternatively positioned at either the L2 or S1 level to ensure accurate measurement (10).
EBQ score calculation
The SI of the endplate bone was calculated using the ROI. The ROI was defined as the subchondral bone area situated 3 mm from both the upper and lower endplates within the surgical segment. This was accomplished by delineating rectangles parallel to the endplates, both above and below the surgical gap, with the intervening area designated as the ROI. The average SI of the upper and lower endplates (endplate SI value) was then normalized by dividing it by the average SI of the CSF space at the L3 level. It is important to note that the ROI excluded cortical bone (12). The EBQ score of each vertebral endplate was measured as shown in Figure 3.
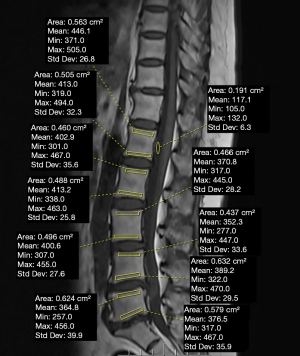
The data were independently assessed by two spinal surgeons (DL and SSS) who were not involved in the surgical procedure, and an average value was derived post-evaluation. In instances where the discrepancy between the two scores was roughly more than 10%, a third evaluator (who was also not part of the surgical team) was sought for consultation. The inter-observer ICC for VBQ and EBQ were 0.87 and 0.93, whereas the intra-observer ICC were 0.92 and 0.96, respectively, indicating good reproducibility.
Visual Analogue Scale (VAS) score and the Oswestry Disability Index (ODI) score
Pain levels in patients were evaluated preoperatively, 3 months postoperatively, and at the final follow-up using the VAS (13) and the ODI questionnaire (14). The VAS is scored out of 10, with 10 indicating the most severe pain and 1 indicating the least. The ODI is scored out of 50, with higher scores reflecting greater disability in lumbar spine function.
Cage subsidence and intervertebral fusion status
To assess the status of intervertebral fusion and potential subsidence of the cage, lumbar radiographs and CT scans were employed. The criteria for fusion as determined by CT imaging included: the visualization of bone trabeculae traversing the adjacent vertebral endplates, segmental motion of less than 5 degrees, and a relative displacement of 3 mm or less (Figure 4). Intervertebral fusion as evaluated by CT was classified into four grades: grade I: bone trabeculae penetrate the vertebral endplate, exhibiting signs of remodeling. Grade II: the intervertebral bone graft is intact, without adhesions, and shows incomplete remodeling. Grade III: the intervertebral bone graft maintains its integrity and displays radiating white spots. Grade IV: the intervertebral bone graft structure is compromised, with evidence of collapse or resorption. Fusion was indicated by grades I and II (15). Cage subsidence was characterized by the embedding of the fusion device into the adjacent upper and lower endplates by more than 2 mm (Figure 5). Severe subsidence was defined as an embedment exceeding 4 mm (16).
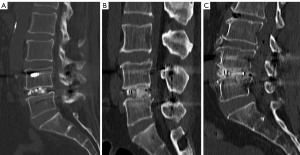

Statistical analysis
Statistical analysis was conducted on the obtained data using SPSS 26.0 software (IBM Corp., Armonk, NY, USA). Measurement data that conformed to a normal distribution were recorded using mean ± standard deviation, and differences between the two groups were compared using independent sample t-tests. A P value of less than 0.05 was considered statistically significant. All relevant variables were included in a multivariate logistic regression model to identify risk factors for subsidence of the cage. The receiver operating characteristic (ROC) curve was calculated to determine the separation criteria between the non-subsidence group and the subsidence group. The area under the curve (AUC) was calculated to evaluate the EBQ and VBQ scores, and BMD.
Results
General information
All patients successfully completed the surgery in one stage. The surgery duration was 81.38±6 minutes, and the blood loss was 139±17 mL. Among the 102 patients, 77 cases (75.5%) achieved grade I fusion, and 25 cases (24.5%) achieved grade II fusion, with no occurrences of grade III or IV fusion. There were 23 cases (22.5%) of cage subsidence and 79 cases (77.5%) without subsidence, and they were divided into two groups accordingly: the subsidence group and the non-subsidence group; postoperative symptoms of lower back and leg pain were alleviated in all patients. The average follow-up period was 19±2 months, during which no issues of screw loosening or cage dislocation were observed. There were no significant differences in the surgical segments between the two groups. There were no significant differences in general patient data between the groups. Statistical differences were found in BMD, immediate postoperative DH, VBQ, and EBQ between the two groups (Table 1).
