Quantitative vessel density around foveal avascular zone as a potential imaging biomarker for detecting central nervous system injury in Wilson’s disease: an optical coherence tomography angiography study
Introduction
Wilson’s disease (WD) is a genetic disorder resulting from mutations in a gene that encodes the ATP7B protein (1), which plays a crucial role in copper transport and excretion (2). The production of defective protein will induce excessive copper accumulation, resulting in tissue and organ damage (3). The damage primarily affects the liver, and later manifests in the central nervous system (CNS), cornea, and other organs (4,5). Consequently, the typical clinical manifestations of WD include hepatic, neuropsychiatric, and ophthalmological symptoms (6).
Optical coherence tomography angiography (OCTA) adapted from optical coherence tomography (OCT), is a non-invasive imaging technique that visualizes the retinal microvascular system (7). Previous research has confirmed that changes in brain and cerebral microvascular structure may lead to alterations in retinal morphology and the microvascular system (8). Therefore, OCTA is widely used in the field of neurology. Studies have indicated that OCTA parameters of retinal microvascular system may be potential biomarkers in neurological diseases (9-12). Recently, several serum biomarkers regarding WD CNS injury have been identified (13-16). Radiological study has also provided useful prognostic and monitoring biomarkers in WD (17).
As far as we know, only one study, conducted by Seyyar et al. (18), has reported that OCTA could pre-clinically detect retinal and optic disc microcirculation changes in WD patients. However, they did not divide patients into the neurological form (NWD) and hepatic form (HWD) of the disease (18). Meanwhile, as far as we know, some of the OCTA parameters were not detected. Therefore, we speculated that changes in OCTA parameters in WD may reflect changes in cerebral microvascular structure.
We conducted the present study with the objective of evaluating OCTA parameters in WD patients. We present this article in accordance with the STROBE reporting checklist (available at https://qims.amegroups.com/article/view/10.21037/qims-24-1709/rc).
Methods
Ethics statement
This was a cross-sectional, non-interventional and observational research project. The work was conducted in accordance with the declaration of Helsinki (as revised in 2013). Written informed consent was provided by all participants or parents. The study was approved by the Ethics Committee of the First Affiliated Hospital of Guangdong Pharmaceutical University (No. WFLB0302).
Participants
We included all consecutive WD patients admitted to the First Affiliated Hospital of Guangdong Pharmaceutical University between June 2021 and April 2022, excluding those who met the exclusion criteria. Finally, a total of 42 WD patients were recruited in this study. All patients met the diagnostic criteria for WD (the diagnostic criteria developed at the 8th International Meeting on WD, Leipzig 2001; patient scores of 4 or higher establish the diagnosis) (19). All recruited patients had scores of 4 or higher in our study. Exclusion criteria: patients with an unclear diagnosis (a score of 3 or less), a contraindication to use of 1% tripicamide (tripicamide is used for pupil dilation before undergoing OCTA), a previous history of ocular trauma, inability to undergo OCTA due to severe involuntary motor symptoms (such as choreic movement, etc.), patients under the age of 15 years, and a history of diabetic retinopathy or hypertension were. In addition, 40 gender- and age-matched healthy individuals were also recruited and served as a healthy controls (HEC) group. HEC were recruited from the hospital staff through advertisement and were matched to patients based on age and gender.
All patients were divided into NWD and HWD. The classification of patients was based on the presence and severity of neuropsychiatric and hepatic manifestations (decided together by Z.H.Z. and M.F.H., neurologists with at least 20 years of treatment experience in WD). All NWD patients were assessed for neurological severity using Global Assessment Scale (GAS) tier 2 (20).
In order to explore the OCTA parameters in different genders, patients were divided into a male group and a female group. Additionally, to assess the differences in OCTA parameters based on the disease duration, all participants were further divided into three groups: patients with a disease duration of less than 5 years (<5 years group), patients with a disease duration of 5–10 years (5–10 years group), and patients with a disease duration exceeding 10 years (21).
OCTA evaluation
Each participant was evaluated by OCTA with RTVue XR Avanti System (Optovue, Inc., Fremont, CA, USA) and supported by AngioVue software (version 2018.1.1.63, Optovue). The erythrocyte movement was detected by AngioVue using a split-spectrum amplitude decorrelation angiography (SSADA) algorithm. Image acquisition and processing were also supported by the SSADA algorithm. All OCTA scans (including superficial and deep scans) were performed by the same experienced operator (blinded to the participants’ group assignments). The evaluator (blinded to the participants’ group assignments) checked all OCTA images for recognized segmentation errors, artifacts, and poor image quality, ensuring that only appropriate images were ultimately included in our study. Artifacts assessed in our study are the most prevalent types, including artifacts associated with eye motion, misidentification of retinal layers, projections, sliding vitreous, and low tomography signal. Therefore, our study excluded inferior images with poor signal strength (signal strength index <7/0), poor clarity, artifacts, doubling of vascular images, and segmentation errors.
