Spectral computed tomography parameters for predicting vessels encapsulating tumor clusters (VETC) pattern in hepatocellular carcinoma: a pilot study
Introduction
Hepatocellular carcinoma (HCC) constitutes 75–85% of primary liver cancer cases (1) and ranks as the third most prevalent malignant tumor globally (2). Despite the application of surgical resection, the primary treatment modality for HCC, the 5-year rates of metastasis and recurrence remain high (2,3). Vessels encapsulating tumor clusters (VETC), characterized by endothelial cells surrounding tumor cell nests, are a prevalent and recently identified microvascular pattern in HCC (4,5). Unlike metastasis mediated by the conventional epithelial-mesenchymal transition (EMT), VETC exhibits enhanced metastatic potential, leading to significantly reduced overall and recurrence-free survival (RFS) rates and thus poorer outcomes in patients with HCC (6). However, VETC detection primarily relies on postoperative immunohistochemical examinations, which are constrained by invasive tissue acquisition requirements. These inherent drawbacks—including limitations in pathology sampling and delayed diagnostic availability—frequently compromise assessment completeness. Consequently, there is an urgent need to develop a preoperative, noninvasive, and precise approach for predicting VETC patterns in HCC, which would aid in personalized therapeutic strategies and prognostic evaluation prior to surgical intervention. Spectral computed tomography (CT) offers multiparameter functional imaging capabilities, allowing for the noninvasive and quantitative assessment of tumor pathology (7,8). The relationship between multiparameter spectral CT and VETC patterns has not been extensively investigated. This study thus aimed to assess the value of multiparameter spectral CT in predicting VETC patterns in HCC and develop a novel preoperative noninvasive prediction method integrating multiparametric spectral CT biomarkers. We present this article in accordance with the STARD reporting checklist (available at https://qims.amegroups.com/article/view/10.21037/qims-24-2077/rc).
Methods
Study design and patient population
This study was conducted in accordance with the Declaration of Helsinki (as revised in 2013) and received ethical approval from the Ethics Committees of Weihai Central Hospital Affiliated to Qingdao University (approval No. 2022-121-01). The requirement for individual consent was waived due to the retrospective nature of the analysis. This retrospective cohort study consecutively enrolled 50 patients with HCC who were admitted to Weihai Central Hospital Affiliated to Qingdao University between May 2020 and May 2023. The inclusion criteria were as follows: (I) primary HCC confirmed by postoperative pathology and CD34 immunohistochemistry; (II) liver contrast-enhanced spectral CT conducted ≤7 days before resection; and (III) complete serum biomarkers tests [alpha-fetoprotein (AFP), protein-induced by vitamin K absence or antagonist-II (PIVKA-II), carcinoembryonic antigen (CEA)] completed ≤7 days before resection. Meanwhile, the exclusion criteria were as follows: (I) a history of HCC recurrence; (II) administration of preoperative anticancer treatment; (III) significant respiratory motion artifacts in the images; and (IV) biopsy-proven diagnosis. VETC(+) was defined according to a previous publication (9) as CD34+ vascular encapsulation occupying ≥5% of the tumor area as confirmed by CD34 immunostaining; otherwise, a VETC(−) status was confirmed. The flow diagram depicting the patient selection process is shown in Figure 1.
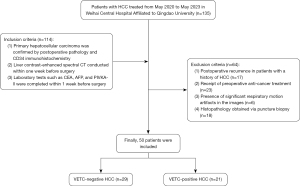
CT image acquisition
All imaging examinations were conducted using a dual-layer detector spectral CT device (IQon, Philips Healthcare, Best, the Netherlands). Scanning sequences included unenhanced phase (UP), arterial phase (AP), portal phase (PP), and equilibrium phase (EP). The technical parameters were as follows: a tube voltage of 120 kVp, a tube current automatically adjusted by tube current modulation (TCM), a detector collimation of 0.625 mm × 64 mm, a rotation speed of 0.5 s, and a pitch factor of 1.234. For contrast-enhanced imaging, ioversol (320 mgI/mL) was administered intravenously via an antecubital vein using a dual-barrel high-pressure syringe at a dose of 1.5 mL/kg body weight and a flow rate of 3.0 mL/s. SmartPrep dynamic monitoring technology was used to synchronize scanning initiation. The AP scan was automatically triggered once the region of interest (ROI) reached a predefined threshold of 100 Hounsfield units (HU). Subsequent phases were acquired with fixed delays: the PP was triggered at 40 s after AP initiation and the EP at 120 s after AP initiation.
