Effect of a breathing exercise program on coronary artery motion artifacts in 256 rows of coronary computed tomography angiography in patients with different heart rate variability
Introduction
Over the past few years, coronary computed tomography angiography (CCTA) has been used as the preferred first-line testing strategy in patients without known coronary artery disease (1-4). However, coronary motion artifacts have been a major limitation of this technique due to the small size of the heart, rapid motion, and tortuous coronary arteries.
Respiratory motion, high heart rate (HR), and HR variability (HRV) are the main sources of motion artifacts (5,6). Traditionally, beta-blockers have been requested before the examination to control HR and HRV, and the patient has been asked to hold their breath during the scan to minimize motion artifacts (7). However, the inability of some patients to hold their breath (e.g., deafness, impaired hearing, communication difficulties, severe lung disease, lack of cooperation), as well as contraindications to beta-blockers and lack of therapeutic response, prevent effective control of HR and HRV in some patients. This has resulted in limiting CCTA testing in patients with respiratory motion, high HR, and high HRV (3,8,9). To date, several articles have been reported on the possibility of free-breathing protocols for CCTA examination, including low HR 320-detector computed tomography (CT) and dual-source CT (10,11), varying HR (12), and varying HR and rhythm (13). However, the investigation of patients with varying HR and rhythm was limited to 11 patients and the amount of data was too low to be convincing. A major limitation of these studies was the restriction on HR stabilization, because HR instability can produce banding artifacts or step artifacts (6), which affect the image quality in CCTA (3). One aspect of the solution is the use of beta-blockers to control HR prior to examination; however, contraindications to beta-blockers and the lack of therapeutic response to them in some patients restrict the utility of beta-blockers in reducing HR instability (3). Meanwhile, the retrospective cardiac-gated spiral scanning mode can be chosen to eliminate the effect of motion artifacts produced by HRV on image quality at the cost of increased radiation dose (14).
With the introduction of 256-row spiral CT, the time resolution has been increased to 140 ms, and the detector width has reached 16 cm, making it possible to cover the entire heart without moving the scanning bed and to complete the data acquisition in a single heartbeat. Whether technological advances can reduce or avoid motion artifacts produced by respiratory motion and high HRV requires further investigation. Therefore, this study aimed to assess the effect of respiratory status on coronary motion artifacts under different HRV conditions using a 256-row, 16-cm-wide detector CT system. We present this article in accordance with the STROBE reporting checklist (available at https://qims.amegroups.com/article/view/10.21037/qims-24-1253/rc).
Methods
Study population
The study was conducted in accordance with the Declaration of Helsinki (as revised in 2013). The study was approved by the Ethics Committee of Affiliated BenQ Hospital of Nanjing Medical University (No. 2022-KL002) and all patients provided written informed consent before participating in the study. Between January 2022 and January 2024, 1,109 consecutive patients undergoing CCTA were included in the study. Contraindications to CCTA adhered strictly to the requirements of the Society of Cardiovascular Computed Tomography (SCCT) guidelines (9), and patients who could not hold their breath as required were excluded. Further, coronary stent and coronary artery bypass graft (CABG) patients were excluded from this study because of the blooming artifacts of CCTA metal stents, which affect the assessment of motion artifacts, and because of the different CCTA scanning ranges of patients with CABGs, the latter of which require larger scanning ranges, resulting in increased radiation exposure of the patients. Patients were randomized into two groups using a computer-generated random number table (by Python 3): group A (breath-holding protocol) and group B (free-breathing protocol). Patients in group A received respiratory training, whereas those in group B were asked to breathe normally during the examination. In group A, 30 patients with stent implantation, two patients with bypass, and 102 patients who could not hold their breath as required were excluded, and a total of 400 patients were included. In group B, 37 patients with stent implantation and three patients with bypass were excluded, and a total of 535 patients were included. additional beta-blockers or nitroglycerin were used in this study. Each group was further divided into three subgroups of low HRV (HRV ≤3 bpm), intermediate HRV (HRV 4–10 bpm), and high HRV (HRV ≥11 bpm) based on the HRV information recorded by electrocardiogram (ECG) during the scanning period.
Scanning programs and injection protocols
All patients were scanned using a 256-row, 16-cm-wide detector CT scanner (Revolution APEX CT, GE Healthcare, Waukesha, WI, USA) in one heartbeat using a prospective ECG-triggered axial scanning mode. The phase windows for HR <65, 65–80, and >80 bpm were set between 65% and 80%, 30% and 80%, and 35% and 50%, respectively. The 100, 120, or 140 kV tube voltage was selected according to the patient’s body mass index (BMI), with automatic tube current modulation (200–800 mA) and a noise index (NI) preset to 22 Hounsfield units (HU). The scanning range was from the level of the tracheal bifurcation to the base of the heart, and the width of the detector was adjusted to 12, 14, or 16 cm depending on the size of the patient’s heart. 0.28 seconds gantry rotation time, 0.625 mm slice thickness/reconstruction interval, 512×512 reconstruction matrix. Smart phase technology was applied during the examination for automated cardiac tensegrity selection, and images were reconstructed using deep learning reconstruction algorithms (GE Healthcare) and second-generation motion correction algorithms (MCA2, GE Healthcare).