All OCTA scans (including superficial and deep scans) both centered on the fovea (Figure 1). In our study, the superficial scan region is located 3 µm below the internal limiting membrane (ILM) to 15 µm below the internal plexiform layer (IPL); and the deep scan region is located 15–70 µm below the IPL. The scan diameters included 1, 3, and 6 mm (Figure 1). The circular areas with diameters of 1, 3, and 6 mm were labeled as foveal, parafoveal, and perifoveal zone, respectively (Figure 1). Additionally, all parafoveal and perifoveal zones were divided into four equal-sized quadrants. We collected the average of vessel density (VD) from four equal-sized quadrants in parafoveal and perifoveal zones. In the superficial scan region, the superficial foveal VD was labeled as SVD-F, superficial parafoveal VD labeled as SVD-3, and superficial perifoveal VD labeled as SVD-6. In the deep scan region, the deep foveal VD was labeled as DVD-F, deep parafoveal VD labeled as DVD-3, and deep perifoveal VD labeled as DVD-6.
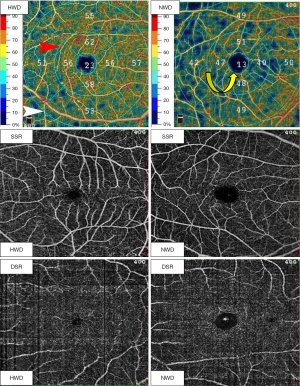
The following measured parameters were recorded: SVD-F, SVD-3 SVD-6, DVD-F, DVD-3, DVD-6, and foveal avascular zone (FAZ) parameters after scanning. The FAZ parameters included the FAZ area in mm2, FAZ perimeter (PERIM-the length of the contour demarcating the FAZ) in mm and VD of the 300 µm-width annulus surrounding the FAZ (FD-300, does not include FAZ) (Figure 2). FD-300 is a recently emerged and promising FAZ parameter in OCTA and the number of studies that have included it is still limited (22). In addition, there are significant differences in FAZ perimeter and FAZ area between normal healthy individuals (23). Therefore, FD-300 was recently introduced into OCTA research as an indicator reflecting capillary loss in the parafoveal region (24).
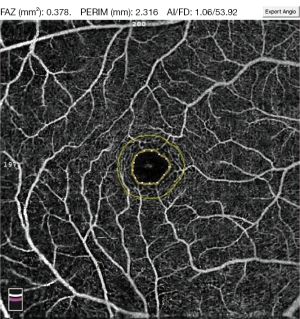
What we finally checked and collected in our study included the following: SVD-F, SVD-3 SVD-6, DVD-F, DVD-3, DVD-6, FAZ area, PERIM, and FD-300 (Figures 1,2). The VD collected in our study was quantified as the proportion of the vessel pixels out of the total number of pixels, in % percentage.
Brain magnetic resonance imaging (MRI) evaluation
In order to identify the difference of OCTA parameters in different brain visual lesions groups, all WD patients underwent 3.0T brain MRI with a GE Discovery MR750 (GE Medical Systems, Chicago, IL, USA). Imaging was performed by using T1, T2, fluid-attenuated inversion recovery (FLAIR), and susceptibility-weighted imaging sequences, and the resulting images were analyzed for changes in 5-mm axial planes. Emphasis was placed on regions commonly associated with pathological changes in WD, specifically the basal ganglia, cerebellum, midbrain, and other areas of white matter. Patients were categorized into three distinct subgroups based on the presence of visual brain MRI lesions in different regions: group 1: normal MRI (no visual brain MRI lesion), group 2: present of basal ganglia lesions, group 3: presence of basal ganglia lesions + brainstem lesions.
Data analysis
The data were examined using the software SPSS 23.0 (IBM Corp., Armonk, NY, USA), and P values below 0.05 (two-tailed) were considered statistically significant. To avoid potential internal correlations, average values of measurements from both eyes were utilized in the analysis. The data were presented as means and standard deviation (M±S). Differences in demographic categorical variables between groups were assessed using the Chi-squared test. The assumption of normality was evaluated using the Shapiro-Wilk test. Depending on the date distribution, either the independent samples t-test or the Mann-Whitney U test was employed to compare numerical variables between groups. For comparison among groups, one-way analysis of variance (ANOVA) was conducted, with Bonferroni and Tukey’s honest significant difference post hoc correction applied to normally distributed data to adjust for multiple comparisons. Pearson (r) and Spearman (ρor rho orrs) correlation coefficients were utilized to evaluate linear and non-linear correlations, respectively, between any OCTA parameters and GAS tier 2.
