Magnetic resonance diffusion tensor imaging is superior to arterial spin labeling in detecting renal allograft fibrosis
Introduction
Renal transplantation (RT) in patients with end-stage renal disease is the most effective means of renal replacement therapy. Compared with dialysis, RT improves survival and quality of life in end-stage renal disease patients (1). However, a variety of complications after RT are important factors that cause graft dysfunction or even loss of function. Chronic graft rejection is one of the main causes of long-term graft function loss after RT (2-4). In its pathogenesis, antigen-dependent and antigen-independent factors play an important role in causing graft fibrosis. Due to the compensatory nature of the kidney and the lag of clinical features, the progression of fibrosis is often not detected early. The degree of fibrosis is often obtained clinically by renal biopsy and classified according to the Banff criteria (5). However, due to the limitation of the biopsy site, false negative results may occur (6). In addition, poor patient acceptance due to its invasive nature makes repeat biopsy difficult to perform.
In addition to providing morphological features, magnetic resonance imaging (MRI) can also well reflect the complications and functional information of RT (7-9). In addition, MRI has good repeatability, is not affected by the operator, and does not require the use of contrast media and ionizing radiation; it does not cause obvious damage to the function of the transplanted kidney, so it can be used in patients with renal insufficiency and measured repeatedly. For renal allograft fibrosis, MRI can reflect the degree of fibrosis through different sequences according to the different pathophysiological changes caused by fibrosis (10-14). Diffusion tensor imaging (DTI) can measure the diffusion of water molecules in all directions. The extent to which diffusion exhibits orientation preference was evaluated by fractional anisotropy (FA), ranging from 0 to 1. Based on this principle, DTI can well detect the changes of renal structure and microstructure in allograft kidney transplantation, such as renal tubular atrophy and interstitial fibrosis (14). Arterial spin labeling (ASL) is performed by selectively labelling the inflow of blood with a magnetization opposite to that of the tissue in the region of interest (ROI). Tissue perfusion can be measured based on the signal difference between labeled images (tag) and unlabeled images (control) without injection of exogenous contrast agent. However, progressive capillary loss during renal fibrosis leads to reduced blood flow and oxygen delivery to renal parenchymal tubular epithelial cells, thereby driving the progression of fibrosis (14,15). Few previous studies have compared the diagnostic performance of MRI sequences to discriminate the degree of fibrosis. Some researchers have compared the diagnostic efficacy of ASL and intravoxel incoherent motion (IVIM) with reduced field of view (FOV) for fibrosis (16). The aim of this study was to compare the diagnostic efficacy of ASL and DTI for fibrosis. We present this article in accordance with the STROBE reporting checklist (available at https://qims.amegroups.com/article/view/10.21037/qims-24-1023/rc).
Methods
Study population
The study was conducted in accordance with the Declaration of Helsinki (as revised in 2013) and approved by the Ethics Review Board of The First Affiliated Hospital of Soochow University on 27 October 2022, with the review number (2022) (No. 412). Informed consent was provided by all individual participants. For participants under 18, informed consent was provided by their legal guardians. Between 21 January 2022 and 31 May 2023, 145 renal transplant recipients who underwent renal allograft biopsy at the urology department of our hospital were recruited. All participants underwent DTI and ASL within 1 week before and after kidney biopsy. The inclusion criteria consisted of RT recipients who met the indications for kidney biopsy, which included abnormal urine examination results, elevated serum creatinine levels, delayed graft function, rejection, and protocol biopsy. The exclusion criteria included claustrophobia, intrauterine device (IUD) placement, and other contraindications to MRI or unavoidable artifacts that severely affected image quality.
MRI data
All participants were subjected to 1.5-T MRI (Ingenia Ambition, Philips Healthcare, Best, the Netherlands) with a 28-channel phased array coil. No special patient preparation was required before MRI examination. Axial T1, T2-weighted, and coronal T2-weighted images were first acquired for morphological evaluation. For DTI, the imaging parameters were as follows: b=0 and 600 s/mm2, 15 diffusion directions, repetition time (TR) =2,300 ms, echo time (TE) =67 ms, slice spacing =1 mm, slice thickness =5 mm, 20 slices/patient, voxel size =2.5×2.5×5 mm3, and FOV =320×400 mm2. For ASL, images with a voxel size of 3.75×3.75×8 mm3 were obtained using pseudo-continuous pulsed ASL along with a three-dimensional (3D) gradient and spin-echo scheme. The imaging parameters were as follows: TE =15 ms, TR =3,963 ms, turbo spin echo (TSE) factor =20, echo planar imaging (EPI) factor =15, matrix =64×60, and FOV =240×240 mm2.
