Comparison of different new ultrasonic technologies in resection assessment of neurosurgery
Introduction
Brain and central nervous system (CNS) tumors consist of neoplasms originating from the brain and adjacent structures (e.g., cranial nerves, the meninx, and the medulla spinalis). Such tumors are comparatively rare worldwide, constituting only approximately 1.6% of all cancers in adults; however, their incidence has increased in some regions following improvements in diagnostic techniques and the standardization of tumor registration (1). The epidemiology of such tumors differs significantly. Notably, East Asia, and China more specifically, has the highest incidence of brain and CNS tumors. Primary malignant brain and CNS cancers often have an unfavorable prognosis, directly impair neurological functions, and cause symptoms such as headaches, vision deterioration, seizures, speech difficulties, and paralysis. Due to their location in the brain, even benign tumors can be life-threatening, resulting in high mortality and disability rates, and significantly affecting patients’ ability to function independently. Thus, the treatment of brain and CNS tumors is crucial. Currently, surgery is the main treatment method for brain and CNS tumors (2,3).
The main goals of neurosurgery are to maximize the extent of resection, minimize normal tissue damage, and most importantly, reduce postoperative recurrence rates. Due to the unique location of the CNS tumors and their structures, neurosurgery relies heavily on continuous, real-time imaging guidance.
Previous studies have shown that the extent of resection is a key prognostic factor in neurosurgery oncology (4-6). Intraoperative assistive imaging techniques include intraoperative magnetic resonance imaging (iMRI), computed tomography (CT), neuronavigation, frameless stereotaxy, fluorescence staining, and ultrasound (US). Although iMRI is the best available technique, it is not widely used due to its prolonged operative times, complex operations, and high costs (7). Intraoperative CT imaging is less accurate, costly, and not widely available. Navigation and frameless stereotaxy is based on the magnetic resonance imaging (MRI) scans acquired preoperatively and acts like a global positioning system for the surgery (8-10); however, its accuracy is compromised due to the brain shift during surgery. Conversely, intraoperative ultrasound (IOUS) has been widely used in neurosurgery due to its ease of use, real-time capability, portability, and affordability (7,8,11-22).
Currently, gray-scale US is the major type of IOUS used to guide the procedure and evaluate the extent of resection in neurosurgery. However, it is difficult for gray-scale IOUS to identify artifacts, such as acoustic shadowing from surgical, post-surgical, and post-radiation artifacts, post-resection hyperechoic rim, peritumoural-oedema hyperechogenicity, and hyperechoic blood. Thus, new ultrasonic techniques, such as contrast-enhanced ultrasound (CEUS) and shear wave elastography (SWE), are used to identify such artifacts, guide the procedure, and evaluate the extent of resection (23-34).
To date, no comparative study has been conducted on the role of several new US technologies in guiding brain tumor resection. This study sought to compare the roles of various new US technologies in guiding brain tumor resection to find a convenient and useful guiding technology for brain tumor resection. We present this article in accordance with the STARD reporting checklist (available at https://qims.amegroups.com/article/view/10.21037/qims-24-2271/rc).
Methods
This prospective study is part of a clinical trial on the application of multimodal US in the intraoperative evaluation of brain tumors. The study was conducted in accordance with the Declaration of Helsinki (as revised in 2013). The study was approved by Institutional Ethics Board of Sichuan Cancer Hospital (No. SCCHEC-02-2023-071), and informed consent was obtained from all individual participants.
From July 2022 to July 2023, 64 brain tumor patients (33 men and 31 women), with ages ranging from 28 to 78 years (mean age: 53.2±11.6 years), were included in our study. To be eligible for inclusion in the study, the patients had to meet the following inclusion criteria: (I) have been diagnosed with a brain tumor by CT and enhanced MRI at Sichuan Cancer Hospital and be eligible for brain tumor surgery; (II) be aged from 18 to 80 years; and (III) voluntarily choose to undergo an IOUS examination and sign an informed consent form. Patients were excluded from the study if they met any of the following exclusion criteria: (I) had previously received other treatments, such as radiotherapy, chemotherapy, or brain tumor surgery; (II) could not undergo brain tumor surgery due to severe coagulation dysfunction, heart failure, etc.; and/or (III) were allergic to ultrasonic contrast agents.