Results
Demographic and clinical features
In this study, we analyzed a cohort of 42 patients diagnosed with WD, consisting of 21 females and 21 males, with a mean age of 30.54±9.83 years. These patients were categorized into two distinct groups: NWD, which included 8 females and 13 males with a mean age of 30.56±10.12 years, and HWD, comprising 10 males and 11 females with a mean age of 30.53±10.00 years. Additionally, the HEC group consisted of 16 females and 24 males, with a mean age of 30.42±7.37 years. Statistical analysis revealed no significant differences in gender or age between the HEC and WD groups, nor between the NWD and HWD groups (Table 1).
Table 1
Feature | HEC | WD | NWD | HWD | P value | |
---|---|---|---|---|---|---|
NWD vs. HWD | WD vs. HEC | |||||
Number | 40 | 42 | 21 (50%) | 21 (50%) | – | – |
Gender (male/female) | 24/16 | 21/21 | 13/8 | 10/11 | 0.352 | 0.363 |
Age (years) | 30.42±7.37 | 30.54±9.83 | 30.56±10.12 | 30.53±10.00 | 0.995 | 0.950 |
Age at onset (years) | – | 19.68±8.66 | 19.96±9.12 | 19.00±11.02 | 0.773 | – |
Age at diagnosis (years) | – | 19.85±10.08 | 20.39±9.43 | 19.00±11.08 | 0.681 | – |
Disease duration (years) | – | 10.78±4.56 | 10.10±6.79 | 11.40±4.25 | 0.513 | – |
Latency from diagnosis to treatments (years) | – | 1.22±4.21 | 1.01±3.24 | 1.40±4.33 | 0.757 | – |
Treatment duration (years) | – | 10.27±6.65 | 9.14±7.05 | 11.13±3.77 | 0.323 | – |
Data are presented as n (%) or n or mean ± standard deviation. HEC, healthy controls; HWD, hepatic form of the disease; NWD, neurological form of the disease; WD, Wilson’s disease.
NWD had smaller DVD-F and FD-300 than HEC
Compared to HEC, NWD had smaller DVD-F (35.32%±7.64% vs. 38.97%±5.09%, P=0.025) and FD-300 (51.67%±5.29% vs. 55.87%±3.85%, P<0.01) (Table 2, Figure 3A). Compared to HWD, NWD had smaller FD-300 (51.67%±5.29% vs. 55.42%±4.09%, P=0.018) (Table 2, Figure 3B; representative image see Figure 1). There was no difference in OCTA parameters between HEC and HWD (Table 2). Male patients had smaller SVD-6, FAZ area, and PERIM than did female patients (Table 3, Figure 3C).
Table 2
Parameters | HEC (n=40) | NWD (n=21) | HWD (n=21) | P value |
---|---|---|---|---|
VD (%) | ||||
SVD-F | 25.33±10.63 | 20.00±8.70 | 18.08±5.66 | 0.025 |
SVD-3 | 52.52±4.20 | 50.65±3.71 | 53.18±3.00 | 0.091 |
SVD-6 | 51.29±2.69 | 50.24±2.58 | 51.29±2.24 | 0.256 |
DVD-F | 38.97±5.09 | 35.32±7.64* | 36.28±5.61 | 0.068 |
DVD-3 | 54.64±4.40 | 53.80±4.73 | 56.42±3.53 | 0.240 |
DVD-6 | 51.49±5.49 | 49.17±5.52 | 53.27±5.45 | 0.081 |
FD-300 | 55.87±3.85 | 51.67±5.29**& | 55.42±4.09 | 0.001 |
FAZ (mm2) | 0.26±0.07 | 0.28±0.11 | 0.29±0.09 | 0.571 |
PERIM (mm) | 1.95±0.26 | 2.09±0.47 | 2.11±0.39 | 0.258 |
Data are presented as mean ± standard deviation. P values below 0.05 (two-tailed) were considered statistically significant. Compared to HEC, *P<0.05, **P<0.01. Compared to HWD, &P<0.05. DVD-3, deep parafoveal vessel density; DVD-6, deep perifoveal vessel density; DVD-F, deep foveal vessel density; FAZ (mm2), foveal avascular zone area in mm2; FD-300, vessel density of the 300 μm-width annulus surrounding the foveal avascular zone (does not include FAZ); HEC, healthy controls; HWD, hepatic form of the disease; NWD, neurological form of the disease; OCTA, optical coherence tomography angiography; PERIM (mm), length of the contour demarcating the foveal avascular zone; SVD-3, superficial parafoveal vessel density; SVD-6, superficial perifoveal vessel density; SVD-F, superficial foveal vessel density; VD, vessel density.