Image processing and analysis
Spectral-based image (SBI) data were uploaded to the postprocessing software (Philips IntelliSpace Portal, Philips Healthcare; version 9.0) on a dedicated workstation for spectral CT, and the morphological features of the HCC were evaluated on 40-keV virtual monoenergetic images (VMIs). Uneven or nonsignificant enhancement of the tumor in the AP can affect the accuracy of the selected ROI. Therefore, quantitative spectral parameters, including iodine concentration (IC), normalized IC (NIC), effective atomic number (Zeff), and energy spectrum curve slope (λ), in the PP and EP were analyzed. NIC was calculated as the ratio of lesion IC to aortic IC (NIC = IClesion/ICaorta) at the same section. λ was defined as the difference in CT numbers at 40 and 90 keV, divided by the energy difference: λ = [CT number (40 keV) − CT number (90 keV)]/(90−40) keV. The 40-keV energy level was selected as it typically shows the greatest enhancement; for the higher energy level, values between 70 and 150 keV are commonly reported. The selection of 90 keV as the upper energy threshold was based on preliminary observations that CT values of lesions show minimal change at energy levels above 90 keV. Qualitative and quantitative assessments of the lesions were performed by two radiologists with more than 8 years of experience in abdominal radiology. Following previous literature (10) and Liver Imaging Reporting and Data System (LI-RADS) v.2018 (11), we selected the following key features for assessment: (I) tumor size (maximum radial length of the main lesion in axial position); (II) intratumor vascularity (persistent vascular within the tumor in AP); (III) nonrim AP hyperenhancement; (IV) nonperipheral PP washout; (V) a well-defined capsule (most or all of the tumor clearly demarcated from the surrounding hepatic parenchyma, with circumferential enhancement visible in the PP or the EP); (VI) a nonsmooth tumor margin (bud-like projections protruding from the tumor margins or local infiltration); (VII) intratumor necrosis (at the level of the largest radial dimension of the tumor in the axial plane and ≥20% of the nonenhanced area across phases); and (VIII) intratumor AP hypovascular component (IAPHC; ≥20% hypoenhanced viable tumor during the AP).
Multiple ROIs were selected from the same lesion, and the final values were averaged across all measurements, with necrotic, calcified, and vascular areas being excluded.
Follow-up after surgery
Patients underwent regular follow-up visits at 2- to 3-month intervals after surgery. RFS was as defined as the interval from surgical resection to radiologically confirmed tumor recurrence. Recurrence was confirmed through at least two radiological examinations [CT, magnetic resonance imaging (MRI), or ultrasound]. Follow-up was terminated upon confirmed local recurrence or distant metastasis, with the date of recurrence or metastasis recorded as the termination point for RFS; for nonrecurrent cases, the end of the follow-up period was June 1, 2024.
Statistical analysis
All statistical analyses were performed using SPSS 23.0 (IBM Corp., Armonk, NY, USA), MedCalc version 22.0 (MedCalc Software Ltd., Ostend, Belgium), and GraphPad Prism version 9.0 (Dotmatics, Boston, MA, USA). Quantitative data with a normal distribution are presented as the mean ± standard deviation (SD), and the independent samples t-test was employed to compare the data between the two groups. Clinical data and qualitative parameters of spectral CT for the two groups were compared using the Chi-squared test. Variables with statistical significance underwent multivariate logistic regression so that the independent VETC predictors could be identified. Finally, the receiver operating characteristic (ROC) curve was used to evaluate the predictive performance of each single parameter that had a significant difference and the combined model. The related 95% confidence interval (CI), sensitivity, and specificity were evaluated with area under the curve (AUC) comparisons performed with the DeLong test. Statistical significance was defined as a P value ≤0.05. Survival curves were generated with the Kaplan-Meier method and compared via the log-rank test.
Results
Clinical characteristics
The VETC(+) (n=21) and VETC(−) (n=29) cohorts demonstrated comparable demographic profiles, with mean ages of 61.43±7.78 and 60.69±9.39 years, respectively (P>0.05). The AFP levels were significantly elevated in VETC(+) patients (P<0.05) (Table 1), while CEA and PIVKA-II showed no intergroup differences (P>0.05) (Table 1).