A quantity of 50 mL of preheated (37 ℃) contrast Omnipaque (350 mgI/mL, GE Healthcare) was injected at 5 mL/s into the anterior elbow vein using a dual-tip CT power injector (Stellant, Bayer, Leverkusen, Germany), followed by 40 mL of saline-flush contrast at the same flow rate. To achieve adequate contrast enhancement in the coronary arteries, a push-injection tracking technique was used with the region of interest (ROI) located in the descending aorta and a trigger threshold of 200 HU. A short breath command (“please hold your breath”) was also used, which took 3.2 seconds to ensure that the breath-hold CCTA examination was completed within the 4-second post-trigger delay (group A).
HR and HRV
Minimum HR (HRMin), average HR, maximum HR (HRMax), and rhythm were recorded for each cardiac cycle based on ECG information recorded during CT acquisition. HR was defined as the average HR recorded during the cardiac cycle. HRV was defined as the highest HR minus the lowest HR recorded during the cardiac cycle, specifically, HRV = HRMax − HRMin, where HRMax is recorded by ECG and HRMin is recorded by ECG (15).
Respiratory movement assessment
Respiratory motion was defined as pulmonary vasomotion. Pulmonary vasomotion was defined as a seagull artifact, double-bordered appearance, or pulmonary vascular opacification in lung window images (16). Two experienced readers (C.Y. and Y.G.), with 10 and 8 years of clinical experience, respectively, blindly assessed respiratory motion on CCTA images of the lung base without knowing the patient’s respiratory status. Meanwhile, the lung motion status was graded according to the four regions at the base of the lungs, namely, the right middle lobe, the right lower lobe, the lingual segment of the left upper lobe, and the left lower lobe, with mild lung motion defined as the presence of lung motion in only one of the four regions, moderate lung motion defined as the presence of lung motion artifacts in two to three of the four regions, and severe lung motion defined as the presence of lung motion artifacts in all four regions. When the opinions of the two readers initially differed, the consensus was reached in a shared reading, as displayed in Figure 1.
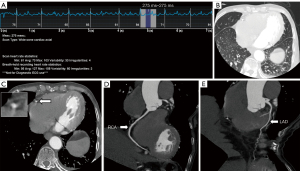
Image quality analysis
Subjective image quality assessment
Image quality was independently and blindly assessed by two experienced readers (C.C. and J.M.) who have 10 and 15 years of clinical experience in CCTA performance and analysis, respectively. Coronary artery segments were evaluated using the SCCT 18-segment model coronary artery segments (17), and coronary artery diameters ≤1.5 mm, lumen occlusion, and diffuse severe calcification of coronary artery segments were excluded from the evaluation. According to previous study, motion artifacts mainly affect the mid-right coronary artery (RCA), mid-left anterior descending artery (LAD), and distal LAD (18); therefore, in the present study, we mainly evaluated the proximal, middle, and distal segments of the RCA, the left main trunk, the proximal, middle, and distal segments of the anterior descending branch of the left coronary artery, and the proximal and distal segments of the left circumflex branch, which comprised nine segments. A four-point Likert scale (19) was used to score subjective image quality: 4, excellent, no artifacts; 3, good, mild artifacts; 2, acceptable, moderate artifacts are present but the image is still interpretable; and 1, unassessable, severe artifacts make interpretation impossible. The distribution of subjective scores was expressed as 4/3/2/1. When two readers’ scores were initially different, consensus was reached via a shared reading.
Objective image quality assessment
The CT attenuations and standard deviations (SDs) of the aortic root at the level of the flat left main coronary artery (LM) were measured by a radiological cardiovascular surgeon with more than 10 years of experience. The SD value of the aortic root was used as the CCTA image noise. Measurements were taken at the maximum area of the vessel, while avoiding the vessel wall, calcified and non-calcified plaques, and three measurements were taken at a time and averaged.
Radiation dose measurement
The dose length product (DLP) of the patient was recorded, and the effective radiation dose (ED) was calculated as ED (mSv) = DLP × K, where K is the chest conversion factor, K=0.014 mSv/(mGy·cm) (20).
Statistical analysis
Continuous variables were expressed as mean ± SD and categorical variables were expressed as frequencies or percentages. According to the results of the Kolmogorov-Smirnov test, continuous normal variables were analyzed with the independent samples t-test, non-normal variables were analyzed with the Mann-Whitney U test, and the Chi-squared test was used to study differences in categorical data. Interobserver agreement was analyzed using the kappa test (k values less than 0.20 were considered to indicate slight agreement; k values of 0.21–0.40, fair agreement; k values of 0.41–0.60, moderate agreement; and k values of 0.61–0.80, substantial agreement; (k values of 0.81 or greater, almost perfect agreement). A P value <0.05 indicated a statistically significant difference; P referred to the probability that the null hypothesis of the statistical test will occur. All statistical analyses were performed using the software SPSS 20.0 (IBM Corp., Armonk, NY, USA).
Results
Baseline characteristics
Of the 400 patients in group A, the mean age was 57.5 years, with an age range of 25 to 96 years. Of the 535 patients in group B, the mean age was 57.5 years, with an age range of 25 to 93 years. There was no statistical difference between the two groups in terms of gender, height, weight, BMI, and HR (all P>0.05). There was a statistically significant difference in HRV between the two groups and their subgroups of patients, with group A being significantly smaller than group B (all P<0.05) (see Table 1).