Before surgery, the resections were planned for all included patients by a multidisciplinary neuro-oncology team. All patients underwent microsurgical resection of the lesion. IOUS (Philips EPIQ7, the Netherlands) equipped with a 5–7- and 3–10-MHZ transducer was used during the surgery. After the craniotomy and before the dural opening, the US techniques, including B-mode, color Doppler flow imaging (CDFI), micro-flow imaging (MFI), CEUS, and SWE, were used to evaluate the features of the brain tumor and its surrounding structure. Those US techniques were then performed on the patient to confirm the microsurgical margin achieving the planned resection (pRS) at the end of the resection.
We developed a grade standard for each evaluation method based on previous literature (Table 1) (18). The US evaluation before and at the end of the resection was performed by two sonographers with at least 3 years of experience in US. Two radiologists defined the grade of resection for each US technique based on the grade standard (Table 1). If a disagreement arose, the two US physicians discussed the issue and made a unified decision. Next, 3 days after surgery, an MRI scan was performed on each patient as the reference standard. All scans were reviewed by two experienced radiologists blinded to the IOUS scan. If a disagreement arose, the two radiologists discussed the issue and made a unified decision.
Table 1
Grade | Explanation |
---|---|
pRS0 | Total resection, no tumor residue |
pRS1 | Near total resection, allowing for a residual edge of the postoperative site ≤3 mm |
pRS2 | Partial resection |
pRS3 | Tumor volume unchanged, biopsy |
MR0 | No visible tumor |
MR1 | Rim enhancement or signal abnormality (matching the tumor) at the operation site only (“Rim”), ≤3 mm in any of the dimensions and enhancement or signal abnormality was equivocal for tumor residue |
MR2 | Residual tumor measuring >3 mm in all three dimensions (greater than MR1, less than MR3) |
MR3 | No significant change to the preoperative tumor size (“minimal change”) |
bRS0 | No visible tumor |
bRS1 | Residual tumor (matching the tumor) at the operation site only (“Rim”), ≤3 mm in any of the dimensions and the image of the B-mode ultrasound was equivocal for tumor residue |
bRS2 | Residual tumor measuring >3 mm in all three dimensions (greater than bSR1, less than bSR3) |
bRS3 | No significant change to the preoperative tumor size (“minimal change”) |
cRS0 | No visible tumor |
cRS1 | Residual tumor with blood flow signal (matching the tumor) at the operation site only (“Rim”), ≤3 mm in any of the dimensions and the image of the blood flow signal was equivocal for tumor residue |
cRS2 | Residual tumor measuring >3 mm in all three dimensions (greater than cSR1, less than cSR3) |
cRS3 | No significant change to the preoperative tumor size (“minimal change”) |
mRS0 | No visible tumor |
mRS1 | Residual tumor with micro-blood flow signal (matching the tumor) at the operation site only (“Rim”), ≤3 mm in any of the dimensions and the image of the micro-blood flow signal was equivocal for tumor residue |
mRS2 | Residual tumor measuring >3 mm in all three dimensions (greater than mSR1, less than mSR3) |
mRS3 | No significant change to the preoperative tumor size (“minimal change”) |
ceRS0 | No visible tumor |
ceRS1 | Rim enhancement or signal abnormality (matching the tumor) at the operation site only (“Rim”), ≤3 mm in any of the dimensions and the enhancement or signal abnormality was equivocal for tumor residue |
ceRS2 | Residual tumor measuring >3 mm in all three dimensions (greater than ceSR1, less than ceSR3) |
ceRS3 | No significant change to the preoperative tumor size (“minimal change”) |
sRS0 | No visible tumor |
sRS1 | Rim stiffness increasing or abnormality (matching the tumor) at the operation site only (“Rim”), ≤3 mm in any of the dimensions and the stiffness increasing or abnormality was equivocal for tumor residue |
sRS2 | Residual tumor measuring >3 mm in all three dimensions (greater than MR1, less than MR3) |
sRS3 | No significant change to the preoperative tumor size (“minimal change”) |
pRS0–3: the planned resection (pRS) grade standard determined by a multidisciplinary neuro-oncology team. MR0–3: the grade standard for MRI. bRS0–3: the grade standard for B-mode. cRS0–3: the grade standard for color Doppler. mRS0–3: the grade standard for MFI. ceRS0–3: the grade standard for CEUS. sRS0–3: the grade standard for SWE. CEUS, contrast-enhanced ultrasound; MRI, magnetic resonance imaging; MFI, micro-flow imaging; SWE, shear wave elastography.