Image post-processing
DTI and ASL data were transferred to a workstation (Intellispace Portal; v10; Philips Healthcare). Images were postprocessed by an abdominal radiologist with more than 10 years of clinical experience who was blinded to clinical and pathological findings. Firstly, DTI data were registered and corrected. FA and apparent diffusion coefficient (ADC) maps of the transplanted kidney were reconstructed. Appropriate cortical and medullary ROIs were delineated on T2 images with high corticomedullary contrast and directly applied to the corresponding FA and ADC maps. At least three image slices near the renal hilum were selected for ROI analysis for each participant. The measured medullary and cortical FA and ADC values were averaged. For ASL, ROI was placed in the renal cortex in a proton density-weighted image and transferred to the obtained renal blood flow (RBF) map to calculate renal perfusion. ROI should be avoided to include cysts, hemorrhage, and areas of heterogeneous signal intensity.
Kidney allograft biopsy
The degree of fibrosis requires the results of renal biopsy as the reference standard. All renal biopsy results were made by a nephropathologist with periodic acid-Schiff (PAS), hematoxylin-eosin (HE), Jones staining, periodic acid-silver methenamine (PASM), and Masson’s trichrome staining, according to the Banff 2019 model (17), without knowledge of the MRI results. The interstitial fibrosis score (ci score) was classified as follows: ci0 (no fibrosis, <5% cortical fibrosis), ci1 (mild fibrosis, 5–25% cortical fibrosis), ci2 (moderate fibrosis, 26–50% cortical fibrosis), and ci3 (severe fibrosis, >50% cortical fibrosis). For better statistical analysis, we divided the fibrosis group into no fibrosis (ci0) versus with fibrosis (ci1–3), no or mild fibrosis (ci0–1) versus moderate to severe fibrosis (ci2–3), and non-severe fibrosis (ci0–2) versus severe fibrosis (ci3).
Statistical analysis
The role of functional MRI parameters in identifying the degree of fibrosis of allografts can be evaluated by the receiver operating characteristic (ROC) curve. Previous studies (18,19) showed that the lowest acceptable area under the ROC curve (AUC) for the diagnosis of allograft fibrosis ci0 versus ci1–3, ci0–1 versus ci2–3, and ci0–2 versus ci3 was 0.70, 0.75, and 0.80, respectively. The best cut-off value was determined according to the maximum Youden index (sensitivity + specificity − 1). Comparison of ROC curve results required the use of DeLong test. The correlation between functional MRI parameters and Banff fibrosis score can be explored by calculating Spearman rank correlation coefficient. One-way analysis of variance (ANOVA) was used to compare the differences in functional MRI parameters between different fibrosis subgroups. When ANOVA results were significant, the post-hoc Bonferroni test was used to compare the groups.
Results
Clinical results
Of 145 patients recruited, two were excluded because they did not complete the MRI scan and another two were excluded because their data could not be postprocessed. Finally, a total of 141 patients were included (83 males and 58 females; average age was 42±11 years, range, 17–67 years). Biopsy results showed that the number of cases in the ci0, ci1, ci2, and ci3 groups were 64, 32, 31, and 14, respectively. The clinical and laboratory characteristics of the participants are shown in Table 1.