Table 1
Parameters | Group A (n=400) | Group B (n=535) | P value |
---|---|---|---|
Age (years) | 57.5±12.5 [25–96] | 57.5±13.8 [25–93] | 0.959 |
Female sex | 183 (45.8) | 218 (40.7) | 0.126† |
Height (cm) | 166.0±8.6 | 166.7±8.5 | 0.238 |
Weight (kg) | 69.1±13.8 | 70.1±13.5 | 0.226 |
BMI (kg/m2) | 24.9±3.6 | 25.1±3.5 | 0.553 |
HR | |||
Overall | 70.5±31.9 | 68.7±13.7 | 0.244 |
HR (HRV ≤3 bpm) | 66.9±11.0 | 68.2±16.5 | 0.532 |
HR (HRV 4–10 bpm) | 69.4±12.5 | 67.3±11.6 | 0.119 |
HR (HRV ≥11 bpm) | 71.5±11.7 | 70.8±15.6 | 0.735 |
HR (65–80 bpm) | 188 (47.0) | 217 (40.5) | <0.001 |
HRV | |||
Overall | 10.3±22.2 | 20.9±39.7 | <0.001 |
HRV ≤3 bpm | 2.08±0.82 | 2.57±0.66 | <0.001 |
HRV 4–10 bpm | 5.59±1.58 | 5.95±1.62 | 0.032 |
HRV ≥11 bpm | 34.85±36.82 | 51.79±57.01 | 0.005 |
Radiation dose (mSv) | |||
HRV ≤3 bpm | 3.62±1.39 | 2.91±1.17 | <0.001 |
HRV 4–10 bpm | 4.01±1.31 | 3.54±1.16 | <0.001 |
HRV ≥11 bpm | 4.23±1.21 | 3.72±1.07 | 0.001 |
Image noise (HU) | |||
HRV ≤3 bpm | 15.3±2.5 | 14.9±3.0 | 0.217 |
HRV 4–10 bpm | 15.5±2.9 | 15.1±2.6 | 0.247 |
HRV ≥11 bpm | 15.5±2.9 | 15.3±2.9 | 0.579 |
Data are presented as mean ± SD [range], n (%), or mean ± SD. †, Chi-squared test. Group A: breath-holding; Group B: free-breathing. BMI, body mass index; HR, heart rate; HRV, heart rate variability; HU, Hounsfield units; SD, standard deviation.
Breathing movement assessment
A total of 102 cases were evaluated for respiratory exercise in group A, accounting for 102/400 (yes/no); a total of 213 patients were evaluated for respiratory exercise in group B, accounting for 213/322 (yes/no), and the number of patients with respiratory exercise in group A was significantly lower than that in group B, with a statistically significant difference (P<0.001). In group A, the number of patients without respiratory motion was 187, 133, and 80 for HRV ≤3, HRV 4–10, and HRV ≥11 bpm, respectively (see Table 2). In group B, the number of patients in mild, moderate, and severe respiratory exercise condition was 22, 20, and 21 in HRV ≤3 bpm, 25, 71, and 36 in HRV 4–10 bpm and 27, 42, and 28 in HRV ≥11 bpm. The above data indicate that a small proportion of patients undergoing breath-holding protocols were unable to hold their breath for various reasons, as well as that the free-breathing state included both respiratory movement and respiratory quiescence, but the percentage of respiratory quiescence patients in free-breathing protocols was significantly lower than that of respiratory quiescence patients in the breath-holding protocols (P<0.001), as shown in Table 3. Analysis of the consistency of the respiratory movements of the two observers showed k=0.802, indicating general agreement, see Table 4.
Table 2
Parameters | Group A | Group B | P value | |||||
---|---|---|---|---|---|---|---|---|
Number | Score distribution (4/3/2/1) | Avg. score | Number | Score distribution (4/3/2/1) | Avg. score | |||
Overall | 400 | 3,349/65/32/28 | 3.94±0.35 | 535 | 4,437/104/40/24 | 3.94±0.34 | 0.584 | |
HRV ≤3 bpm | 187 | 1,575/29/11/10 | 3.95±0.31 | 75 | 624/13/5/1 | 3.95±0.32 | 0.650 | |
HRV 4–10 bpm | 133 | 1,113/22/13/11 | 3.93±0.37 | 279 | 2,332/41/19/13 | 3.94±0.32 | 0.229 | |
HRV ≥11 bpm | 80 | 661/14/8/7 | 3.92±0.38 | 181 | 1,481/50/16/10 | 3.92±0.37 | 0.435 |
Data are presented as frequency distribution tables or mean ± SD. Group A: breath-holding; Group B: free-breathing. Avg., average; HRV, heart rate variability; SD, standard deviation.