Table 1
Variables | Subsidence (n=23) | Non-subsidence (n=79) | t | P value |
---|---|---|---|---|
Age (years) | 59.48±9.24 | 55.22±11.22 | 1.66 | 0.1 |
Gender (M/F) | 14/9 | 42/37 | 0.42 | 0.513 |
BMI (kg/m2) | 25.3±1.5 | 25.4±1.2 | 0.343 | 0.732 |
Diagnosis | 2.31* | 0.876 | ||
LDH | 12 | 39 | ||
LDH and LSS | 11 | 40 | ||
Surgical level | 2.34* | 0.858 | ||
L3–4 | 1 | 1 | ||
L4–5 | 20 | 63 | ||
L5–S1 | 2 | 15 | ||
Blood loss (mL) | 138.2±18.5 | 140.5±16.6 | 0.683 | 0.496 |
Operative time (min) | 81.2±6.8 | 81.4±6.7 | 0.149 | 0.882 |
Follow-up (months) | 19.1±1.1 | 19.5±1.3 | 1.751 | 0.083 |
Preop DH (mm) | 9.21±0.70 | 9.43±1.05 | 1.16 | 0.352 |
Immediate postop DH (mm) | 12.79±0.92 | 11.52±0.96 | 5.62 | <0.001 |
3-month postop DH (mm) | 10.18±0.79 | 10.36±0.88 | 0.87 | 0.384 |
VBQ | 3.37±0.37 | 2.64±0.37 | 8.26 | <0.001 |
EBQ | 3.40±0.49 | 2.63±0.40 | 7.77 | <0.001 |
T-score (DXA) | −1.97±0.1 | −1.62±0.12 | 12.97 | <0.001 |
Data are presented as mean ± standard deviation for continuous variables or number for categorical variables. P values were calculated using independent t-tests for continuous variables and χ2 tests for categorical variables. *, stand for χ2 tests. BMI, body mass index; DH, disc height; DXA, dual-energy X-ray absorptiometry; EBQ, endplate bone quality; F, female; M, male; LDH, lumbar disc herniation; LSS, lumbar spinal stenosis; postop, postoperative; preop, preoperative; VBQ, vertebral bone quality.
VAS score and ODI score
Before surgery, at 3 months postoperative, and at the last follow-up, there were no statistically significant differences in the VAS scores for back and leg pain or the ODI scores between the two groups of patients (P>0.05). There were no statistically significant differences in the ODI and VAS scores between patients with grade I and grade II fusion, whether before surgery, after surgery, or at the last follow-up. The ODI and VAS scores of all patients were significantly improved at 3 months postoperative and at the last follow-up compared to the preoperative scores (P<0.05) (Table 2).
Table 2
Variables | Subsidence | Non-subsidence | t | P value |
---|---|---|---|---|
ODI | ||||
Preoperative | 41.78±3.692 | 28.2±8.5 | 0.699 | 0.486 |
3 months follow-up | 20.91±3.397 | 20.84±3.53 | 0.094 | 0.926 |
Final follow-up | 21.65±3.663 | 20.63±2.82 | 1.42 | 0.159 |
VAS for LBP | ||||
Preoperative | 6.41±0.31 | 6.39±0.35 | 0.303 | 0.763 |
3 months follow-up | 2.13±0.412 | 2.24±0.44 | 1.183 | 0.244 |
Final follow-up | 2.57±0.43 | 2.73±0.39 | 1.753 | 0.083 |
VAS for leg pain | ||||
Preoperative | 6.55±0.41 | 6.42±0.37 | 1.348 | 0.181 |
3 months follow-up | 2.18±0.65 | 2.28±0.44 | 0.647 | 0.523 |
Final follow-up | 2.63±0.49 | 2.83±0.51 | 1.667 | 0.099 |
Data are presented as mean ± standard deviation. P values were derived from independent t-tests. LBP, lower back pain; ODI, Oswestry Disability Index; VAS, Visual Analogue Scale.