Table 1
Parameters | VETC(+) (n=21) | VETC(−) (n=29) | χ2/t | P |
---|---|---|---|---|
Sex (male) | 15 | 24 | χ2=0.371 | 0.543 |
Age (years) | 61.43±7.78 | 60.69±9.39 | t=0.304 | 0.763 |
AFP ≥400 ng/mL | 9 | 4 | χ2=5.348 | 0.021 |
CEA ≥5 ng/mL | 1 | 12 | χ2=1.729 | 0.189 |
PIVKA-II ≥500 mAU/mL | 7 | 5 | χ2=0.335 | 0.563 |
Data are presented as number or as the mean ± SD. AFP, alpha-fetoprotein; CEA, carcinoembryonic antigen; PIVKA-II, protein induced by vitamin K absence or antagonist-II; SD, standard deviation; VETC, vessels encapsulating tumor clusters.
Qualitative spectral parameters in predicting VETC
As compared to VETC(−) tumors, VETC(+) HCC tumors demonstrated a significantly higher prevalence of IAPHC (P<0.05) (Table 2). However, no significant intergroup differences were observed regarding tumor size, nonrim AP hyperenhancement, nonperipheral PP washout, nonsmooth tumor margin, well-defined capsule, intratumor necrosis, or intratumor vascularity (all P values >0.05) (Table 2). Cases of HCC with VETC(+) and VETC(−) are shown in Figure 2.
Table 2
Parameters | VETC(+) (n=21) | VETC(−) (n=29) | χ2 | P |
---|---|---|---|---|
Tumor size (>5 cm) | 10 | 8 | 2.122 | 0.145 |
Intratumor vascularity | 11 | 8 | 3.178 | 0.075 |
Nonrim AP hyperenhancement | 17 | 23 | 0.021 | >0.99 |
Nonperipheral PP washout | 9 | 12 | 0.011 | 0.917 |
Well-defined capsule | 7 | 15 | 1.672 | 0.196 |
Nonsmooth tumor margin | 12 | 14 | 0.384 | 0.536 |
Intratumor necrosis (≥20%) | 14 | 12 | 3.120 | 0.077 |
IAPHC (≥20%) | 12 | 9 | 5.889 | 0.015 |
Data are presented as number. AP, arterial phase; CT, computed tomography; IAPHC, intratumor arterial phase hypovascular component; PP, portal phase; VETC, vessels encapsulating tumor clusters.
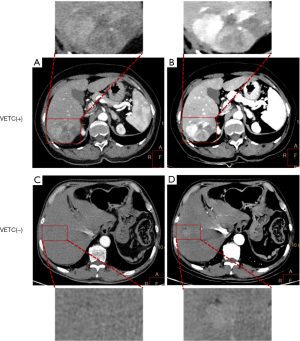
Quantitative spectral parameters in predicting VETC
The results showed that patients with VETC(+) HCC exhibited elevated spectral parameters in the PP (IC: P=0.012; Zeff: P=0.031; λ: P=0.034) and in the EP (IC: P=0.002; Zeff: P=0.023; λ; P=0.028) (Table 3 and Figure 3). However, there were no significant differences in NIC between the two groups in either the PP or EP (P>0.05) (Table 3 and Figure 3). Cases of HCC with VETC(+) and VETC(−) are shown in Figure 4 and Figure 5, respectively.
Table 3
Parameters | VETC(+) (n=21) | VETC(−) (n=29) | t | P |
---|---|---|---|---|
IC-PP | 1.58±0.51 | 1.20±0.50 | 2.625 | 0.012 |
NIC-PP | 0.42±0.09 | 0.35±0.13 | 1.966 | 0.055 |
IC-EP | 1.16±0.32 | 0.84±0.34 | 3.341 | 0.002 |
NIC-EP | 0.48±0.11 | 0.40±0.16 | 1.890 | 0.065 |
Zeff-PP | 8.17±0.24 | 8.01±0.28 | 2.225 | 0.031 |
Zeff-EP | 7.97±0.17 | 7.83±0.22 | 2.352 | 0.023 |
λ-PP | 2.22±0.69 | 1.77±0.75 | 2.187 | 0.034 |
λ-EP | 1.67±0.46 | 1.34±0.55 | 2.259 | 0.028 |
Data are presented as mean ± SD. CT, computed tomography; IC-EP, iodine concentration in the equilibrium phase; IC-PP, iodine concentration in the portal phase; λ-EP, energy spectrum curve slope of the equilibrium phase; λ-PP, energy spectrum curve slope of the portal phase; NIC-EP, normalized iodine concentration in the equilibrium phase; NIC-PP, normalized iodine concentration in the portal phase; SD, standard deviation; VETC, vessels encapsulating tumor clusters; Zeff-EP, effective atomic number in the equilibrium phase; Zeff-PP, effective atomic number in the portal phase.