Table 3
Parameters | Group A | Group B | P value | |||||
---|---|---|---|---|---|---|---|---|
B1 | B2 | B3 | B1 vs. A | B2 vs. A | B3 vs. A | |||
Subjective scoring | ||||||||
HRV ≤3 bpm | 3.95±0.31 | 3.94±0.35 | 3.97±0.20 | 3.89±0.53 | 0.823 | 0.409 | 0.113 | |
HRV 4–10 bpm | 3.93±0.37 | 3.93±0.35 | 3.95±0.31 | 3.86±0.54 | 0.935 | 0.196 | 0.064 | |
HRV ≥11 bpm | 3.92±0.38 | 3.87±0.54 | 3.92±0.36 | 3.90±0.39 | 0.154 | 0.971 | 0.447 | |
Image noise (HU) | ||||||||
HRV ≤3 bpm | 15.3±2.5 | 15.1±2.3 | 15.3±2.5 | 14.8±3.1 | 0.490 | 0.941 | 0.269 | |
HRV 4–10 bpm | 15.5±2.9 | 15.5±2.9 | 14.7±2.3 | 15.0±2.4 | 0.938 | 0.062 | 0.357 | |
HRV ≥11 bpm | 15.5±2.9 | 14.9±2.1 | 15.3±2.6 | 15.3±3.2 | 0.318 | 0.704 | 0.812 | |
Radiation dose (mSv) | ||||||||
HRV ≤3 bpm | 3.62±1.39 [187] | 2.86±1.17 [22] | 2.96±1.13 [20] | 2.69±0.98 [21] | 0.008 | 0.043 | 0.001 | |
HRV 4–10 bpm | 4.01±1.31 [132] | 3.50±1.09 [25] | 3.43±1.07 [71] | 3.27±1.02 [36] | 0.074 | 0.002 | 0.002 | |
HRV ≥11 bpm | 4.23±1.21 [80] | 3.82±1.31 [27] | 3.64±1.02 [42] | 3.41±0.86 [28] | 0.143 | 0.008 | 0.001 |
Data are presented as mean ± SD or mean ± SD [number]. Group A: breath-holding; Group B: free-breathing. B1: mild respiratory motion group; B2: moderate respiratory motion group; B3: severe respiratory motion group. HRV, heart rate variability; HU, Hounsfield units; SD, standard deviation.
Table 4
Parameters | Reader A | Reader B | Kappa value |
---|---|---|---|
Subjective scoring (4/3/2/1) | 7,781/190/60/48 | 7,795/140/88/56 | 0.729 |
Pulmonary movement (yes/no) | 298/739 | 346/691 | 0.802 |
Data are presented as frequency distribution tables.
Comparison of image quality between groups
Subjective image quality assessment
In group A, coronary artery diameters ≤1.5 mm (expressed as 0), occlusions, and severely calcified segments were excluded. A total of 1,625 coronary segments were analyzed in the HRV ≤3 bpm subgroup excluding 0=40, occlusion =8, and calcification =10; a total of 1,159 coronary segments were analyzed in the HRV 4–10 bpm subgroup excluding 0=24, occlusion =4, and calcification =10; and a total of 690 coronary segments were analyzed in the HRV ≥11 bpm subgroups excluding 0=30.
In group B, a total of 643 coronary segments were analyzed in the HRV ≤3 bpm subgroup excluding 0=14, occlusion =14, and calcification =4; a total of 2,405 coronary segments were analyzed in the HRV 4–10 bpm subgroup excluding 0=86, occlusion =8, and calcification =12; and a total of 1,557 coronary segments were analyzed in the HRV ≥11 bpm subgroup excluding 0=50, occlusion =12, and calcification =10.
The subjective motor score two-observation consistency analysis showed k=0.729, indicating general agreement (see Table 4). The distribution of scores between the two groups and their subgroups is shown in Table 2. There was no statistically significant difference in the comparison of image quality in group B of the free-breathing protocol compared to group A of the breath-holding protocol (3.94±0.35 vs. 3.94±0.34, P=0.584). When we compared the two respiratory protocols in the three subgroups of HRV ≤3 bpm (3.95±0.31 vs. 3.95±0.32, P=0.650), HRV 4–10 bpm (3.93±0.37 vs. 3.94±0.32, P=0.229), and HRV ≥11 bpm (3.92±0.38 vs. 3.92±0.37, P=0.435), there was no statistically significant (all P>0.05) (see Table 2 and Figure 2).
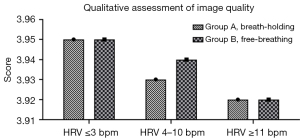
Respiratory motion was further classified as mild, moderate, and severe according to the degree of respiratory motion. With HRV ≤3 bpm, HRV 4–10 bpm, and HRV ≥11 bpm, there was no statistically significant difference between mild, moderate, and severe subjective image quality assessments in group B compared to group A (all P>0.05), as shown in Table 3.
Objective image quality assessment
There was no statistically significant difference (all P>0.05) in the comparison of image noise in group B of the free-breathing protocol compared to group A of the breath-holding protocol at HRV ≤3 bpm, HRV 4–10 bpm, and HRV ≥11 bpm, as shown in Table 1.
Further assessment of the objective image quality at different degrees of respiratory motion showed that there was no statistical difference (all P>0.05) in the comparison of image noise across mild, moderate, and severe degrees of respiratory motion in group B of the free-breathing protocol compared to group A of the breath-holding protocol with no respiratory motion, as shown in Table 3.
Within-group comparison of subjective image quality, HR, and HRV
Comparison of image quality within groups: within-group comparisons showed that image quality decreased with increasing HRV. The image quality of the low HRV (HRV ≤3 bpm), intermediate HRV (HRV 4–10 bpm), and high HRV (HRV ≥11 bpm) subgroups were 3.95±0.31, 3.93±0.36, and 3.92±0.37, respectively. Compared with the low HRV subgroup, the intermediate HRV and high HRV subgroups were not statistically different in comparison (both P>0.05).