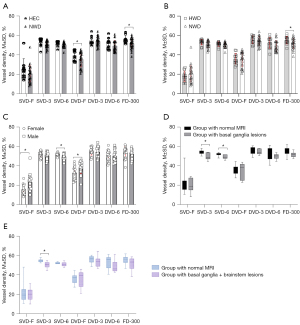
Table 3
Parameters | Male (n=21) | Female (n=21) | P value |
---|---|---|---|
VD (%) | |||
SVD-F | 22.8±17.97 | 15.40±5.39 | 0.001 |
SVD-3 | 50.38±3.90 | 51.80±3.80 | 0.239 |
SVD-6 | 49.38±2.84 | 51.11±2.68 | 0.048 |
DVD-F | 38.19±6.10 | 32.86±6.27 | 0.008 |
DVD-3 | 54.25±4.85 | 54.91±3.76 | 0.627 |
DVD-6 | 50.04±5.87 | 50.70±5.22 | 0.704 |
FD-300 | 52.13±4.26 | 53.55±5.54 | 0.358 |
FAZ (mm2) | 0.25±0.10 | 0.32±0.09 | 0.032 |
PERIM (mm) | 1.95±0.45 | 2.24±0.35 | 0.027 |
Data are presented as mean ±standard deviation. P values below 0.05 (two-tailed) were considered statistically significant. DVD-3, deep parafoveal vessel density; DVD-6, deep perifoveal vessel density; DVD-F, deep foveal vessel density; FAZ (mm2), foveal avascular zone area in mm2; FD-300, vessel density of the 300 μm-width annulus surrounding the foveal avascular zone (does not include FAZ); HEC, healthy controls; HWD, hepatic form of the disease; NWD, neurological form of the disease; OCTA, optical coherence tomography angiography; PERIM (mm), length of the contour demarcating the foveal avascular zone; SVD-3, superficial parafoveal vessel density; SVD-6, superficial perifoveal vessel density; SVD-F, superficial foveal vessel density; VD, vessel density.
The group with basal ganglia + brainstem lesions and group with basal ganglia lesions had smaller SVD-3 than the group with normal MRI
Compared to the group with normal MRI, the group with basal ganglia lesions had smaller SVD-3 (49.37%±3.34% vs. 54.45%±2.17%, P=0.002) and SVD-6 (49.20%±2.22% vs. 51.80%±1.63%, P=0.044) (Table 4, Figure 3D). Compared to the group with normal MRI, the group with basal ganglia + brainstem lesions had smaller SVD-3 (50.50%±3.3% vs. 54.45%±2.17%, P=0.002) (Table 4, Figure 3E). There was no difference in OCTA between the group with basal ganglia lesions and group with basal ganglia + brainstem lesions (Table 4).
Table 4
Parameters | Group 1 (n=12) | Group 2 (n=17) | Group 3 (n=13) | P value |
---|---|---|---|---|
VD (%) | ||||
SVD-F | 20.77±10.36 | 18.56±2.65 | 19.80±6.62 | 0.855 |
SVD-3 | 54.45±2.17 | 49.37±3.34** | 50.50±3.3# | 0.002 |
SVD-6 | 51.80±1.63 | 49.20±2.22* | 50.85±2.30 | 0.044 |
DVD-F | 35.91±4.73 | 34.43±7.61 | 35.94±8.73 | 0.881 |
DVD-3 | 55.58±3.94 | 54.87±3.38 | 53.44±5.72 | 0.555 |
DVD-6 | 52.60±6.48 | 50.10±3.04 | 48.25±5.87 | 0.213 |
FD-300 | 54.93±4.25 | 52.07±2.89 | 51.27±6.34 | 0.209 |
FAZ (mm2) | 0.28±0.09 | 0.29±0.11 | 0.29±0.13 | 0.983 |
PERIM (mm) | 2.08±0.37 | 2.12±0.42 | 2.14±0.56 | 0.963 |
Data are presented as mean ±standard deviation. Compared to group 1, #P<0.01. Compared to group 1, *P<0.05, **P<0.01. Group 1: normal MRI (no visual brain magnetic resonance imaging lesions); group 2: present of basal ganglia lesions; group 3: present of basal ganglia lesions + brainstem lesions. P values below 0.05 (two-tailed) were considered statistically significant. DVD-3, deep parafoveal vessel density; DVD-6, deep perifoveal vessel density; DVD-F, deep foveal vessel density; FAZ (mm2), foveal avascular zone area in mm2; FD-300, vessel density of the 300 μm-width annulus surrounding the foveal avascular zone (does not include FAZ); MRI, magnetic resonance imaging; OCTA, optical coherence tomography angiography; PERIM (mm), length of the contour demarcating the foveal avascular zone; SVD-3, superficial parafoveal vessel density; SVD-6, superficial perifoveal vessel density; SVD-F, superficial foveal vessel density; VD, vessel density.