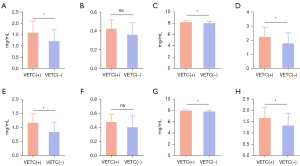
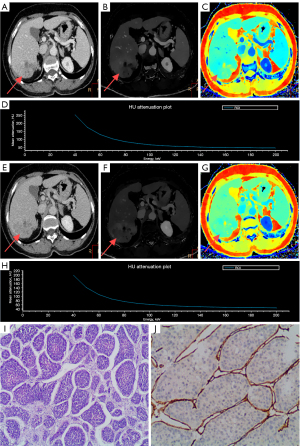
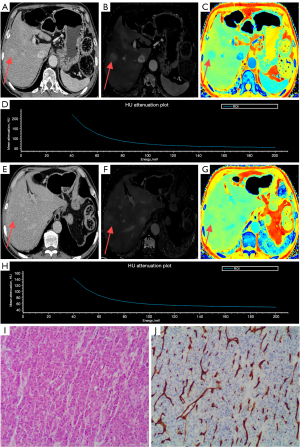
Risk factors of predicting VETC and ROC analysis
Multivariate logistic regression identified two independent predictors of VETC positivity: IAPHC and IC-EP (Table 4). A combined model was constructed, and the ROC curve showed that the AUC of IAPHC, IC-EP, and the combined model were 0.672, 0.766, and 0.810, respectively (Table 5, Table S1, Figure 6, and Figure S1). The AUC of the combined model for predicting VETC(+) HCC was greater than that of IAPHC and IC-EP. The difference in AUC value between the combined model and IAPHC was statistically significant (Z=2.34; P=0.02), while the difference between the combined model and IC-EP was not (Z=0.98; P=0.33).
Table 4
Variables | Regression coefficient | OR (95% CI) | P |
---|---|---|---|
IAPHC (≥20%) | 1.423 | 4.149 (1.133–15.192) | 0.032 |
IC-EP | 2.543 | 12.724 (1.594–101.555) | 0.016 |
CI, confidence interval; HCC, hepatocellular carcinoma; IC-EP, iodine concentration in the equilibrium phase; IAPHC, intratumor arterial phase hypovascular component; OR, odds ratio; VETC, vessels encapsulating tumor clusters.
Table 5
Variables | AUC (95% CI) | Sensitivity (%) | Specificity (%) |
---|---|---|---|
IAPHC (≥20%) | 0.672 (0.524–0.798) | 61.90 | 72.41 |
IC-EP | 0.766 (0.625–0.874) | 80.95 | 68.97 |
Combined model | 0.810 (0.675–0.907) | 66.67 | 89.66 |
AUC, area under the curve; CI, confidence interval; HCC, hepatocellular carcinoma; IC-EP, iodine concentration in the equilibrium phase; IAPHC, intratumor arterial phase hypovascular component; VETC, vessels encapsulating tumor clusters.
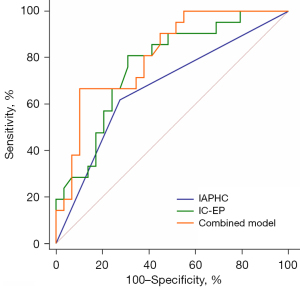
Prognostic evaluation
Recurrence was detected in 15 of 50 patients (30%) during follow-up, and the RFS rates had significantly different between groups. Patients in the VETC(+) group had significantly poor RFS rates than did those in the VETC(−) group at 1-year (75.2% vs. 86.2%), 2-year (44.5% vs. 79.6%), and 3-year follow-up (44.5% vs. 79.6%). The Kaplan-Meier curves for RFS were significantly different between the two groups (log-rank χ2=5.31; P=0.02) (Figure 7).