Comparison within HRV groups: the HRVs of the low, medium, and high subgroups were 2.23±0.80, 5.83±1.61, and 46.5±52.1, respectively, and there was a statistically significant difference in the comparison of the medium and high HRV subgroups compared with the low HRV subgroups (both P<0.001).
Comparison of HR within groups: the HRs of the three low, intermediate, and high HRV subgroups were 67.2±13.2, 68.3±11.4, and 71.2±11.3 bpm, respectively. The HR of the subgroup of HRV ≤3 bpm was the lowest among the three A-groups, in comparison with which, only the HR of the subgroup of high HRV ≥11 bpm showed a statistically significant difference (both P<0.001), as shown in Table 5.
Table 5
Parameters | HRV | P value | ||||
---|---|---|---|---|---|---|
≤3 bpm (subgroup 1) | 4–10 bpm (subgroup 2) | ≥11 bpm (subgroup 3) | Subgroup 2 vs. 1 | Subgroup 3 vs. 1 | ||
Subjective scoring | 3.95±0.31 | 3.93±0.36 | 3.92±0.37 | 0.370 | 0.132 | |
Group A | 3.95±0.31 | 3.93±0.37 | 3.92±0.38 | 0.201 | 0.159 | |
Group B | 3.95±0.32 | 3.94±0.32 | 3.92±0.37 | 0.741 | 0.109 | |
HR (bpm) | 67.2±13.2 | 68.3±11.4 | 71.2±11.3 | 0.673 | 0.016 | |
Group A | 66.9±11.0 | 69.4±12.5 | 71.5±11.7 | 0.060 | 0.003 | |
Group B | 68.2±16.5 | 67.3±11.6 | 70.8±15.6 | 0.674 | 0.244 | |
HRV (bpm) | 2.23±0.80 | 5.83±1.61 | 46.5±52.1 | <0.001 | <0.001 | |
Group A | 2.08±0.82 | 5.59±1.58 | 34.85±36.82 | <0.001 | <0.001 | |
Group B | 2.57±0.66 | 5.95±1.62 | 51.79±57.01 | <0.001 | <0.001 |
Data are presented as mean ± SD. Group A: breath-holding; Group B: free-breathing. HR, heart rate; HRV, heart rate variability; SD, standard deviation.
The above data show that image quality is independent of HRV and HR.
Radiation dose assessment
The radiation dose to CCTA patients increased with increasing HRV. In the three subgroups of HRV ≤3 bpm, HRV 4–10 bpm, and HRV ≥11 bpm, the radiation doses in the breath-holding protocol (group A) were 3.62±1.39, 4.01±1.31, and 4.23±1.21 mSv, respectively. The radiation doses in the free-breathing protocol (group B) were 2.91±1.17, 3.54±1.16, and 3.72±1.07 mSv, respectively. The radiation dose in the free-breathing protocol was significantly lower than that in the breath-holding protocol, all of which were statistically significantly different in comparison (all P<0.001).
According to the degree of respiratory motion, there was no statistical difference in the comparison of mild respiratory motion in the case of HRV 4–10 bpm and HRV ≥11 bpm compared to group A without respiratory motion (both P>0.05), and the rest of the comparisons were not statistically different. This occurrence may be related to low data volume.
Discussion
We found that motion artifacts based on a 140-ms temporal resolution, 16-cm wide-body detector 256-row helical CT are not affected by respiratory motion and HRV, which enables free-breathing CCTA examinations under different HRV conditions without loss of image quality. Importantly, the free-breathing protocol reduces the radiation dose to the patient and improves the range of applications of CCTA.
Breathing motion and high HRV have been a limitation of CCTA, mainly due to the artifacts it produces that reduce image quality and diagnostic accuracy (5). With advances in CT technology, particularly improved temporal resolution (140 ms for the GE Revolution 256 rows, 135 ms for the Toshiba Aquilion ONE 320 rows (Toshiba America Medical Systems; Toshiba, Tustin, CA, USA), increased detector widths up to 16-cm-wide detectors represented by the Revolution (GE Healthcare) and the Aquilion ONE (Toshiba) represented by the widest 16-cm detector, the limitations of respiratory motion and high HRV on CCTA will probably improve.
Cardiac motion artifacts are the most common artifacts in the heart and include motion artifacts due to coronary artery motion in the X-Y axis as well as misalignment artifacts in the Z-axis (5,6). Increased detector width reduces the incidence of misalignment artifacts in the Z-axis. Jin et al. (21) found that compared to second-generation dual-source CT, third-generation dual-source CT scanner racks were faster, had increased Z-coverage, and had fewer acquisition steps, which resulted in a reduction in the incidence of misalignment artifacts during CCTA. With the increase of the detector width to 16 cm, the entire heart can be covered completely without moving the scanning bed, and the Z-axis time resolution is uniformly distributed, avoiding the occurrence of Z-axis misalignment artifacts. Therefore, current research is focused on how to reduce or avoid coronary motion artifacts in the X-Y axis.