Radiographic parameter measurements
Before surgery, the DH in the subsidence group was 9.21±0.70 mm, and in the non-subsidence group was 9.43±1.05 mm, with no significant statistical difference (P=0.352). After surgery, the DH in the subsidence group increased to 12.79±0.92 mm, and in the non-subsidence group, it increased to 11.52±0.96 mm. At all three time points—postoperatively, at 3 months post-operation, and at the last follow-up—the DH was significantly higher than before surgery in both groups (P<0.05). According to the logistic regression analysis, there is a correlation between the immediate postoperative intervertebral DH and the subsidence of the fusion device. The Youden index for the immediate postoperative intervertebral DH was 12.05 mm, indicating that a greater postoperative intervertebral DH is associated with a higher risk of subsidence of the fusion device postoperatively. At 3 months post-operation, the DH in the subsidence group decreased to 10.18±0.79 mm, and in the non-subsidence group, it decreased to 10.36±0.88 mm, with no statistical difference between the two groups. However, at the last follow-up, the DH in the subsidence group was 9.97±0.83 mm, and in the non-subsidence group, it was 10.26±0.77 mm, showing a significant statistical difference (P<0.001).
Before surgery, the LL for the non-subsidence group was 50.52°±5.312°; for the subsidence group, it was 50.38°±4.12°. Postoperatively, the LL increased to 55.46°±4.739° in the non-subsidence group and to 54.31°±3.91° in the subsidence group. At the 3-month postoperative follow-up, the LL was 53.44°±4.66° in the non-subsidence group and 52.02°±3.97° in the subsidence group. At the final follow-up, the LL was maintained at 53.06°±4.735° in the non-subsidence group and 51.79°±3.99° in the subsidence group. In both groups, the LL at the immediate postoperative period, at the 3-month follow-up, and at the final follow-up all increased compared to the preoperative values (P<0.05), with no significant difference between the two groups (P>0.05).
Before surgery, the sagittal level SL for the non-subsidence group was 15.12°±1.72°, and it was the same for the sinking group at 15.12°±1.72°. Postoperatively, the SL increased to 17.81°±1.44° in the non-subsidence group and to 18.28°±1.47° in the subsidence group. At 3 months post-surgery, the SL was maintained at 17.74°±1.20° in the non-subsidence group and at 17.35°±1.25° in the subsidence group. At the last follow-up, the SL remained at 17.6°±1.21° in the non-subsidence group and at 17.25°±1.26° in the subsidence group. In both groups, the SL postoperatively, 3 months post-surgery, and at the last follow-up was significantly higher than before surgery (P<0.05), with no significant difference between the two groups (P>0.05) (Table 3).
Table 3
Variables | Subsidence | Non-subsidence | t | P value |
---|---|---|---|---|
Preoperative | ||||
DH (mm) | 9.21±0.70 | 9.43±1.05 | 1.16 | 0.352 |
LL (°) | 50.38±4.12 | 50.52±5.312 | 0.14 | 0.886 |
SL (°) | 15.12±1.72 | 15.12±1.72 | 1.03 | 0.302 |
Postoperative | ||||
DH (mm) | 12.79±0.92* | 11.52±0.96* | 5.62 | <0.001 |
LL (°) | 54.31±3.91* | 55.46±4.739* | 1.34 | 0.183 |
SL (°) | 17.81±1.44* | 18.28±1.47* | 1.67 | 0.105 |
3 months follow-up | ||||
DH (mm) | 10.18±0.79* | 10.36±0.88* | 0.87 | 0.384 |
LL (°) | 52.02±3.97* | 53.44±4.66* | 1.65 | 0.145 |
SL (°) | 17.35±1.25* | 17.74±1.20* | 1.61 | 0.157 |
Final follow-up | ||||
DH (mm) | 9.97±0.83* | 10.26±0.77* | 4.04 | <0.001 |
LL (°) | 51.79±3.99* | 53.06±4.735* | 1.46 | 0.100 |
SL (°) | 17.25±1.26* | 17.6±1.21* | 1.42 | 0.109 |
Data are presented as mean ± standard deviation. Comparisons between the subsidence and non-subsidence groups were analyzed using independent t-tests. Comparisons within groups (preoperative vs. postoperative/follow-up) were performed using repeated-measures ANOVA (normal distributions) or Friedman tests (non-normal distributions). *, significant differences compared to preoperative values (P<0.05). ANOVA, analysis of variance; DH, disc height; LL, lumbar lordosis; SL, segmental lordosis.