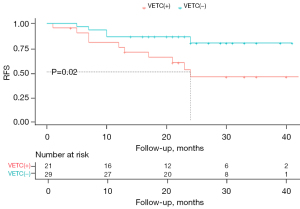
Discussion
VETC is a recently discovered HCC metastatic pattern, and the research related to its radiology-based preoperative prediction remains in the exploratory stage. In this study, we employed spectral CT multiparameter features to predict the VETC pattern for noninvasive VETC detection, which may offer valuable guidance for treatment planning and prognosis assessment.
In this study, AP 40-keV VMI was used to visualize intratumor hypoenhanced areas, and the results showed that IAPHC was significantly correlated with VETC(+) HCC. These findings are consistent with previous work on contrast-enhanced MRI. For instance, Fan et al. (12) identified AP tumors with diffuse and heterogeneous enhancement as independent predictive factors for VETC(+) HCC; Chen et al. (13) noted a significant correlation between tumor heterogeneous enhancement patterns (uneven enhancement with septa and irregular ring-like structures) and VETC(+) HCC. In our study, we found inhomogeneous and heterogenous intratumoral enhancement. We speculate that this may be related to the unique vascular structure of VETC. VETC is composed of functional blood vessels with blood perfusion, and these functional sinusoidal vessels, arranged in cobweb-like networks, can compress the adjacent vasculature, leading to insufficient local blood supply (4); moreover, this cobweb-like vascular structure may also impede the flow of blood, slowing down the inflow and outflow of the iodine contrast agent, which manifests as a hypointensified area inside the tumor in the AP. The above speculation was also consistent with the Lan et al. (14), who found that ultrasonography of VETC(+) HCC first revealed circumferential hyperenhancement around the periphery of the tumor and then filling in the center in the form of stripes and fissures, with a prolonged filling time per unit diameter compared with that of VETC(−) HCC. In their study, Rhee et al. (15) reported that 50.8% of macrotrabecular-massive (MTM)-HCC specimens exhibited VETC positivity, with a correlation between IAPHC and MTM-HCC. Approximately 77% of MTM-HCCs showed intra-arterial stage tumors or diffuse areas of hypoenhancement with peripheral or speckled areas of hyperenhancement. This radiopathological correlation was attributed to two distinct mechanisms: intratumor necrosis and abundant fibrotic mesenchyme within the tumor (which is enriched with VETC patterns in MTM-HCC). Therefore, we speculate that the abundant fibrotic mesenchyme within the tumor is a key determinant of IAPHC manifestation in VETC(+) HCC.
Our quantitative analysis demonstrated significantly elevated IC-PP and IC-EP in the VETC(+) HCC group as compared to the VETC(−) HCC groups. While angiogenesis is central to tumor growth and metastasis, quantitative analysis of angiogenesis in vivo remains challenging. Microvessel density (MVD) within tumors has shown promise as a biomarker. High MVD within tumors may be associated with angiogenesis and tumor invasiveness (16). Huang et al. (17) found increased intratumoral MVD in VETC(+) HCC, whereas in nontumor areas, MVD was not significantly different between the VETC(+) and VETC(−) groups, suggesting that VETC(+) HCC has increased vascularity within the tumor and is highly invasive. However, another study found that hypovascularity in VETC-HCC may arise from sinusoidal-pattern HCC due to low MVD (18). Similarly, Li et al. found a positive correlation between IC and NIC measured by spectral CT with MVD among patients with lung cancer (19); moreover, venous-phase IC had the strongest correlation with MVD and was also associated lung cancer pathological classification, tumor differentiation, tumor size, and intratumoral necrosis status. In our HCC study, we also found statistical differences in the IC in the EP between the two groups. Wang et al. examined the relationship between tumor angiogenesis and dynamic enhanced MRI features in HCC (20). Their analysis revealed that the MVD in the group with PP enhancement was higher than that of the group without enhancement. Meanwhile, delayed-phase ring-enhancement patterns correlated with increased MVD as compared the nonring enhancement patterns. High-MVD HCC maintained persistent enhancement of tumor in the PP and delayed phase. Despite the different enhancement mechanisms of MRI and CT, both may be related to tumor angiogenesis (20). Our results are similar to those of Li et al. (19) and Wang et al. (20), and our IC findings may be explained by the following three pathophysiological mechanisms. First, in VETC(+) HCC, elevated MVD increases intratumoral vascularity and blood perfusion, augmenting intratumoral iodine contrast agent content and IC. Second, the unique reticular vascular pattern of VETC may delay the outflow of contrast agent, resulting in iodine contrast agent retention and elevation of IC during the PP and EP (4). Third, the abundant fibrotic stroma within the tumor tissue manifests as delayed enhancement, and fibrotic components during the EP increase the iodine content within the lesion, thereby increasing IC (15). This may explain why the IC in the PP and EP was higher in the VETC(+) group than in the VETC(−) group in our study.