Motion artifacts in the X-Y axis are generated by the heart, respiration, and overall motion of the patient (5,6). Patient movement can be avoided during the examination by instructing the patient before the examination to remain still during the examination and by tethering the patient with an immobilization strap on the examination bed. For respiratory movements, traditionally, patients are asked to hold their breath during the scan; however, due to a variety of factors, patients may be unable to hold their breath, limiting their CCTA examination. Under normal respiratory conditions, the respiratory rate is 12–20 breaths per minute, the range of diaphragmatic movement is 1.6 to 4.4 cm, and the speed of diaphragmatic movement is roughly 6.4–29.3 mm/s (22). Meanwhile, coronary artery velocities induced by the heartbeat were 22.4–108.6 mm/s (23). The former is much lower than the latter, a factor inherent in the patient. Meanwhile, the development of CT scanners, especially the improvement of temporal resolution (140 ms for 256 rows CT, 66 ms for third-generation dual-source CT (24), has improved the temporal resolution in the X-Y axis, which allows effective suppression of motion artifacts in coronary arteries, including motion artifacts induced by cardiac motion and respiratory motion. Therefore, artifacts caused by respiration are negligible based on inherent patient factors and improved temporal resolution of the CT scanner.
Kang et al. (10) utilized a 320-detector CT (with a temporal resolution of 135 ms) to achieve free-breathing CCTA under low HR (59±6.7 bpm) conditions. Liu et al. (12) utilized 256-row CT (140 ms temporal resolution) under different HR conditions, and the free-breathing protocol showed no significant difference in image quality or diagnostic performance. In this study, 256-row CT (140 ms temporal resolution) was used for comparison with the breath-holding protocol (group A). There was no significant difference in the free-breathing protocol (group B), indicating that the image quality is independent of respiratory motion, which further validates that the motion artifacts induced by respiration are negligible with the improved temporal resolution.
Importantly, the free-breathing protocol reduced the radiation dose to the patients, although the radiation dose to the CCTA patients increased with increasing HRV. The reason for this is that the percentage of the number of patients with a HR of 65–80 bpm in the free-breathing protocol [217/535 (40.5%)] was significantly lower than the percentage of the number of patients with a HR of 65–80 bpm in the breath-holding protocol [188/400 (47%)], and there was a statistically significant difference between the comparisons (P<0.001), which resulted in the radiation dose in the free protocol of the present study being significantly lower than that in the breath-holding protocol. Since different phase windows were set according to the patients’ HR in this study, the phase windows for HR <65, 65–80, and >80 bpm were set to 65–80%, 30–80%, and 35–50%, respectively, and the radiation dose to patients with a HR range of 65–80 bpm was significantly higher than that for HR <65 and >80 bpm. However, this was not the focus of this study because the radiation dose to the patient is affected by a number of factors such as tube voltage, patient weight, and scanning protocol.
However, 400 patients out of 502 who underwent breath-holding protocols were assessed to be in a respiratory resting state, and 322 out of 535 who underwent free-breathing protocols were assessed to be respiratory resting, so there are limitations in assessing free-breathing movement artifacts compared to data from respiratory resting patients in breath-holding protocols, as the free-breathing state consists of both respiratory movement and respiratory resting states. Therefore, the assessment of free-breathing-induced respiratory motion artifacts should consider the respiratory state factor, which is our next research direction. In addition, importantly, coronary motion artifacts are one of the important factors for high HR and HRV in addition to being associated with respiratory motion.
Cardiac motion produces artifacts in the X-Y axis caused mainly by high HR and high HRV. High HRs result in coronary motion that exceeds the acquisition rate, namely, insufficient temporal resolution, which is beyond the scope of this study. The effect of high HRV on image quality is related to the scanning mode, and the two main modes of CCTA scanning are retrospective electrocardiographic-gated spiral scanning and prospective ECG-triggered axial scanning. The guidelines strongly recommend the use of prospective ECG-triggered axial scanning modes as far as possible when the machine’s performance permits, as the latter can substantially reduce the radiation dose to the patient (9). For retrospective cardiac gating, high HRV is not associated with image quality because retrospective cardiac gating uses full-phase scanning at the expense of a high radiation dose. For the prospective ECG-triggered axial scanning mode, the scan is triggered at one phase of a cardiac cycle, with end-systole and mid-late diastole, the lowest cardiac motility, generally being the best phases.
High HRV leads to coronary motion artifacts associated with misconfiguration of the cardiac phase (6). For example, due to the sudden change in HR, the end-diastolic phase, which is supposed to be selected as the scanning phase because of the lowest motion, becomes early systolic, increasing motion artifacts because the myocardium is in a state of rapid motion during early systole. Therefore, for scanning protocols with fixed narrow phase (e.g., 65–80%) selection, high HRV will inevitably produce motion artifacts. However, for scanning schemes with wide phase (e.g., 30–80%, at the cost of increased radiation dose) selection, the effect of high HRV on motion artifacts will be substantially reduced, partly due to the wide range of phase selection and the low probability of complete mismatch to the 80–30% with high motion. Meanwhile, the application of software, especially second-generation motion correction technology, can select the phase with the smallest motion artifacts inside the wide phase for reconstruction, so the artifacts generated by the partial mismatch due to HRV will be greatly reduced. Chen et al. (15) found no statistically significant difference in subjective image quality between patients with high HRV (10–84 bpm) compared to those with low HRV (HRV <10 bpm), which can be explained by the fact that the CT scanner automatically widens the acquisition window in patients with high HRV (at the cost of an increased radiation dose), as well as by the use of a combination of motion correction techniques. We chose a 30–80% phase for patients with high HRV. The study showed that, in a within-group comparison, image quality decreased with increasing HRV. Still, there was no statistically significant difference, suggesting that the use of 30–80% phase for patients with high HRV, in combination with the second-generation motion correction technique, reduces the incidence of alignment errors, and that the quality of the image is independent of HRV.