Table 1
Parameters | ci0 (n=64) | ci1 (n=32) | ci2 (n=31) | ci3 (n=14) | Total |
---|---|---|---|---|---|
Age (years) | 39±11 | 44±11 | 42±11 | 46±10 | 42±11 |
Sex | |||||
Male | 32 [50] | 21 [66] | 22 [71] | 9 [64] | 83 [59] |
Female | 32 [50] | 11 [34] | 9 [29] | 5 [36] | 58 [41] |
Baseline Scr (μmol/L) | 115.3 [83.4–158.4] | 131.4 [97.1–172.1] | 139.8 [113.1–194.0] | 181.5 [124.5–274.4] | 131.7 [96.7–177.2] |
Baseline eGFR (mL/min/1.73 m2) | 62 [39–88] | 52 [40–75] | 49 [35–58] | 32 [23–52] | 54 [34–74] |
Urinary protein (g/24 h) | 0.40 [0.13–0.52] | 0.32 [0.14–1.04] | 0.37 [0.14–1.97] | 0.80 [0.28–1.60] | 0.38 [0.14–1.02] |
Albumin (g/L) | 41.4±4.1 | 39.4±3.7 | 38.8±4.6 | 37.4±7.1 | 40.0±4.7 |
Hemoglobin (g/L) | 105.8±21.9 | 125.9±23.0 | 121.4±20.5 | 109.6±15.5 | 114.1±22.9 |
Systolic blood pressure (mmHg) | 135.3±17.0 | 132.7±18.7 | 131.7±17.9 | 136.5±15.8 | 134.0±17.4 |
Diastolic blood pressure (mmHg) | 85.5±9.9 | 82.7±10.7 | 83.8±11.9 | 84.5±11.3 | 84.4±10.6 |
Height (cm) | 167.0±9.0 | 168.6±9.2 | 161.9±28.7 | 164.3±6.5 | 166.0±15.5 |
Weight (kg) | 59.8±13.2 | 64.9±14.1 | 65.1±12.6 | 59.3±5.0 | 62.1±12.9 |
Hypertension (%) | 65 | 88 | 70 | 43 | 69 |
Diabetes (%) | 6 | 3 | 3 | 7 | 5 |
Immunosuppressive regimens | |||||
Pre + MMF + Tac | 61 [95] | 25 [78] | 28 [90] | 12 [86] | 126 [90] |
Pre + MMF + CsA | 3 [5] | 3 [9] | 2 [7] | 1 [7] | 9 [6] |
Others | 0 | 4 [13] | 1 [3] | 1 [7] | 6 [4] |
Data are presented as mean ± SD, median [Q1–Q3], n [%], or %. ci0, no fibrosis (<5% of cortex occupied by fibrosis); ci1, mild fibrosis (5–25% of cortex occupied by fibrosis); ci2, moderate fibrosis (26–50% of cortex occupied by fibrosis); ci3, severe fibrosis (>50% of cortex occupied by fibrosis). SD, standard deviation; CsA, cyclosporine A; MMF, mycophenolate mofetil; Pre, prednisone; Tac, tacrolimus; eGFR, estimated glomerular filtration rate; Scr, serum creatinine.
Relationships between functional MRI parameters and Banff fibrosis score
Cortical FA was negatively related to Banff fibrosis score (rho =−0.30, P<0.001). Medullary FA was also negatively related to Banff fibrosis score (rho =−0.50, P<0.001). Cortical ADC showed a negative relation to Banff fibrosis score (rho =−0.19, P<0.05). Medullary ADC showed a negative relation to Banff fibrosis score (rho =−0.22, P<0.01). There was a negative relation between RBF and Banff fibrosis score (rho =−0.43, P<0.001) (Figure 1).
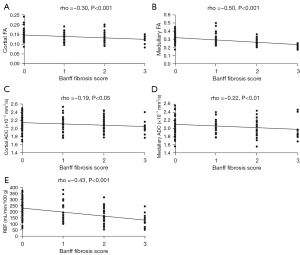
Differences in functional MRI parameters between fibrosis subgroups
The ci0 group [mean ± standard deviation (SD), 2.13±0.19 ×10−3 mm2/s], ci1 group (2.13±0.19 ×10−3 mm2/s), ci2 group (2.09±0.18 ×10−3 mm2/s), ci3 group (2.02±0.15 ×10−3 mm2/s) cortical ADC values decreased with the progression of fibrosis, but there was no statistically significant difference. The cortical FA value in the ci0 group (0.15±0.03, P<0.05) was significantly higher than that in the ci2 group (0.13±0.03) and ci3 group (0.13±0.02). There was no significant difference in cortical FA value between the ci1 group (0.14±0.03) and other groups. The ci0 group (2.09±0.24 ×10−3 mm2/s), ci1 group (2.06±0.19 ×10−3 mm2/s), ci2 group (2.05±0.26 ×10−3 mm2/s), and ci3 group (1.93±0.23 ×10−3 mm2/s) medullary ADC values decreased gradually with the progression of fibrosis, but there was no significant difference. The medullary FA values in the ci2 (0.27±0.04, P<0.001) and ci3 (0.21±0.03, P<0.001) groups were significantly lower than those in the ci0 group (0.31±0.05). The medullary FA value in the ci3 group (0.21±0.03) was significantly lower than that in the ci1 group (0.30±0.07, P<0.001) and ci2 group (0.27±0.04, P<0.01). The RBF value of the ci0 group (228.39±75.12 mL/min/100 g) was significantly higher than that of the ci2 group (165.87±66.75 mL/min/100 g, P<0.001) and ci3 group (127.79±50.36 mL/min/100 g, P<0.001). The RBF value in the ci1 group (196.46±73.91 mL/min/100 g, P<0.05) was significantly higher than that in the ci3 group (127.79±50.36 mL/min/100 g) (Figures 2-4).