Statistical analysis
Fleiss’ kappa agreement was used to measure the agreement between the multiple US evaluations and MRI. The intra-operator agreement for the US techniques and MRI was also measured by Fleiss’ kappa agreement. The correlation between the categorical variables was analyzed using the Pearson correlation coefficient. The categorical variables are expressed as the absolute number and percentage. The statistical analyses were performed using SPSS 22.0 (SPSS Inc., USA).
Results
In total, 68 brain tumor patients were included in the study. Most of the tumors had supratentorial locations (55 supratentorial, 8 infratentorial, and 1 both supratentorial and infratentorial). The most frequent histology was glioma (n=31), followed by meningioma (n=13), metastases (n=14), schwannoma (n=2), lymphoma (n=2), and other (n=2) (Table 2).
Table 2
Variable | Value |
---|---|
Gender (male:female) | 33:31 |
Age (years) | |
Range | 26–78 |
Mean ± SD | 53.2±11.6 |
Tumor size (cm), n | |
≤5 | 39 |
>5 | 25 |
Pathology, n | |
Glioma | 31 |
Meningioma | 13 |
Metastases | 14 |
Schwannoma | 2 |
Lymphoma | 2 |
Other | 2 |
Location, n | |
Supratentorial | 55 |
Subtentorial | 8 |
Both | 1 |
SD, standard deviation.
Complete resection was the most commonly planned procedure (pRS0 in 47 cases), followed by “near total” resection (pRS1 in 4 cases), partial resection (pRS2 in 11 cases), and biopsy (pRS3 in 2 cases) (Table 1).
During the surgery, microscopy and US techniques were integrated to estimate the extent of resection. The US techniques were used in all cases (Figures 1,2). Once the information is provided by integrating the microscopy and US techniques accorded with the pRS, the surgery could be completed.
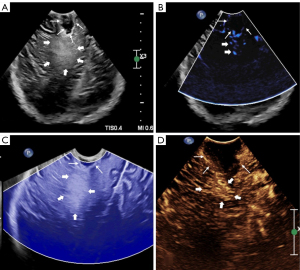
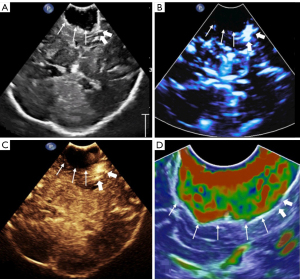
Two sonographers and two MRI radiologists randomly selected 10 lesions in each group to independently evaluate the grade. There was a good correlation between the observers’ evaluations of the grade for each US technique and MRI (rb=0.855, P<0.0001; rs=0.811, P<0.0001; rs=0.811, P<0.0001; rm=0.841, P<0.0001; rc=0.855, P<0.0001; rM=0.845, P<0.0001).
Compared to the MRI results, all the included US technologies showed substantial agreement. The Kappa values were 0.717, 0.751, 0.714, and 0.892 for B-mode, MFI, SWE, and CEUS, respectively. CEUS and MRI had the best diagnostic consistency (Table 3). In general, CEUS underestimated the tumor residual. B-mode, MFI, and SWE can both underestimate and overestimate the tumor residual. CEUS had the highest sensitivity, specificity, positive predictive value, and negative predictive value under the receiver operating characteristic (ROC) curve analysis (77.78%, 100%, 100%, and 86.05%, respectively) (Table 4).
Table 3
Technic | RS0 | RS1 | RS2 | RS3 | Kappa |
---|---|---|---|---|---|
MRI | 37 | 13 | 12 | 2 | – |
B-mode | 36 | 15 | 11 | 2 | 0.717 |
MFI | 38 | 13 | 11 | 2 | 0.751 |
SWE | 40 | 11 | 11 | 2 | 0.714 |
CEUS | 42 | 9 | 11 | 2 | 0.892 |
CEUS, contrast-enhanced ultrasound; MRI, magnetic resonance imaging; MFI, micro-flow imaging; SWE, shear wave elastography.