Course of disease did not influence OCTA parameters
In our study, we also found that OCTA parameters were not associated with different disease course.
The correlation between GAS tier 2 scores and OCTA parameters
There was no association detected between OCTA parameters and GAS tier 2 scores.
Discussion
Our results firstly indicated that NWD had a smaller FD-300 compared to HEC and HWD, with no significant difference in OCTA parameters between HWD and HEC. Additionally, we observed variations in OCTA parameters across different gender groups and among various subgroups with abnormal brain MRI lesions.
Several studies have revealed WD had cerebral vascular injury and low cerebral blood flow perfusion (25,26). The increased expression of vascular injury factors in the WD mouse model may account for the reduced cerebral blood flow perfusion in WD patients (27). At present, owing to the constraints of human anatomy and lack of direct pathway, it is difficult to conduct non-invasive monitoring of human cerebral microvascular injury in vivo. However, due to the anatomical and physiological similarities between the retinal microvascular system and cerebral microvascular system (8), changes in brain and cerebral microvascular structure may lead to alterations in retinal morphology and microvascular system. The embryological, structural, and functional continuity between the retina and CNS makes the visual pathway a potential non-invasive research target for neurodegenerative diseases (8). Previous studies have detected retinal histological lesions by OCT in WD (21,28-30). In some respects, these results reflected the feasibility of OCT application in WD.
OCTA, adapted from OCT, is a non-invasive imaging technique that visualizes the retinal microvascular system (7). Previous studies have identified abnormalities in retinal blood flow perfusion in Alzheimer’s disease by OCTA (31). Furthermore, the observed abnormalities in retinal vasculature of Alzheimer’s patients could serve as a biomarker for the severity and progression of the disease (32). Consequently, OCTA may prove to be a valuable tool for detecting CNS injury in neurodegenerative diseases.
In our study, we found that NWD had smaller FD-300 than HEC and HWD. Furthermore, there was no significant difference in OCTA parameters between HWD and HEC. Our results suggested that NWD may be associated with retinal microvascular damage and reduced retinal blood flow perfusion. More importantly, our research findings suggested that FD-300 may be a potential index to reflect the appearance of CNS injury in WD. Therefore, we speculated that the decrease of FD-300 may be a sign of CNS injury in WD.
In the early stage, copper predominantly deposits in the liver of WD patients (4,5). We speculated that this may be why there was no significant difference in OCTA parameters between HWD and HEC in our study. As the disease progresses, copper gradually migrates to and deposits in the brain (4,5). That may be one of the reasons why NWD had a smaller FD-300 than HWD in our study. Therefore, we speculated that the FD-300 may decrease when WD patients transform from HWD into NWD or upon the onset of NWD, CNS injury will present.
Previous research had revealed that then macular layer and retinal nerve fiber layer were thinner in patients with MRI lesions (33). In our study, we found that compared to the normal MRI group, the group with basal ganglia lesions and group with basal ganglia + brainstem lesions had smaller SVD-3. Therefore, we speculated that lesions in CNS may be reflected by changes in the retinal microvascular system. In addition, OCTA may not be applicable for qualitative and quantitative assessment of CNS lesions, but can probably reflect the presence of CNS lesions.
Svetel et al. revealed that retinal nerve fiber layer thickness remained unchanged when WD patients were divided into different course of disease groups (21). Although we had not performed a follow-up study, we had reached a similar conclusion that course of disease did not affect OCTA parameters in WD. We inferred that it may also be related to the fact that most of the patients were 25–32 years old in our study.