VBQ, BMD, and EBQ
Multifactorial regression analysis indicated that EBQ is a major risk factor for its integration subsidence (Table 4). The AUC for EBQ score was 0.863 [95% confidence interval (CI): 0.815–0.924], and the optimal threshold for EBQ score was 3.125 (sensitivity: 84.3%; specificity: 72.2%); the AUC for VBQ score was 0.834 (95% CI: 0.762–0.883), with the optimal threshold for VBQ score being 2.98 (sensitivity: 73.2%; specificity: 65.3%). Meanwhile, the AUC for bone density was 0.811 (95% CI: 0.721–0.889), with the optimal threshold for bone density being −1.8 (sensitivity: 70.2%; specificity: 73.5%); thus, it is considered that there is a higher risk of integration sinkage when the bone density is <−1.8 This is consistent with the results of VBQ (Figure 6).
Table 4
Variable | OR (95% CI) | P value |
---|---|---|
Age | 1.309 (0.99, 1.73) | 0.102 |
Sex | −1.37 (−3.54, −0.55) | 0.51 |
BMI | 0.78 (0.56, 1.08) | 0.14 |
Preop DH | 0.79 (0.49, 1.28) | 0.34 |
Immediate postop DH | 3.85 (2.04, 7.26) | <0.001 |
EBQ | 74.95 (11.8, 472) | <0.001 |
VBQ | 71.44 (13.34, 382) | <0.001 |
T-score (DXA) | 0.556 (0.369, 0.837) | <0.005 |
Data are derived from multivariate logistic regression analysis. ORs and 95% CIs are reported. P values represent two-sided significance adjusted for covariates. BMI, body mass index; CI, confidence interval; DH, disc height; DXA, dual-energy X-ray absorptiometry; EBQ, endplate bone quality; OR, odds ratio; postop, postoperative; preop, preoperative; VBQ, vertebral bone quality.
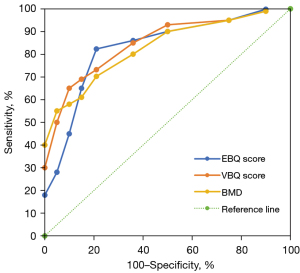
Discussion
Lumbar degenerative disease is a condition primarily characterized by pain in the lower back and legs. A research survey conducted in the United States has shown that the annual cumulative loss attributed to lower back pain exceeds $100 billion (17). For severe lumbar degenerative diseases, lumbar fusion fixation surgery can effectively alleviate symptoms. TLIF is a common surgical technique for treating lumbar degenerative diseases, first reported in 1982 and subsequently improved by Harms and others (18-20). Cage subsidence represents a frequent complication associated with TLIF surgery, potentially resulting in nerve compression symptoms, loosening of internal fixations, and other severe adverse outcomes (21-25). A report by Choi et al. indicated that following minimally invasive surgery-TLIF (MIS-TLIF) surgery, the incidence of subsidence greater than 2 mm for a banana-shaped cage was 31.8%, compared to 17.5% for a straight cage (26). Consequently, the ability to predict cage subsidence could decrease its incidence and enhance patient quality of life.
In this study, there were no statistically significant differences between the two groups of patients in terms of gender, age, duration of surgery, and blood loss. The most common segments involved were L4/5 and L5/S1. All 102 patients achieved either grade I or grade II fusion, with 77 cases (75.5%) achieving grade I fusion and 25 cases (24.5%) achieving grade II fusion. There were no cases of grade III or grade IV fusion. Among all patients, 23 cases (22.5%) experienced subsidence of the fusion device, whereas 79 cases (77.5%) did not. Despite the occurrence of fusion device subsidence, postoperative symptoms of lower back and leg pain were significantly relieved in all 102 patients. There was no significant difference between the two groups in terms of the ODI and VAS scores. Likewise, there was no difference between the groups in LL and SL.
Our research has found that the cage subsidence is closely related to the immediate postoperative DH, BMD, VBQ score, and EBQ score. In the subsidence group, the DH was significantly greater immediately postoperatively compared to the non-subsidence group, showing a statistically significant difference. The Youden index of the immediate postoperative DH was 12.05 mm. The immediate postoperative DH is indicative of the cage size; an excessively large cage can result in an increase in the immediate postoperative DH, as well as a higher cage-to-endplate ratio. Research conducted by Ohiorhenuan et al. demonstrated that a larger cage-to-lower endplate ratio increases the likelihood of cage subsidence (27). During surgical procedures, the placement of an oversized cage may require excessive expansion of the intervertebral space, potentially increasing the risk of postoperative cage subsidence. Consequently, selecting an appropriately sized cage can effectively reduce the risk.