Table 4
Technic | Sensitivity | Specificity | Positive predictive value | Negative predictive value |
---|---|---|---|---|
B-mode | 70.37% | 78.38.5% | 78.38% | 70.37% |
MFI | 70.37% | 83.78% | 76% | 79.49% |
SWE | 62.96% | 83.78% | 73.91% | 75.61% |
CEUS | 77.78% | 100% | 100% | 86.05% |
CEUS, contrast-enhanced ultrasound; MRI, magnetic resonance imaging; MFI, micro-flow imaging; SWE, shear wave elastography.
To analyze the factors affecting the evaluation accuracy of the US techniques, a Pearson correlation analysis was conducted. The results showed that only the number of lesions was associated with discordance in the two imaging tests for B-mode, MFI, and SWE (P=0.011, 0.020, and 0.015, respectively). In relation to those three techniques, no correlations were found in relation to the other clinical variables, including age, gender, histology, tumor size, lesion location, boundaries, shape, and edema zone around the tumor. In relation to CEUS, only tumor size was found to be correlated with evaluation accuracy (P=0.021) (Table 5).
Table 5
Factor | P value | |||
---|---|---|---|---|
B-mode | MFI | SWE | CEUS | |
Age | 0.162 | 0.086 | 0.061 | 0.584 |
Gender | 0.887 | 0.469 | 0.313 | 0.937 |
Histology | 0.127 | 0.307 | 0.201 | 0.056 |
Lesion location | >0.999 | 0.823 | 0.913 | 0.339 |
Numbers of lesions | 0.011* | 0.020* | 0.015* | 0.162 |
Tumor size | 0.238 | 0.359 | 0.167 | 0.021* |
Boundaries | >0.999 | 0.823 | 0.913 | 0.339 |
Shape | 0.302 | 0.445 | 0.368 | 0.751 |
Edema zone around the tumor | 0.867 | 0.844 | 0.725 | 0.687 |
*, statistically significant. CEUS, contrast-enhanced ultrasound; MFI, micro-flow imaging; SWE, shear wave elastography.
Discussion
IOUS is widely used in neurosurgery, but new US techniques are also being employed (7,8,11-22). Several studies have evaluated the role of these new US techniques in assessing tumor residual in the procedure. He et al. used B-mode, CDFI, and CEUS to evaluate tumor residual in brain surgery, and found that CEUS was better able to distinguish between the border of the tumor and the remaining tumor tissue, and the healthy brain tissue than conventional US during the operation (24). Cepeda et al. reported that tumor stiffness measured by IOUS strain elastography was correlated with histopathology (25). Tao et al. explored the use of CEUS in the removal of remnants surrounding the resection cavity in 51 patients, and found that the total removal rate of the CEUS group (23/28, 82%) was significantly higher than that of the US group (11/23, 48%) (P<0.05) and the recurrence rate was lower [18% (5/23) in the CEUS group vs. 22% (5/28) in the US group, P>0.05] (26). Yu et al. evaluated the diagnostic significance of CEUS in estimating the resection degree of brain glioma, and found that IOUS contrast has high sensitivity (62.2%) and specificity (92.8%) in evaluating the excision degree of tumors (32). Chan et al. studied the clinical application of SWE in assisting brain tumor resection, and found that the sensitivities of residual tumor detection by the surgeon, US B-mode, and SWE were 36%, 73% and 94%, respectively, while their specificities were 100%, 63%, and 77%, respectively (34). However, no comparative study had previously been conducted on the role of different new US technologies in guiding brain tumor resection.
The present study compared the roles of different US technologies in guiding brain tumor resection. We found that compared to the MRI results, all the included US technologies showed substantial agreement. The Kappa values were 0.717, 0.751, and 0.714, and 0.892 for B-mode, MFI, SWE, and CEUS, respectively. CEUS and MRI had the best diagnostic consistency. CEUS had the highest sensitivity, specificity, positive predictive value, and negative predictive value under the ROC curve analysis (77.78%, 100%, 100%, and 86.05%, respectively), followed by MFI. B-mode and SWE had similar accuracy in detecting tumor residue. Thus, the use of CEUS in IOUS may be beneficial in reducing tumor residue and recurrence rates, and improving the quality of life of patients. Further research on CEUS in IOUS could provide insights into the advantages and disadvantages of CEUS in practical applications and improve its related parameters.