An animal experiment revealed that WD significantly increased the expression of vascular injury factors (27). In addition, excessive copper induces cell death by targeting lipoylated tricarboxylic acid (TCA) cycle proteins (34). We speculated that excessive copper in the brain may stimulate an increase of the expression of vascular injury factors, leading to cerebral microvascular cell death by targeting acylated TCA circulating proteins. The result of the aforementioned pathophysiological changes may be cerebral micrangium occlusion and reduction. The cerebral micrangium occlusion and reduction may induce abnormalities in retinal VD because of the anatomical and physiological similarities between the retinal microvascular system and cerebral microvascular system. Therefore, the decrease of FD-300 in HWD may suggest CNS injury or the transformation of HWD to NWD. However, the application of Pearson (r) and Spearman (ρor rho orrs) correlation coefficients to assess linear and non-linear correlations, respectively, revealed no association between OCTA parameters and GAS tier 2 scores. In our study, we speculated that the decrease of FD-300 in WD patients may not be related to disease severity assessed by GAS tier 2. However, it is worth noting that the decrease of FD-300 in HWD may suggest CNS injury or the transformation of HWD to NWD.
Research has shown that OCTA is a relatively new, inexpensive, short duration, and dye-free imaging technology (35). It can pre-clinically and clinically provide a quantitative assessment of microvascular changes correlated with eye conditions (35). These advantages make it a dynamic method for observing the progression of diseases. However, as far as we know, it cannot directly perform a qualitative assessment of the brain lesions. MRI is a qualitative technique which can provide comprehensive information on brain anatomy, function, and lesions (36). Correspondingly, the technology behind its qualitative ability makes it expensive and time-consuming. Thus, we suggested that OCTA may be an option for long-term monitoring in WD.
Of course, our study had several limitations. Firstly, Litwin et al. found several new MRI sequences and techniques were used for evaluation in WD (37). We also believe that more features and MRI positivity might have been possible if more MRI sequences were used in our study. In future research, we will increase the sample size to facilitate a comparison of the differences in OCTA parameters across various new MRI sequences and techniques. Furthermore, due to the limited sample size of the current study, we cannot draw definitive conclusions regarding the significance of changes in retinal OCTA parameters in WD. Future studies will aim to expand the sample size to provide more robust findings, necessitating additional research to confirm or refute these results.
Conclusions
Our results firstly found that NWD had smaller SVD-F, DVD-F and FD-300 than HEC and NWD had smaller FD-300 than HWD. Our study suggested that OCTA may be a useful tool for detecting CNS in WD. We speculated that the decrease of FD-300 may be a sign of CNS injury in WD.
Acknowledgments
We would like to thank the participants for their support in this study.
Footnote
Reporting Checklist: The authors have completed the STROBE reporting checklist. Available at https://qims.amegroups.com/article/view/10.21037/qims-24-1709/rc
Funding: The work was supported by
Conflicts of Interest: All authors have completed the ICMJE uniform disclosure form (available at https://qims.amegroups.com/article/view/10.21037/qims-24-1709/coif). The authors have no conflicts of interest to declare.
Ethical Statement: The authors are accountable for all aspects of the work in ensuring that questions related to the accuracy or integrity of any part of the work are appropriately investigated and resolved. The study was conducted according to the statement of the Declaration of Helsinki (as revised in 2013). The use of data for publication was approved by the Ethics Committee of the First Affiliated Hospital of Guangdong Pharmaceutical University (No. WFLB0302). All patients or parents gave their informed consent to participate.
Open Access Statement: This is an Open Access article distributed in accordance with the Creative Commons Attribution-NonCommercial-NoDerivs 4.0 International License (CC BY-NC-ND 4.0), which permits the non-commercial replication and distribution of the article with the strict proviso that no changes or edits are made and the original work is properly cited (including links to both the formal publication through the relevant DOI and the license). See: https://creativecommons.org/licenses/by-nc-nd/4.0/.