The results regarding IC and NIC are of particular interest and initially difficult to explain, as they deviate from the conclusions of many previous studies. Specifically, in research on gastric and lung cancers using spectral CT, NIC has often demonstrated superior diagnostic and predictive value as compared to IC (21,22). We believe this discrepancy may be attributed to two factors: patient characteristics and sample size limitations. Most patients included in this study had coexisting liver cirrhosis, and some were in the decompensated stage. The hemodynamic disturbances caused by complications of liver cirrhosis or concurrent heart failure could lead to differences between the IC within the lesion and the IC of the abdominal aorta (23). Additionally, the liver’s dual blood supply and potential presence of arteriovenous shunts in some HCC cases might have also contributed to this (24). Additionally, our relatively small sample size might have influenced the overall findings, particularly given the inclusion of a significant proportion of cases with decompensated liver cirrhosis combined with HCC.
This study further integrated IAPHC and IC-EP to construct a combined prediction model. The results showed that compared to single risk factors, the combined model demonstrated a significantly superior ability to predict VETC(+) HCC. This suggests that the combined model has better preoperative diagnostic efficacy and can provide useful auxiliary indicators for clinical treatment selection. Histologic VETC has been reported to be associated with a poor prognosis in patients with HCC in a number of studies (25,26), with which our results are consistent. This may be related to the VETC pattern, in which tumor nests form at the primary site and then actively protrude from the tumor mass as a whole, floating within the vascular lumen of the tumor stroma. Through migration and invasion, tumor cells traverse the vascular wall. During circulation, endothelial cell encapsulation protects the cancer cells from hypoxia and immune attacks, thus preventing tumor cells from being killed during the metastatic process (12,27).
This study involved certain limitations that should be acknowledged. First, the single-center retrospective design might have introduced selection bias. Second, the sample size of this study is small. Future studies with larger cohorts are warranted to verify these preliminary findings.
Conclusions
IAPHC and IC-EP may have significant application value in predicting VETC(+) HCC. Additionally, the combination of these two factors demonstrated improved diagnostic performance compared to IAPHC or IC-EP alone and may be an effective approach for the preoperative, noninvasive, and quantitative prediction of the VETC pattern.
Acknowledgments
The authors would like to thank the Weihai Key Laboratory of Interventional Minimally Invasive Technology based on Radiology, the Weihai Clinical Research Center for Radiology, and the Shandong Provincial Key Medical and Health Laboratory of Iron Metabolism Clinical Research for their support of this study.
Footnote
Reporting Checklist: The authors have completed the STARD reporting checklist. Available at https://qims.amegroups.com/article/view/10.21037/qims-24-2077/rc
Funding: This work was supported by
Conflicts of Interest: All authors have completed the ICMJE uniform disclosure form (available at https://qims.amegroups.com/article/view/10.21037/qims-24-2077/coif). X.C. is an employee of Philips Healthcare, the manufacturer of the CT system used in this study. The other authors have no conflicts of interest to declare.
Ethical Statement: The authors are accountable for all aspects of the work by ensuring that questions related to the accuracy or integrity of any part of the work are appropriately investigated and resolved. This study was conducted in accordance with the Declaration of Helsinki (as revised in 2013) and was approved by the Ethics Committees of Weihai Central Hospital Affiliated to Qingdao University (approval No. 2022-121-01). The requirement for individual consent was waived due to the retrospective nature of the analysis.
Open Access Statement: This is an Open Access article distributed in accordance with the Creative Commons Attribution-NonCommercial-NoDerivs 4.0 International License (CC BY-NC-ND 4.0), which permits the non-commercial replication and distribution of the article with the strict proviso that no changes or edits are made and the original work is properly cited (including links to both the formal publication through the relevant DOI and the license). See: https://creativecommons.org/licenses/by-nc-nd/4.0/.