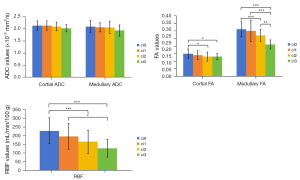
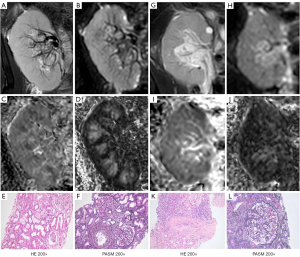
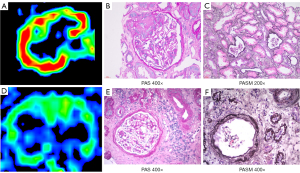
The role of DTI and ASL in fibrosis staging
In the comparison between ci0 and ci1–3, the diagnostic performance of medullary FA was better than that of RBF, but there was no statistical significance (AUC =0.725 vs. 0.712, P>0.05). When 0.268 was used as the cut-off value of medullary FA, the sensitivity was 84.4% and the specificity was 53.2%. The diagnostic performance of RBF combined with medullary FA was not significantly better than that of medullary FA alone (AUC =0.746 vs. 0.725, P>0.05). In the comparison between ci0–1 and ci2–3, medullary FA was better than RBF (AUC =0.787 vs. 0.735, P>0.05), but the results were not statistically significant. When 0.266 was used as the cut-off value of medullary FA, the sensitivity and specificity were 79.2% and 66.7%, respectively. The diagnostic performance of RBF combined with medullary FA was not significantly better than that of medullary FA alone (AUC =0.811 vs. 0.787, P>0.05). In the comparison between ci0–2 and ci3, medullary FA was significantly better than RBF (AUC =0.945 vs. 0.802, P<0.05), the results were statistically significant. When the cut-off value of medullary FA was 0.251, the sensitivity was 81.1% and the specificity was 99.9%. Compared with medullary FA alone, the combination of RBF and medullary FA did not significantly increase the diagnostic efficacy (AUC =0.958 vs. 0.945, P>0.05) (Table 2).
Table 2
Parameters | ci0 vs. ci1–3 | ci0–1 vs. ci2–3 | ci0–2 vs. ci3 |
---|---|---|---|
ASL (RBF) | |||
AUC | 0.712 | 0.735 | 0.802 |
P value | <0.001 | <0.001 | <0.001 |
Cut-off | 203.53 | 166.50 | 149.93 |
Sensitivity | 0.641 | 0.719 | 0.740 |
Specificity | 0.714 | 0.667 | 0.786 |
DTI (medullary FA) | |||
AUC | 0.725 | 0.787 | 0.945 |
P value | <0.001 | <0.001 | <0.001 |
Cut-off | 0.268 | 0.266 | 0.251 |
Sensitivity | 0.844 | 0.792 | 0.811 |
Specificity | 0.532 | 0.667 | 0.999 |
RBF is in the unit of mL/min/100 g. ci0 vs. ci1–3, no fibrosis vs. with fibrosis; ci0–1 vs. ci2–3, no or mild fibrosis vs. moderate to severe fibrosis; ci0–2 vs. ci3, non-severe fibrosis vs. severe fibrosis. DTI, diffusion tensor imaging; ASL, arterial spin labeling; AUC, area under the curve; RBF, renal blood flow; FA, fractional anisotropy.