References
- Członkowska A, Litwin T, Dusek P, Ferenci P, Lutsenko S, Medici V, Rybakowski JK, Weiss KH, Schilsky ML. Wilson disease. Nat Rev Dis Primers 2018;4:21. [PubMed]
- Bull PC, Thomas GR, Rommens JM, Forbes JR, Cox DW. The Wilson disease gene is a putative copper transporting P-type ATPase similar to the Menkes gene. Nat Genet 1993;5:327-37. [PubMed]
- Cai H, Cheng X, Wang XP. ATP7B gene therapy of autologous reprogrammed hepatocytes alleviates copper accumulation in a mouse model of Wilson's disease. Hepatology 2022;76:1046-57. [Crossref] [PubMed]
- Scheinberg IH, Sternlieb I. Wilson disease and idiopathic copper toxicosis. Am J Clin Nutr 1996;63:842S-5S. [PubMed]
- Litwin T, Gromadzka G, Szpak GM, Jabłonka-Salach K, Bulska E, Członkowska A. Brain metal accumulation in Wilson's disease. J Neurol Sci 2013;329:55-8. [PubMed]
- EASL Clinical Practice Guidelines. Wilson's disease. J Hepatol 2012;56:671-85. [PubMed]
- Carpineto P, Mastropasqua R, Marchini G, Toto L, Di Nicola M, Di Antonio L. Reproducibility and repeatability of foveal avascular zone measurements in healthy subjects by optical coherence tomography angiography. Br J Ophthalmol 2016;100:671-6. [PubMed]
- London A, Benhar I, Schwartz M. The retina as a window to the brain-from eye research to CNS disorders. Nat Rev Neurol 2013;9:44-53. [Crossref] [PubMed]
- Wang X, Jia Y, Spain R, Potsaid B, Liu JJ, Baumann B, Hornegger J, Fujimoto JG, Wu Q, Huang D. Optical coherence tomography angiography of optic nerve head and parafovea in multiple sclerosis. Br J Ophthalmol 2014;98:1368-73. [Crossref] [PubMed]
- Spain RI, Liu L, Zhang X, Jia Y, Tan O, Bourdette D, Huang D. Optical coherence tomography angiography enhances the detection of optic nerve damage in multiple sclerosis. Br J Ophthalmol 2018;102:520-4. [Crossref] [PubMed]
- López-Cuenca I, Salobrar-García E, Elvira-Hurtado L, Fernández-Albarral JA, Sánchez-Puebla L, Salazar JJ, Ramírez JM, Ramírez AI, de Hoz R. The Value of OCT and OCTA as Potential Biomarkers for Preclinical Alzheimer's Disease: A Review Study. Life (Basel) 2021;11:712. [PubMed]
- Giachos I, Doumazos S, Tsiogka A, Manoli K, Tagaris G, Rotsos T, Kozobolis V, Iliopoulos I, Moschos M. Retinal capillary plexus in Parkinson's disease using optical coherence tomography angiography. Int J Ophthalmol 2024;17:131-6. [PubMed]
- Lin J, Zheng Y, Liu Y, Lin Y, Wang Q, Lin XH, Zhu W, Lin WH, Wang N, Chen WJ, Fu Y. Higher Concentration of Plasma Glial Fibrillary Acidic Protein in Wilson Disease Patients with Neurological Manifestations. Mov Disord 2021;36:1446-50. [Crossref] [PubMed]
- Lekomtseva Y, Voloshyn-Gaponov I, Tatayna G. Targeting Higher Levels of Tau Protein in Ukrainian Patients with Wilson's Disease. Neurol Ther 2019;8:59-68. [Crossref] [PubMed]
- Ziemssen T, Smolinski L, Członkowska A, Akgun K, Antos A, Bembenek J, Kurkowska-Jastrzębska I, Przybyłkowski A, Skowrońska M, Redzia-Ogrodnik B, Litwin T. Serum neurofilament light chain and initial severity of neurological disease predict the early neurological deterioration in Wilson's disease. Acta Neurol Belg 2023;123:917-25. [PubMed]
- Shribman S, Poujois A, Bandmann O, Czlonkowska A, Warner TT. Wilson's disease: update on pathogenesis, biomarkers and treatments. J Neurol Neurosurg Psychiatry 2021;92:1053-61. [Crossref] [PubMed]
- Shribman S, Bocchetta M, Sudre CH, Acosta-Cabronero J, Burrows M, Cook P, Thomas DL, Gillett GT, Tsochatzis EA, Bandmann O, Rohrer JD, Warner TT. Neuroimaging correlates of brain injury in Wilson's disease: a multimodal, whole-brain MRI study. Brain 2022;145:263-75. [PubMed]
- Seyyar SA, Kanat E, Özcan ZÖ, Özçakmakcı GB, Tokuc EO, Barutçu S, Güngör K. Vascular changes in macula, optic disc, and choroid in Wilson's disease: A cross-sectional optical coherence tomography angiography study. Photodiagnosis Photodyn Ther 2023;42:103549. [Crossref] [PubMed]
- Ferenci P, Caca K, Loudianos G, Mieli-Vergani G, Tanner S, Sternlieb I, Schilsky M, Cox D, Berr F. Diagnosis and phenotypic classification of Wilson disease. Liver Int 2003;23:139-42. [Crossref] [PubMed]