References
- Sung H, Ferlay J, Siegel RL, Laversanne M, Soerjomataram I, Jemal A, Bray F. Global Cancer Statistics 2020: GLOBOCAN Estimates of Incidence and Mortality Worldwide for 36 Cancers in 185 Countries. CA Cancer J Clin 2021;71:209-49. [Crossref] [PubMed]
- Sun Y, Wu L, Zhong Y, Zhou K, Hou Y, Wang Z, et al. Single-cell landscape of the ecosystem in early-relapse hepatocellular carcinoma. Cell 2021;184:404-421.e16. [Crossref] [PubMed]
- Hepatocellular carcinoma. Nat Rev Dis Primers 2021;7:7. [Crossref] [PubMed]
- Fang JH, Zhou HC, Zhang C, Shang LR, Zhang L, Xu J, Zheng L, Yuan Y, Guo RP, Jia WH, Yun JP, Chen MS, Zhang Y, Zhuang SM. A novel vascular pattern promotes metastasis of hepatocellular carcinoma in an epithelial-mesenchymal transition-independent manner. Hepatology 2015;62:452-65. [Crossref] [PubMed]
- Li Z, Song W, Zhang J, Li Q, Song Z, Ren X, Wen Y, Li X, Yao H, Gao Y, Tang Z. Identification of vessels encapsulating tumor clusters in solitary hepatocellular carcinoma via imaging biomarkers in preoperative contrast-enhanced magnetic resonance imaging. Quant Imaging Med Surg 2024;14:8586-600. [Crossref] [PubMed]
- Ding T, Xu J, Zhang Y, Guo RP, Wu WC, Zhang SD, Qian CN, Zheng L. Endothelium-coated tumor clusters are associated with poor prognosis and micrometastasis of hepatocellular carcinoma after resection. Cancer 2011;117:4878-89. [Crossref] [PubMed]
- Sauerbeck J, Adam G, Meyer M. Spectral CT in Oncology. Rofo 2023;195:21-9. [Crossref] [PubMed]
- So A, Nicolaou S. Spectral Computed Tomography: Fundamental Principles and Recent Developments. Korean J Radiol 2021;22:86-96. [Crossref] [PubMed]
- Zhong L, Long S, Pei Y, Liu W, Chen J, Bai Y, Luo Y, Zou B, Guo J, Li M, Li W. MRI Tomoelastography to Assess the Combined Status of Vessels Encapsulating Tumor Clusters and Microvascular Invasion in Hepatocellular Carcinoma. J Magn Reson Imaging 2024; Epub ahead of print. [Crossref]
- Xu X, Zhang HL, Liu QP, Sun SW, Zhang J, Zhu FP, Yang G, Yan X, Zhang YD, Liu XS. Radiomic analysis of contrast-enhanced CT predicts microvascular invasion and outcome in hepatocellular carcinoma. J Hepatol 2019;70:1133-44. [Crossref] [PubMed]
- Chernyak V, Fowler KJ, Kamaya A, Kielar AZ, Elsayes KM, Bashir MR, Kono Y, Do RK, Mitchell DG, Singal AG, Tang A, Sirlin CB. Liver Imaging Reporting and Data System (LI-RADS) Version 2018: Imaging of Hepatocellular Carcinoma in At-Risk Patients. Radiology 2018;289:816-30. [Crossref] [PubMed]
- Fan Y, Yu Y, Hu M, Wang X, Du M, Guo L, Hu C. Imaging features based on Gd-EOB-DTPA-enhanced MRI for predicting vessels encapsulating tumor clusters (VETC) in patients with hepatocellular carcinoma. Br J Radiol 2021;94:20200950. [Crossref] [PubMed]
- Chen FM, Du M, Qi X, Bian L, Wu D, Zhang SL, Wang J, Zhou Y, Zhu X. Nomogram Estimating Vessels Encapsulating Tumor Clusters in Hepatocellular Carcinoma From Preoperative Gadoxetate Disodium-Enhanced MRI. J Magn Reson Imaging 2023;57:1893-905. [Crossref] [PubMed]
- Lan CY, Ling B, Guo WW, Yin W, Zhong XG, Han YM, Dong XF. The relationship between vimentin protein expression in endothelial cells and contrast-enhanced ultrasound characters in VETC (+) hepatocellular carcinoma. Zhonghua Zhong Liu Za Zhi 2018;40:105-9. [Crossref] [PubMed]