Limitations
There are several limitations to this study. (I) The effect of free breathing on image quality in patients with high HR and tardive dyskinesia was not investigated and needs to be studied next. (II) The effect of the second generation of motion correction techniques on image motion correction needs to be further investigated. (III) This study is a single-center study and requires further validation with multiple centers, multiple machine models, and large sample sizes.
Conclusions
Based on the combined use of a 16 cm wide-body detector, 140 ms temporal resolution, and second-generation motion correction technology, it is possible to realize free-breathing CCTA examinations under different HRV conditions without loss of image quality, and the image quality is independent of HRV and respiration, which improves the range of CCTA applications.
Acknowledgments
We would like to express our gratitude to Dr. Shuai Zhang and Dr. Aiyun Sun from the GE medical team for the support of this research.
Footnote
Reporting Checklist: The authors have completed the STROBE reporting checklist. Available at https://qims.amegroups.com/article/view/10.21037/qims-24-1253/rc
Funding: None.
Conflicts of Interest: All authors have completed the ICMJE uniform disclosure form (available at https://qims.amegroups.com/article/view/10.21037/qims-24-1253/coif). The authors have no conflicts of interest to declare.
Ethical Statement: The authors are accountable for all aspects of the work in ensuring that questions related to the accuracy or integrity of any part of the work are appropriately investigated and resolved. The study was conducted in accordance with the Declaration of Helsinki (as revised in 2013). The study was approved by the Ethics Committee of Affiliated BenQ Hospital of Nanjing Medical University (No. 2022-KL002) and all patients provided written informed consent before participating in the study.
Open Access Statement: This is an Open Access article distributed in accordance with the Creative Commons Attribution-NonCommercial-NoDerivs 4.0 International License (CC BY-NC-ND 4.0), which permits the non-commercial replication and distribution of the article with the strict proviso that no changes or edits are made and the original work is properly cited (including links to both the formal publication through the relevant DOI and the license). See: https://creativecommons.org/licenses/by-nc-nd/4.0/.
References
- Knuuti J, Wijns W, Saraste A, Capodanno D, Barbato E, Funck-Brentano C, et al. 2019 ESC Guidelines for the diagnosis and management of chronic coronary syndromes. Eur Heart J 2020;41:407-77. [Crossref] [PubMed]
- Collet JP, Thiele H, Barbato E, Barthélémy O, Bauersachs J, Bhatt DL, et al. 2020 ESC Guidelines for the management of acute coronary syndromes in patients presenting without persistent ST-segment elevation. Eur Heart J 2021;42:1289-367. [Crossref] [PubMed]
- Gulati M, Levy PD, Mukherjee D, Amsterdam E, Bhatt DL, Birtcher KK, et al. 2021 AHA/ACC/ASE/CHEST/SAEM/SCCT/SCMR Guideline for the Evaluation and Diagnosis of Chest Pain: A Report of the American College of Cardiology/American Heart Association Joint Committee on Clinical Practice Guidelines. Circulation 2021;144:e368-454. [Crossref] [PubMed]
- Moss AJ, Williams MC, Newby DE, Nicol ED. The Updated NICE Guidelines: Cardiac CT as the First-Line Test for Coronary Artery Disease. Curr Cardiovasc Imaging Rep 2017;10:15. [Crossref] [PubMed]
- Kalisz K, Buethe J, Saboo SS, Abbara S, Halliburton S, Rajiah P. Artifacts at Cardiac CT: Physics and Solutions. Radiographics 2016;36:2064-83. [Crossref] [PubMed]
- Ghekiere O, Salgado R, Buls N, Leiner T, Mancini I, Vanhoenacker P, Dendale P, Nchimi A. Image quality in coronary CT angiography: challenges and technical solutions. Br J Radiol 2017;90:20160567. [Crossref] [PubMed]
- Maroules CD, Rybicki FJ, Ghoshhajra BB, Batlle JC, Branch K, Chinnaiyan K, Hamilton-Craig C, Hoffmann U, Litt H, Meyersohn N, Shaw LJ, Villines TC, Cury RC. 2022 use of coronary computed tomographic angiography for patients presenting with acute chest pain to the emergency department: An expert consensus document of the Society of cardiovascular computed tomography (SCCT): Endorsed by the American College of Radiology (ACR) and North American Society for cardiovascular Imaging (NASCI). J Cardiovasc Comput Tomogr 2023;17:146-63. [Crossref] [PubMed]