- Aggarwal A, Aggarwal N, Nagral A, Jankharia G, Bhatt M. A novel Global Assessment Scale for Wilson's Disease (GAS for WD). Mov Disord 2009;24:509-18. [Crossref] [PubMed]
- Svetel M, Božić M, Vitković J, Jovanović Č, Dragašević N, Pekmezović T, Svetel M, Tomić A, Kresojević N, Kostić V. Optical coherence tomography in patients with Wilson's disease. Acta Neurol Scand 2021;144:149-54. [Crossref] [PubMed]
- Inanc M, Tekin K, Kiziltoprak H, Ozalkak S, Doguizi S, Aycan Z. Changes in Retinal Microcirculation Precede the Clinical Onset of Diabetic Retinopathy in Children With Type 1 Diabetes Mellitus. Am J Ophthalmol 2019;207:37-44. [Crossref] [PubMed]
- Linderman RE, Muthiah MN, Omoba SB, Litts K, Tarima S, Visotcky A, Kim JE, Carroll J. Variability of Foveal Avascular Zone Metrics Derived From Optical Coherence Tomography Angiography Images. Transl Vis Sci Technol 2018;7:20. [Crossref] [PubMed]
- Ragkousis A, Kozobolis V, Kabanarou S, Bontzos G, Mangouritsas G, Heliopoulos I, Chatziralli I. Vessel Density around Foveal Avascular Zone as a Potential Imaging Biomarker for Detecting Preclinical Diabetic Retinopathy: An Optical Coherence Tomography Angiography Study. Semin Ophthalmol 2020;35:316-23. [Crossref] [PubMed]
- Hawkins RA, Mazziotta JC, Phelps ME. Wilson's disease studied with FDG and positron emission tomography. Neurology 1987;37:1707-11. [Crossref] [PubMed]
- Smith FW, Gemmell HG, Sharp PF, Besson JA. Technetium-99m HMPAO imaging in patients with basal ganglia disease. Br J Radiol 1988;61:914-20. [Crossref] [PubMed]
- Chen Y, Zhang B, Cao S, Huang W, Liu N, Yang W. GanDouLing combined with Penicillamine improves cerebrovascular injury via PERK/eIF2α/CHOP endoplasmic reticulum stress pathway in the mouse model of Wilson's disease. Biosci Rep 2018;38:BSR20180800. [Crossref] [PubMed]
- Langwińska-Wośko E, Litwin T, Dzieżyc K, Karlinski M, Członkowska A. Optical coherence tomography as a marker of neurodegeneration in patients with Wilson's disease. Acta Neurol Belg 2017;117:867-71. [Crossref] [PubMed]
- Albrecht P, Müller AK, Ringelstein M, Finis D, Geerling G, Cohn E, Aktas O, Hartung HP, Hefter H, Methner A. Retinal neurodegeneration in Wilson's disease revealed by spectral domain optical coherence tomography. PLoS One 2012;7:e49825. [Crossref] [PubMed]
- Ning WQ, Lyu CX, Diao SP, Huang YQ, Liu AQ, Yu QY, Peng ZX, Hong MF, Zhou ZH. Optical coherence tomography in patients with Wilson's disease. Brain Behav 2023;13:e3014. [Crossref] [PubMed]
- Tsokolas G, Tsaousis KT, Diakonis VF, Matsou A, Tyradellis S. Optical Coherence Tomography Angiography in Neurodegenerative Diseases: A Review. Eye Brain 2020;12:73-87. [Crossref] [PubMed]
- Garcia-Martin E, Bambo MP, Marques ML, Satue M, Otin S, Larrosa JM, Polo V, Pablo LE. Ganglion cell layer measurements correlate with disease severity in patients with Alzheimer's disease. Acta Ophthalmol 2016;94:e454-9. [Crossref] [PubMed]
- Langwińska-Wośko E, Litwin T, Szulborski K, Członkowska A. Optical coherence tomography and electrophysiology of retinal and visual pathways in Wilson's disease. Metab Brain Dis 2016;31:405-15. [Crossref] [PubMed]
- Tsvetkov P, Coy S, Petrova B, Dreishpoon M, Verma A, Abdusamad M, Rossen J, Joesch-Cohen L, Humeidi R, Spangler RD, Eaton JK, Frenkel E, Kocak M, Corsello SM, Lutsenko S, Kanarek N, Santagata S, Golub TR. Copper induces cell death by targeting lipoylated TCA cycle proteins. Science 2022;375:1254-61. [Crossref] [PubMed]
- Yao X, Alam MN, Le D, Toslak D. Quantitative optical coherence tomography angiography: A review. Exp Biol Med (Maywood) 2020;245:301-12. [Crossref] [PubMed]
- Yousaf T, Dervenoulas G, Politis M. Advances in MRI Methodology. Int Rev Neurobiol 2018;141:31-76. [Crossref] [PubMed]
- Litwin T, Rędzia-Ogrodnik B, Antos A, Przybyłkowski A, Członkowska A, Bembenek JP. Brain Magnetic Resonance Imaging in Wilson's Disease-Significance and Practical Aspects-A Narrative Review. Brain Sci 2024;14:727. [Crossref] [PubMed]