Current research indicates that reduced BMD is a critical factor among the numerous contributors to cage subsidence (28-30). Link et al. demonstrated that patients with a BMD T-score below −1.0 exhibited a significantly increased risk of cage subsidence (3). Similarly, research conducted by Okano et al. indicated a positive correlation between osteoporosis and the degree of cage subsidence (4). Our findings reveal that BMD lower than −1.8 substantially increase the risk of cage subsidence. DXA is considered the gold standard for assessing BMD. However, DXA involves exposure to radiation and its accuracy can be compromised by factors such as bone hyperplasia and arterial calcification, potentially introducing measurement biases. Magnetic resonance-based VBQ assessment addresses these shortcomings. This technique utilizes analysis of MRI parameters to detect changes in bone structure. As vertebral bone density decreases, trabecular bone increases and fatty infiltration occurs, resulting in a high signal on T1-weighted images (31). A higher VBQ score is indicative of an increased volume of adipose tissue and a corresponding decrease in bone density. This technique has been validated as a precise tool for evaluating VBQ. Furthermore, it can supplant DXA in forecasting osteoporotic conditions (32). Previous research has indicated that VBQ can effectively predict the loosening of pedicle screws under conditions of osteoporosis (33). Additionally, a higher VBQ score has been independently identified as a risk factor for cage subsidence subsequent to TLIF surgery (34-36). Our research indicates that a VBQ score exceeding 2.98 is associated with an elevated risk of cage subsidence. However, VBQ analysis encompasses the entire vertebral body, and thus fails to evaluate the localized bone quality of the vertebra. Cage subsidence predominantly results from stress concentration around the cage. Consequently, assessing the bone quality in the vicinity of the cage provides a more accurate prediction of potential cage subsidence. EBQ is quantified using preoperative MRI by defining an ROI beneath the endplate and selectively measuring the bone quality in this area, thereby enabling a precise assessment of the bone quality at this specific site.
Our research has revealed that EBQ is a significant predictor of cage subsidence after TLIF surgery. The ROC curve analysis indicates that EBQ offers superior sensitivity and specificity in predicting cage subsidence compared to VBQ. When the EBQ score exceeds 3.125, the risk of cage subsidence significantly increases. EBQ represents the condition of the bone beneath the endplate; an elevated EBQ suggests lower BMD under the endplate, which may inadequately support the intervertebral fusion device. Moreover, osteoporosis in the area beneath the endplate can heighten stress around the cage, potentially leading to endplate collapse. In recent years, researchers have used EBQ to predict cage subsidence after oblique lateral interbody fusion (OLIF) surgery, as it accurately reflects the bone density at the vertebral endplate. Consequently, EBQ is considered both credible and reliable for assessing cage subsidence, offering substantial clinical application value (37). Our research results indicate that the endplate’s EBQ score is a convenient and feasible method for predicting cage subsidence after TLIF. Given that majority of spinal surgery patients undergo preoperative MRI scans, the EBQ can be measured for most patients without additional costs. Consequently, EBQ assessment can be routinely implemented in all spinal surgery patients. Furthermore, EBQ measurement relies on MRI technology; it does not involve exposure to radiation. This advantage renders the method particularly suitable for special populations, including children and pregnant women.
This study has several limitations. First, this was a retrospective study, with associated potential for bias, and prospective studies are needed to further verify the relationship between EBQ and cage subsidence. Second, the quantity of surgical cases examined was relatively modest, suggesting the need for a larger sample size in future investigations. Third, the follow-up period was brief, indicating the potential value of extended follow-up durations in subsequent studies.
Conclusions
The correlation between the cage subsidence and the bone quality of the endplate has been established. The EBQ score serves as an effective predictor of the incidence of cage subsidence following TLIF surgery. It has been observed that a high EBQ score presents a risk factor for cage subsidence in patients who have undergone TLIF surgery.
Acknowledgments
None.
Footnote
Reporting Checklist: The authors have completed the STROBE reporting checklist. Available at https://qims.amegroups.com/article/view/10.21037/qims-20204-2533/rc
Funding: This work was supported by
Conflicts of Interest: All authors have completed the ICMJE uniform disclosure form (available at https://qims.amegroups.com/article/view/10.21037/qims-20204-2533/coif). C.L. reports funding from the Hebei Health Committee (No. 20241689). Y.H. reports funding from the Baoding Science and Technology Bureau (No. 2341ZF335) and the Affiliated Hospital of Hebei University (No. 2023ZB01). The other authors have no conflicts of interest to declare.