MFI is a novel US technique that enables the detection of slow-velocity flow, providing the visualization of the blood flow in small vessels without the need of intravenous contrast agent administration (35,36). The applications of MFI in the clinic have increased in recent years due to the quality of this non-invasive, swift, and user-friendly technique. Moreover, it has been shown to be useful in tumor diagnosis and the treatment of related conditions, including cervical cancer, head and neck malignancies, and thyroid tumors (37-40). However, no study on the use of MFI in neurosurgery had previously been conducted. Our team used MFI to evaluate tumor residual in neurosurgery. The concordance analysis between MFI and MRI showed substantial agreement (kappa =0.751). The diagnostic efficiency of MFI was the second best after CEUS (sensitivity, specificity, positive predictive value, and negative predictive value under the ROC curve analysis: 70.37%, 83.78%, 76%, and 79.49%, respectively). Compared to CEUS, MFI is non-invasive, swift, and user-friendly. Thus, MFI can be recommended as a technique for guiding and evaluating tumor residual in neurosurgery in addition to CEUS. CEUS can be used when the MFI is unable to distinguish tumor residue.
There are several artifacts related to surgery, including acoustic shadowing from surgical, post-surgical, and post-radiation artifacts, post-resection hyperechoic rim, and hyperechoic blood. CEUS can overcome the limitations of B-mode, MFI, and SWE in identifying these artifacts. This may be the reason for the high diagnostic efficiency of CEUS. In our study, B-mode overestimated resection in 8 cases and underestimated resection in 8 cases, SWE overestimated resection in 6 cases and underestimated resection in 9 cases, and MFI, overestimated resection in 6 cases and underestimated resection in 8 cases. Conversely, CEUS only underestimated resection in 6 cases.
We evaluated the relevant factors of residual misdiagnosis caused by these US techniques. The results showed that only the number of lesions was associated with discordance in the two imaging tests for B-mode, MFI, and SWE. In relation to CEUS, only tumor size was correlated with evaluation accuracy. Frassanito et al. reported similar results (16). This may be due to the collapsed surgical cavity, especially when the ventricular system is opened, and by artifacts that may simulate or hide residual tumors. Based on our analysis, all cases with residual tumors misdiagnosed by CEUS had peripheral edema zones. However, no statistically significant results were found in relation to the relationship between the misdiagnosis of CEUS and the surrounding edema zone. However, this might have been related to the small sample size of the study.
No major complications related to US arose in our study. US is more convenient and cost-effective than MRI and CT. In addition, US has no radiation.
This study had some limitations. The sample size was relatively small. Thus, our findings and conclusions must be considered preliminary. Further studies need to be conducted with a larger variety and number of cases. Additionally, the relevant factors of residual misdiagnosis caused by the US techniques need to be studied, and the accuracy of residual diagnosis by US should be improved.
Conclusions
US is a convenient and cost-effective method for guiding the procedure and evaluating the extent of resection in neurosurgery. CEUS has the highest diagnostic accuracy for residual lesions among the new US technologies. MFI can be recommended as a technique for guiding and evaluating residuals in neurosurgery in addition to CEUS.
Acknowledgments
None.
Footnote
Reporting Checklist: The authors have completed the STARD reporting checklist. Available at https://qims.amegroups.com/article/view/10.21037/qims-24-2271/rc
Funding: This study was funded by
Conflicts of Interest: All authors have completed the ICMJE uniform disclosure form (available at https://qims.amegroups.com/article/view/10.21037/qims-24-2271/coif). The authors have no conflicts of interest to declare.
Ethical Statement: The authors are accountable for all aspects of the work in ensuring that questions related to the accuracy or integrity of any part of the work are appropriately investigated and resolved. The study was conducted in accordance with the Declaration of Helsinki (as revised in 2013). The study was approved by the Institutional Ethics Board of Sichuan Cancer Hospital (No. SCCHEC-02-2023-071), and informed consent was obtained from all individual participants.