- Rhee H, Cho ES, Nahm JH, Jang M, Chung YE, Baek SE, Lee S, Kim MJ, Park MS, Han DH, Choi JY, Park YN. Gadoxetic acid-enhanced MRI of macrotrabecular-massive hepatocellular carcinoma and its prognostic implications. J Hepatol 2021;74:109-21. [Crossref] [PubMed]
- Wada H, Nagano H, Yamamoto H, Yang Y, Kondo M, Ota H, Nakamura M, Yoshioka S, Kato H, Damdinsuren B, Tang D, Marubashi S, Miyamoto A, Takeda Y, Umeshita K, Nakamori S, Sakon M, Dono K, Wakasa K, Monden M. Expression pattern of angiogenic factors and prognosis after hepatic resection in hepatocellular carcinoma: importance of angiopoietin-2 and hypoxia-induced factor-1 alpha. Liver Int 2006;26:414-23. [Crossref] [PubMed]
- Huang CW, Lin SE, Huang SF, Yu MC, Tang JH, Tsai CN, Hsu HY. The Vessels That Encapsulate Tumor Clusters (VETC) Pattern Is a Poor Prognosis Factor in Patients with Hepatocellular Carcinoma: An Analysis of Microvessel Density. Cancers (Basel) 2022;14:5428. [Crossref] [PubMed]
- Rhee H, Park YN, Choi JY. Advances in Understanding Hepatocellular Carcinoma Vasculature: Implications for Diagnosis, Prognostication, and Treatment. Korean J Radiol 2024;25:887-901. [Crossref] [PubMed]
- Li Q, Li X, Li XY, Huo JW, Lv FJ, Luo TY. Spectral CT in Lung Cancer: Usefulness of Iodine Concentration for Evaluation of Tumor Angiogenesis and Prognosis. AJR Am J Roentgenol 2020;215:595-602. [Crossref] [PubMed]
- Wang B, Gao ZQ, Yan X. Correlative study of angiogenesis and dynamic contrast-enhanced magnetic resonance imaging features of hepatocellular carcinoma. Acta Radiol 2005;46:353-8. [Crossref] [PubMed]
- Mu R, Meng Z, Guo Z, Qin X, Huang G, Yang X, Jin H, Yang P, Zhang X, Zhu X. Dual-layer spectral detector computed tomography parameters can improve diagnostic efficiency of lung adenocarcinoma grading. Quant Imaging Med Surg 2022;12:4601-11. [Crossref] [PubMed]
- Mao LT, Chen WC, Lu JY, Zhang HL, Ye YS, Zhang Y, Liu B, Deng WW, Liu X. Quantitative parameters in novel spectral computed tomography: Assessment of Ki-67 expression in patients with gastric adenocarcinoma. World J Gastroenterol 2023;29:1602-13. [Crossref] [PubMed]
- Juanola A, Tiwari N, Solé C, Adebayo D, Wong F, Ginès P. Organ dysfunction and failure in liver disease. Liver Int 2025;45:e15622. [Crossref] [PubMed]
- Kim HC, Suk KT, Kim DJ, Yoon JH, Kim YS, Baik GH, Kim JB, Kim CH, Sung H, Choi JY, Han KH, Park SH. Transarterial chemoembolization in Barcelona Clinic Liver Cancer Stage 0/A hepatocellular carcinoma. World J Gastroenterol 2014;20:745-54. [Crossref] [PubMed]
- Matsuda K, Ueno A, Tsuzaki J, Kurebayashi Y, Masugi Y, Yamazaki K, Tamura M, Abe Y, Hasegawa Y, Kitago M, Jinzaki M, Sakamoto M. Vessels encapsulating tumor clusters contribute to the intratumor heterogeneity of HCC on Gd-EOB-DTPA-enhanced MRI. Hepatol Commun 2024;9:e0593. [Crossref] [PubMed]
- Xiong SP, Wang CH, Zhang MF, Yang X, Yun JP, Liu LL. A multi-parametric prognostic model based on clinicopathologic features: vessels encapsulating tumor clusters and hepatic plates predict overall survival in hepatocellular carcinoma patients. J Transl Med 2024;22:472. [Crossref] [PubMed]
- Renne SL, Woo HY, Allegra S, Rudini N, Yano H, Donadon M, Viganò L, Akiba J, Lee HS, Rhee H, Park YN, Roncalli M, Di Tommaso L. Vessels Encapsulating Tumor Clusters (VETC) Is a Powerful Predictor of Aggressive Hepatocellular Carcinoma. Hepatology 2020;71:183-95. [Crossref] [PubMed]