Ethical Statement: The authors are accountable for all aspects of the work in ensuring that questions related to the accuracy or integrity of any part of the work are appropriately investigated and resolved. This study was conducted in accordance with the Declaration of Helsinki and its subsequent amendments. This study was conducted with approval from the Ethics Committee of Affiliated Hospital of Hebei University (No. HDFY-LL-2022-090). Written informed consent was provided by all participants.
Open Access Statement: This is an Open Access article distributed in accordance with the Creative Commons Attribution-NonCommercial-NoDerivs 4.0 International License (CC BY-NC-ND 4.0), which permits the non-commercial replication and distribution of the article with the strict proviso that no changes or edits are made and the original work is properly cited (including links to both the formal publication through the relevant DOI and the license). See: https://creativecommons.org/licenses/by-nc-nd/4.0/.
References
- Morgan SL, Prater GL. Quality in dual-energy X-ray absorptiometry scans. Bone 2017;104:13-28. [Crossref] [PubMed]
- Muraki S, Yamamoto S, Ishibashi H, Horiuchi T, Hosoi T, Orimo H, Nakamura K. Impact of degenerative spinal diseases on bone mineral density of the lumbar spine in elderly women. Osteoporos Int 2004;15:724-8. [Crossref] [PubMed]
- Link TM, Kazakia G. Update on Imaging-Based Measurement of Bone Mineral Density and Quality. Curr Rheumatol Rep 2020;22:13. [Crossref] [PubMed]
- Okano I, Jones C, Salzmann SN, Miller CO, Shirahata T, Rentenberger C, Shue J, Carrino JA, Sama AA, Cammisa FP, Girardi FP, Hughes AP. Postoperative decrease of regional volumetric bone mineral density measured by quantitative computed tomography after lumbar fusion surgery in adjacent vertebrae. Osteoporos Int 2020;31:1163-71. [Crossref] [PubMed]
- Hu YH, Yeh YC, Niu CC, Hsieh MK, Tsai TT, Chen WJ, Lai PL. Novel MRI-based vertebral bone quality score as a predictor of cage subsidence following transforaminal lumbar interbody fusion. J Neurosurg Spine 2022;37:654-62. [Crossref] [PubMed]
- Li R, Yin Y, Ji W, Wu X, Jiang H, Chen J, Zhu Q. MRI-based vertebral bone quality score effectively reflects bone quality in patients with osteoporotic vertebral compressive fractures. Eur Spine J 2022;31:1131-7. [Crossref] [PubMed]
- Haffer H, Muellner M, Chiapparelli E, Moser M, Dodo Y, Zhu J, Shue J, Sama AA, Cammisa FP, Girardi FP, Hughes AP. Bone quality in patients with osteoporosis undergoing lumbar fusion surgery: analysis of the MRI-based vertebral bone quality score and the bone microstructure derived from microcomputed tomography. Spine J 2022;22:1642-50. [Crossref] [PubMed]
- Jones C, Okano I, Salzmann SN, Reisener MJ, Chiapparelli E, Shue J, Sama AA, Cammisa FP, Girardi FP, Hughes AP. Endplate volumetric bone mineral density is a predictor for cage subsidence following lateral lumbar interbody fusion: a risk factor analysis. Spine J 2021;21:1729-37. [Crossref] [PubMed]
- Okano I, Jones C, Salzmann SN, Reisener MJ, Sax OC, Rentenberger C, Shue J, Carrino JA, Sama AA, Cammisa FP, Girardi FP, Hughes AP. Endplate volumetric bone mineral density measured by quantitative computed tomography as a novel predictive measure of severe cage subsidence after standalone lateral lumbar fusion. Eur Spine J 2020;29:1131-40. [Crossref] [PubMed]
- Ehresman J, Schilling A, Pennington Z, Gui C, Chen X, Lubelski D, Ahmed AK, Cottrill E, Khan M, Redmond KJ, Sciubba DM. A novel MRI-based score assessing trabecular bone quality to predict vertebral compression fractures in patients with spinal metastasis. J Neurosurg Spine 2020;32:499-506. [Crossref] [PubMed]