Open Access Statement: This is an Open Access article distributed in accordance with the Creative Commons Attribution-NonCommercial-NoDerivs 4.0 International License (CC BY-NC-ND 4.0), which permits the non-commercial replication and distribution of the article with the strict proviso that no changes or edits are made and the original work is properly cited (including links to both the formal publication through the relevant DOI and the license). See: https://creativecommons.org/licenses/by-nc-nd/4.0/.
References
- Nabors LB, Portnow J, Ammirati M, Brem H, Brown P, Butowski N, et al. Central nervous system cancers, version 2.2014. Featured updates to the NCCN Guidelines. J Natl Compr Canc Netw 2014;12:1517-23. [Crossref] [PubMed]
- Huang J, Li H, Yan H, Li FX, Tang M, Lu DL. The comparative burden of brain and central nervous system cancers from 1990 to 2019 between China and the United States and predicting the future burden. Front Public Health 2022;10:1018836. [Crossref] [PubMed]
- Hou X, Song Z, Zhang F, Liu Z, Long W, Long Z, Zhou M, Wang E, Yin P, Zhu M. Burden of brain and other central nervous system cancer in China, 1990-2019: a systematic analysis of observational data from the global burden of disease study 2019. BMJ Open 2022;12:e059699. [Crossref] [PubMed]
- Barbagallo GM, Maione M, Peschillo S, Signorelli F, Visocchi M, Sortino G, Fiumanò G, Certo F. Intraoperative computed tomography, navigated ultrasound, 5-amino-levulinic acid fluorescence and neuromonitoring in brain tumor surgery: overtreatment or useful tool combination? J Neurosurg Sci 2024;68:31-43. [Crossref] [PubMed]
- Trevisi G, Barbone P, Treglia G, Mattoli MV, Mangiola A. Reliability of intraoperative ultrasound in detecting tumor residual after brain diffuse glioma surgery: a systematic review and meta-analysis. Neurosurg Rev 2020;43:1221-33. [Crossref] [PubMed]
- Chacko AG, Kumar NK, Chacko G, Athyal R, Rajshekhar V. Intraoperative ultrasound in determining the extent of resection of parenchymal brain tumours--a comparative study with computed tomography and histopathology. Acta Neurochir (Wien) 2003;145:743-8; discussion 748. [Crossref] [PubMed]
- El Beltagy MA, Aggag M, Kamal M. Role of intraoperative ultrasound in resection of pediatric brain tumors. Childs Nerv Syst 2010;26:1189-93. [Crossref] [PubMed]
- Neidert MC, Hostettler IC, Burkhardt JK, Mohme M, Held U, Kofmehl R, Eisele G, Woernle CM, Regli L, Bozinov O. The influence of intraoperative resection control modalities on survival following gross total resection of glioblastoma. Neurosurg Rev 2016;39:401-9. [Crossref] [PubMed]
- Incekara F, Smits M, Dirven L, Bos EM, Balvers RK, Haitsma IK, Schouten JW, Vincent AJPE. Intraoperative B-Mode Ultrasound Guided Surgery and the Extent of Glioblastoma Resection: A Randomized Controlled Trial. Front Oncol 2021;11:649797. [Crossref] [PubMed]
- Eyüpoglu IY, Hore N, Savaskan NE, Grummich P, Roessler K, Buchfelder M, Ganslandt O. Improving the extent of malignant glioma resection by dual intraoperative visualization approach. PLoS One 2012;7:e44885. [Crossref] [PubMed]
- Bal J, Camp SJ, Nandi D. The use of ultrasound in intracranial tumor surgery. Acta Neurochir (Wien) 2016;158:1179-85. [Crossref] [PubMed]
- Sastry R, Bi WL, Pieper S, Frisken S, Kapur T, Wells W 3rd, Golby AJ. Applications of Ultrasound in the Resection of Brain Tumors. J Neuroimaging 2017;27:5-15. [Crossref] [PubMed]
- Regelsberger J, Lohmann F, Helmke K, Westphal M. Ultrasound-guided surgery of deep seated brain lesions. Eur J Ultrasound 2000;12:115-21. [Crossref] [PubMed]
- de Quintana-Schmidt C, Salgado-Lopez L, Aibar-Duran JA, Alvarez Holzapfel MJ, Cortes CA, Alvarado JDP, Rodriguez RR, Teixidó JM. Neuronavigated Ultrasound in Neuro-Oncology: A True Real-Time Intraoperative Image. World Neurosurg 2022;157:e316-26. [Crossref] [PubMed]
- Ohue S, Kumon Y, Nagato S, Kohno S, Harada H, Nakagawa K, Kikuchi K, Miki H, Ohnishi T. Evaluation of intraoperative brain shift using an ultrasound-linked navigation system for brain tumor surgery. Neurol Med Chir (Tokyo) 2010;50:291-300. [Crossref] [PubMed]
- Frassanito P, Stifano V, Bianchi F, Tamburrini G, Massimi L. Enhancing the Reliability of Intraoperative Ultrasound in Pediatric Space-Occupying Brain Lesions. Diagnostics (Basel) 2023.