- Abbara S, Arbab-Zadeh A, Callister TQ, Desai MY, Mamuya W, Thomson L, Weigold WG. SCCT guidelines for performance of coronary computed tomographic angiography: a report of the Society of Cardiovascular Computed Tomography Guidelines Committee. J Cardiovasc Comput Tomogr 2009;3:190-204. [Crossref] [PubMed]
- Abbara S, Blanke P, Maroules CD, Cheezum M, Choi AD, Han BK, Marwan M, Naoum C, Norgaard BL, Rubinshtein R, Schoenhagen P, Villines T, Leipsic J. SCCT guidelines for the performance and acquisition of coronary computed tomographic angiography: A report of the society of Cardiovascular Computed Tomography Guidelines Committee: Endorsed by the North American Society for Cardiovascular Imaging (NASCI). J Cardiovasc Comput Tomogr 2016;10:435-49. [Crossref] [PubMed]
- Kang EJ, Lee J, Lee KN, Kown H, Ha DH, Kim RB. An initial randomised study assessing free-breathing CCTA using 320-detector CT. Eur Radiol 2013;23:1199-209. [Crossref] [PubMed]
- Bischoff B, Meinel FG, Del Prete A, Reiser MF, Becker HC. High-pitch coronary CT angiography in dual-source CT during free breathing vs. breath holding in patients with low heart rates. Eur J Radiol 2013;82:2217-21. [Crossref] [PubMed]
- Liu Z, Sun Y, Zhang Z, Chen L, Hong N. Feasibility of Free-breathing CCTA using 256-MDCT. Medicine (Baltimore) 2016;95:e4096. [Crossref] [PubMed]
- Shuai T, Deng L, Pan Y, Li W, Liao K, Li J, Peng L, Li Z. Free-breathing coronary CT angiography using 16-cm wide-detector for challenging patients: comparison with invasive coronary angiography. Clin Radiol 2018;73:986.e1-6. [Crossref] [PubMed]
- Halliburton SS, Abbara S, Chen MY, Gentry R, Mahesh M, Raff GL, Shaw LJ, Hausleiter JSociety of Cardiovascular Computed Tomography. SCCT guidelines on radiation dose and dose-optimization strategies in cardiovascular CT. J Cardiovasc Comput Tomogr 2011;5:198-224. [Crossref] [PubMed]
- Chen Y, Wei D, Li D, Liu Z, Hu Z, Li M, Jia Y, Yu Y, Han D, Ren R, Yu N, He T. The Value of 16-cm Wide-Detector Computed Tomography in Coronary Computed Tomography Angiography for Patients With High Heart Rate Variability. J Comput Assist Tomogr 2018;42:906-11. [Crossref] [PubMed]
- Ajlan AM, Binzaqr S, Jadkarim DA, Jamjoom LG, Leipsic J. High-pitch Helical Dual-source Computed Tomographic Pulmonary Angiography: Comparing Image Quality in Inspiratory Breath-hold and During Free Breathing. J Thorac Imaging 2016;31:56-62. [Crossref] [PubMed]
- Leipsic J, Abbara S, Achenbach S, Cury R, Earls JP, Mancini GJ, Nieman K, Pontone G, Raff GL. SCCT guidelines for the interpretation and reporting of coronary CT angiography: a report of the Society of Cardiovascular Computed Tomography Guidelines Committee. J Cardiovasc Comput Tomogr 2014;8:342-58. [Crossref] [PubMed]
- Muenzel D, Noel PB, Dorn F, Dobritz M, Rummeny EJ, Huber A. Step and shoot coronary CT angiography using 256-slice CT: effect of heart rate and heart rate variability on image quality. Eur Radiol 2011;21:2277-84. [Crossref] [PubMed]
- Liu Z, Zhang Z, Hong N, Chen L, Cao C, Liu J, Sun Y. Diagnostic performance of free-breathing coronary computed tomography angiography without heart rate control using 16-cm z-coverage CT with motion-correction algorithm. J Cardiovasc Comput Tomogr 2019;13:113-7. [Crossref] [PubMed]
- Hirshfeld JW Jr, Ferrari VA, Bengel FM, Bergersen L, Chambers CE, Einstein AJ, et al. 2018 ACC/HRS/NASCI/SCAI/SCCT Expert Consensus Document on Optimal Use of Ionizing Radiation in Cardiovascular Imaging-Best Practices for Safety and Effectiveness, Part 1: Radiation Physics and Radiation Biology: A Report of the American College of Cardiology Task Force on Expert Consensus Decision Pathways. J Am Coll Cardiol 2018;71:2811-28. [Crossref] [PubMed]
- Jin L, Zhou J, Gao Y, Zhao W, Li M, Wang Z. Reduction of cardiac motion artifact in step-and-shoot coronary CT angiography with third-generation as compared with second-generation dual-source CT scanners. Diagn Interv Radiol 2021;27:482-7. [Crossref] [PubMed]
- Boussuges A, Gole Y, Blanc P. Diaphragmatic motion studied by m-mode ultrasonography: methods, reproducibility, and normal values. Chest 2009;135:391-400. [Crossref] [PubMed]
- Mao S, Lu B, Oudiz RJ, Bakhsheshi H, Liu SC, Budoff MJ. Coronary artery motion in electron beam tomography. J Comput Assist Tomogr 2000;24:253-8. [Crossref] [PubMed]
- Miller RJH, Eisenberg E, Friedman J, Cheng V, Hayes S, Tamarappoo B, Thomson L, Berman DS. Impact of heart rate on coronary computed tomographic angiography interpretability with a third-generation dual-source scanner. Int J Cardiol 2019;295:42-7. [Crossref] [PubMed]