- Ai Y, Zhu C, Chen Q, Huang Y, Wang J, Ding H, Deng W, Song Y, Feng G, Liu L. Comparison of predictive value for cage subsidence between MRI-based endplate bone quality and vertebral bone quality scores following transforaminal lumbar interbody fusion: a retrospective propensity-matched study. Spine J 2024;24:1046-55. [Crossref] [PubMed]
- Jones C, Okano I, Arzani A, Dodo Y, Moser M, Reisener MJ, Chiapparelli E, Adl Amini D, Shue J, Sama AA, Cammisa FP, Girardi FP, Hughes AP. The predictive value of a novel site-specific MRI-based bone quality assessment, endplate bone quality (EBQ), for severe cage subsidence among patients undergoing standalone lateral lumbar interbody fusion. Spine J 2022;22:1875-83. [Crossref] [PubMed]
- Huskisson EC. Measurement of pain. Lancet 1974;2:1127-31. [Crossref] [PubMed]
- Fairbank JC, Pynsent PB. The Oswestry Disability Index. Spine (Phila Pa 1976) 2000;25:2940-52; discussion 2952. [Crossref] [PubMed]
- Bridwell KH, Lenke LG, McEnery KW, Baldus C, Blanke K. Anterior fresh frozen structural allografts in the thoracic and lumbar spine. Do they work if combined with posterior fusion and instrumentation in adult patients with kyphosis or anterior column defects? Spine (Phila Pa 1976) 1995;20:1410-8. [Crossref] [PubMed]
- Kim MC, Chung HT, Cho JL, Kim DJ, Chung NS. Subsidence of polyetheretherketone cage after minimally invasive transforaminal lumbar interbody fusion. J Spinal Disord Tech 2013;26:87-92. [Crossref] [PubMed]
- Katz JN. Lumbar disc disorders and low-back pain: socioeconomic factors and consequences. J Bone Joint Surg Am 2006;88:21-4. [Crossref] [PubMed]
- Hirabayashi K, Miyakawa J, Satomi K, Maruyama T, Wakano K. Operative results and postoperative progression of ossification among patients with ossification of cervical posterior longitudinal ligament. Spine (Phila Pa 1976) 1981;6:354-64. [Crossref] [PubMed]
- Ghodsi SM, Rouhani R, Abdollahzade S, Khadivi M, Faghih Jouibari M. Frequency of Vertebral Endplate Modic Changes in Patients with Unstable Lumbar Spine and Its Effect on Surgical Outcome. Asian Spine J 2015;9:737-40. [Crossref] [PubMed]
- Rao RD, Gourab K, David KS. Operative treatment of cervical spondylotic myelopathy. J Bone Joint Surg Am 2006;88:1619-40. [Crossref] [PubMed]
- Levy HA, Pinter ZW, Reed R, Harmer JR, Raftery K, Nathani KR, Katsos K, Bydon M, Fogelson JL, Elder BD, Currier BL, Newell N, Nassr AN, Freedman BA, Karamian BA, Sebastian AS. Transforaminal lumbar interbody fusion subsidence: computed tomography analysis of incidence, associated risk factors, and impact on outcomes. J Neurosurg Spine 2024;41:463-72. [Crossref] [PubMed]
- Toop N, Dhaliwal J, Gifford CS, Gibbs D, Keister A, Miracle S, Forghani R, Grossbach AJ, Farhadi HF. Promotion of higher rates of early fusion using activated titanium versus polyetheretherketone cages in adults undergoing 1- and 2-level transforaminal lumbar interbody fusion procedures: a randomized controlled trial. J Neurosurg Spine 2023;39:709-18. [Crossref] [PubMed]
- Park MK, Kim KT, Bang WS, Cho DC, Sung JK, Lee YS, Lee CK, Kim CH, Kwon BK, Lee WK, Han I. Risk factors for cage migration and cage retropulsion following transforaminal lumbar interbody fusion. Spine J 2019;19:437-47. [Crossref] [PubMed]
- Yao YC, Chou PH, Lin HH, Wang ST, Liu CL, Chang MC. Risk Factors of Cage Subsidence in Patients Received Minimally Invasive Transforaminal Lumbar Interbody Fusion. Spine (Phila Pa 1976) 2020;45:E1279-85. [Crossref] [PubMed]