- Pino MA, Imperato A, Musca I, Maugeri R, Giammalva GR, Costantino G, Graziano F, Meli F, Francaviglia N, Iacopino DG, Villa A. New Hope in Brain Glioma Surgery: The Role of Intraoperative Ultrasound. A Review. Brain Sci 2018;8:202. [Crossref] [PubMed]
- Carai A, De Benedictis A, Calloni T, Onorini N, Paternò G, Randi F, Colafati GS, Mastronuzzi A, Marras CE. Intraoperative Ultrasound-Assisted Extent of Resection Assessment in Pediatric Neurosurgical Oncology. Front Oncol 2021;11:660805. [Crossref] [PubMed]
- Mathon B, Clemenceau S, Carpentier A. Intraoperative Ultrasound Shear-Wave Elastography in Focal Cortical Dysplasia Surgery. J Clin Med 2021;10:1049. [Crossref] [PubMed]
- Cepeda S, García-García S, Arrese I, Sarabia R. Non-navigated 2D intraoperative ultrasound: An unsophisticated surgical tool to achieve high standards of care in glioma surgery. J Neurooncol 2024;167:387-96. [Crossref] [PubMed]
- Cepeda S, García-García S, Velasco-Casares M, Fernández-Pérez G, Zamora T, Arrese I, Sarabia R. Is There a Relationship between the Elasticity of Brain Tumors, Changes in Diffusion Tensor Imaging, and Histological Findings? A Pilot Study Using Intraoperative Ultrasound Elastography. Brain Sci 2021;11:271. [Crossref] [PubMed]
- Mathon B, Amelot A, Carpentier A, Clemenceau S. Intraoperative real-time guidance using ShearWave Elastography for epilepsy surgery. Seizure 2019;71:24-7. [Crossref] [PubMed]
- Prada F, Perin A, Martegani A, Aiani L, Solbiati L, Lamperti M, Casali C, Legnani F, Mattei L, Saladino A, Saini M, DiMeco F. Intraoperative contrast-enhanced ultrasound for brain tumor surgery. Neurosurgery 2014;74:542-52; discussion 552. [Crossref] [PubMed]
- He W, Jiang XQ, Wang S, Zhang MZ, Zhao JZ, Liu HZ, Ma J, Xiang DY, Wang LS. Intraoperative contrast-enhanced ultrasound for brain tumors. Clin Imaging 2008;32:419-24. [Crossref] [PubMed]
- Cepeda S, García-García S, Arrese I, Velasco-Casares M, Sarabia R. Advantages and Limitations of Intraoperative Ultrasound Strain Elastography Applied in Brain Tumor Surgery: A Single-Center Experience. Oper Neurosurg (Hagerstown) 2022;22:305-14. [Crossref] [PubMed]
- Tao AY, Chen X, Zhang LY, Chen Y, Cao D, Guo ZQ, Chen J. Application of Intraoperative Contrast-Enhanced Ultrasound in the Resection of Brain Tumors. Curr Med Sci 2022;42:169-76. [Crossref] [PubMed]
- Prada F, Del Bene M, Casali C, Saladino A, Legnani FG, Perin A, Moiraghi A, Richetta C, Rampini A, Mattei L, Vetrano IG, Fornaro R, Saini M, Martegani A, DiMeco F. Intraoperative Navigated Angiosonography for Skull Base Tumor Surgery. World Neurosurg 2015;84:1699-707. [Crossref] [PubMed]
- Ilunga-Mbuyamba E, Avina-Cervantes JG, Lindner D, Cruz-Aceves I, Arlt F, Chalopin C. Vascular Structure Identification in Intraoperative 3D Contrast-Enhanced Ultrasound Data. Sensors (Basel) 2016.