- Rickert M, Fennema P, Wehner D, Rahim T, Hölper B, Eichler M, Makowski M, Meurer A, Brenneis M. Postoperative cage migration and subsidence following TLIF surgery is not associated with bony fusion. Sci Rep 2023;13:12597. [Crossref] [PubMed]
- Choi WS, Kim JS, Hur JW, Seong JH. Minimally Invasive Transforaminal Lumbar Interbody Fusion Using Banana-Shaped and Straight Cages: Radiological and Clinical Results from a Prospective Randomized Clinical Trial. Neurosurgery 2018;82:289-98. [Crossref] [PubMed]
- Ohiorhenuan IE, Walker CT, Zhou JJ, Godzik J, Sagar S, Farber SH, Uribe JS. Predictors of subsidence after lateral lumbar interbody fusion. J Neurosurg Spine 2022;37:183-7. [Crossref] [PubMed]
- Narayanan R, Tarawneh OH, Trenchfield D, Meade MH, Lee Y, Opara O, McCurdy MA, Pineda N, Kaye LD, Alhassan F, Vo M, Mangan JJ, Canseco JA, Hilibrand AS, Vaccaro AR, Kepler CK, Schroeder GD. Preoperative Hounsfield Units Predict Pedicle Screw Loosening in Osteoporotic Patients Following Short Segment Lumbar Fusion. Spine (Phila Pa 1976) 2024; Epub ahead of print. [Crossref] [PubMed]
- Li Q, Zhu C, Xia Q, Yang H, Liu L, Feng G, Song Y. The Association between High Preoperative MRI-based Vertebral Bone Quality (VBQ) Score and Titanium Mesh Cage Subsidence after Anterior Cervical Corpectomy and Fusion. Orthop Surg 2024;16:303-11. [Crossref] [PubMed]
- Subramanian T, Merrill RK, Shahi P, Pathania S, Araghi K, Maayan O, Zhao E, Shinn D, Kim YE, Kamil R, Song J, Dalal SS, Vaishnav AS, Othman Y, Steinhaus ME, Sheha ED, Dowdell JE, Iyer S, Qureshi SA. Predictors of Subsidence and its Clinical Impact After Expandable Cage Insertion in Minimally Invasive Transforaminal Interbody Fusion. Spine (Phila Pa 1976) 2023;48:1670-8. [Crossref] [PubMed]
- Kadri A, Binkley N, Hernando D, Anderson PA. Opportunistic Use of Lumbar Magnetic Resonance Imaging for Osteoporosis Screening. Osteoporos Int 2022;33:861-9. [Crossref] [PubMed]
- Huang W, Gong Z, Zheng C, Chen Y, Ma X, Wang H, Jiang J. Preoperative Assessment of Bone Density Using MRI-Based Vertebral Bone Quality Score Modified for Patients Undergoing Cervical Spine Surgery. Global Spine J 2024;14:1238-47. [Crossref] [PubMed]
- Li W, Zhu H, Hua Z, Miao D, Wang F, Tong T, Wang L. Vertebral Bone Quality Score as a Predictor of Pedicle Screw Loosening Following Surgery for Degenerative Lumbar Disease. Spine (Phila Pa 1976) 2023;48:1635-41. [Crossref] [PubMed]
- Wu H, Shan Z, Zhao F, Cheung JPY. Poor Bone Quality, Multilevel Surgery, and Narrow and Tall Cages Are Associated with Intraoperative Endplate Injuries and Late-onset Cage Subsidence in Lateral Lumbar Interbody Fusion: A Systematic Review. Clin Orthop Relat Res 2022;480:163-88. [Crossref] [PubMed]
- Ji C, Yu S, Yan N, Wang J, Hou F, Hou T, Cai W. Risk factors for subsidence of titanium mesh cage following single-level anterior cervical corpectomy and fusion. BMC Musculoskelet Disord 2020;21:32. [Crossref] [PubMed]
- Soliman MAR, Aguirre AO, Kuo CC, Ruggiero N, Azmy S, Khan A, Ghannam MM, Almeida ND, Jowdy PK, Mullin JP, Pollina J. Vertebral bone quality score independently predicts cage subsidence following transforaminal lumbar interbody fusion. Spine J 2022;22:2017-23. [Crossref] [PubMed]
- Chen Q, Ai Y, Huang Y, Li Q, Wang J, Ding H, Zhu C, Feng G, Liu L. MRI-based Endplate Bone Quality score independently predicts cage subsidence following transforaminal lumbar interbody fusion. Spine J 2023;23:1652-8. [Crossref] [PubMed]