- Mattei L, Prada F, Legnani FG, Perin A, Olivi A, DiMeco F. Neurosurgical tools to extend tumor resection in hemispheric low-grade gliomas: conventional and contrast enhanced ultrasonography. Childs Nerv Syst 2016;32:1907-14. [Crossref] [PubMed]
- Prada F, Ciocca R, Corradino N, Gionso M, Raspagliesi L, Vetrano IG, Doniselli F, Del Bene M, DiMeco F. Multiparametric Intraoperative Ultrasound in Oncological Neurosurgery: A Pictorial Essay. Front Neurosci 2022;16:881661. [Crossref] [PubMed]
- Shi J, Zhang Y, Yao B, Sun P, Hao Y, Piao H, Zhao X. Application of Multiparametric Intraoperative Ultrasound in Glioma Surgery. Biomed Res Int 2021;2021:6651726. [Crossref] [PubMed]
- Yu SQ, Wang JS, Chen SY, Liu XM, Li Y, Ding YM, Li XY, Sun YL, Chen H. Diagnostic significance of intraoperative ultrasound contrast in evaluating the resection degree of brain glioma by transmission electron microscopic examination. Chin Med J (Engl) 2015;128:186-90. [Crossref] [PubMed]
- Giammalva GR, Ferini G, Musso S, Salvaggio G, Pino MA, Gerardi RM, Brunasso L, Costanzo R, Paolini F, Di Bonaventura R, Umana GE, Graziano F, Palmisciano P, Scalia G, Tumbiolo S, Midiri M, Iacopino DG, Maugeri R. Intraoperative Ultrasound: Emerging Technology and Novel Applications in Brain Tumor Surgery. Front Oncol 2022;12:818446. [Crossref] [PubMed]
- Chan HW, Uff C, Chakraborty A, Dorward N, Bamber JC. Clinical Application of Shear Wave Elastography for Assisting Brain Tumor Resection. Front Oncol 2021;11:619286. [Crossref] [PubMed]
- Aziz MU, Eisenbrey JR, Deganello A, Zahid M, Sharbidre K, Sidhu P, Robbin ML. Microvascular Flow Imaging: A State-of-the-Art Review of Clinical Use and Promise. Radiology 2022;305:250-64. [Crossref] [PubMed]
- Bartolotta TV, Taibbi A, Randazzo A, Gagliardo C. New frontiers in liver ultrasound: From mono to multi parametricity. World J Gastrointest Oncol 2021;13:1302-16. [Crossref] [PubMed]
- Lu R, Meng Y, Zhang Y, Zhao W, Wang X, Jin M, Guo R. Superb microvascular imaging (SMI) compared with conventional ultrasound for evaluating thyroid nodules. BMC Med Imaging 2017;17:65. [Crossref] [PubMed]
- de Koekkoek-Doll PK, Roberti S, van den Brekel MW, Maas M, Smit L, Beets-Tan R, Castelijns J. Value of Assessing Peripheral Vascularization with Micro-Flow Imaging, Resistive Index and Absent Hilum Sign as Predictor for Malignancy in Lymph Nodes in Head and Neck Squamous Cell Carcinoma. Cancers (Basel) 2021.
- Song Q, Kang LL, Lan Y, Yan L, Li W, Ren L, Luo YK. Application of Micro-flow Imaging in the Differentiation of Benign and Malignant Thyroid Nodules. Zhongguo Yi Xue Ke Xue Yuan Xue Bao 2022;44:40-44. [PubMed]
- Zhu Y, Tang Y, Zhang G, Zhang J, Li Y, Jiang Z. Quantitative analysis of superb microvascular imaging for monitoring tumor response to chemoradiotherapy in locally advanced cervical cancer. Front Oncol 2022;12:1074173. [Crossref] [PubMed]