Discussion
By assessing the degree of fibrosis in renal allograft, it is possible to identify high-risk patients with potential progression to allograft dysfunction in advance, and carry out corresponding clinical intervention. Most previous MRI studies of renal allograft fibrosis have been inadequate due to the limitation of the number of transplanted kidney biopsies (18,20-23). In this study, we collected data from a relatively large sample and compared the value of DTI and ASL in identifying the degree of fibrosis in allografts. We found that DTI had better diagnostic performance than ASL, and the results were statistically different in the discrimination between the ci0–2 and ci3 groups.
In the present study, we used the fibrosis results obtained from biopsy as the reference standard, and found that the cortical ADC values decreased with fibrosis progression in each group but did not reach statistical significance. Cortical FA values were significantly higher in the ci0 group (mean ± SD, 0.15±0.03, P<0.05) than in the ci2 (0.13±0.03) and ci3 (0.13±0.02) groups. This may be attributed to the progression of renal fibrosis, which is accompanied by the destruction of cortical microstructure and microcirculation. As a result, it has an impact on cortical DTI. In an earlier study, Razek et al. (24) found that the renal cortical parameters obtained by DTI were correlated with laboratory indicators, and the changes of laboratory indicators were closely correlated with the disruption of renal cortical microstructure and microcirculation. For the medullary ADC values, each group also showed a decreasing trend with the progression of fibrosis, but the difference was not statistically significant. For medullary FA values, the ci2 (0.27±0.04, P<0.001) and ci3 (0.21±0.03, P<0.001) groups were significantly lower than the ci0 (0.31±0.05) group, and the ci3 group (0.21±0.03) was significantly lower than the ci1 (0.30±0.07, P<0.001) and ci2 (0.27±0.04, P<0.01) groups, which was consistent with the previous results of Hueper et al. (25), which found that medullary FA and ADC values were negatively correlated with the degree of fibrosis, and the results of other researchers (26-29). Similar results were obtained in previously published studies, the diffusion anisotropy of renal allografts was higher in the renal medulla than in the cortex because the longitudinal arrangement of renal tubules within the medulla as well as the glomeruli within the cortex limit the direction of water molecule diffusion (30,31). We found that RBF decreased gradually with the progression of fibrosis. The RBF in the ci0 group (228.39±75.12 mL/min/100 g) was significantly higher than that in the ci2 (165.87±66.75 mL/min/100 g, P<0.001) and ci3 groups (127.79±50.36 mL/min/100 g, P<0.001), and that in the ci1 group (196.46±73.91 mL/min/100 g, P<0.05) was significantly higher than that in the ci3 group (127.79±50.36 mL/min/100 g). Both RBF and diffusion parameter values were negatively correlated with the degree of fibrosis. This is similar to the previous results of Yu et al. (16). However, the study by Yu et al. suggested that compared with diffusion parameters, ASL has a stronger correlation with fibrosis and a higher diagnostic efficiency for the degree of fibrosis. In this study, we chose DTI to compare with ASL. Compared with IVIM sequence, the FA values obtained by DTI can better reflect the diffusion degree of water molecules in each direction. We found a stronger correlation between medullary FA values and fibrosis scores than RBF. DTI had a higher diagnostic efficiency than ASL in the differentiation of fibrosis stages, and the results were statistically different in the differentiation of the ci0–2 and ci3 groups (AUC =0.945 vs. 0.802, P<0.05). This may indicate that the effect of the fibrotic response on the transplanted kidney is more on the direction of water diffusion, possibly due to the accumulation of fibrotic matrix which hinders the diffusion of water molecules. These results indicate that DTI is more sensitive than ASL in noninvasive identification of fibrosis severity in renal allografts. Compared with DTI alone, the combination of DTI and ASL has no significant improvement in the diagnosis of renal allograft fibrosis of different degrees, indicating that the added value of ASL to DTI is limited. In addition, ASL has its limitations and cannot distinguish the reduction of renal perfusion function caused by fibrosis or other damage factors (32-35).
Our study has certain limitations. First, the sample size in the ci3 group is small, which may have caused selection bias and the results to have certain errors. Second, due to the limitation of MRI technology itself, it is impossible to distinguish the changes of parameters caused by fibrotic and non-fibrotic factors. The actual parameter values may have not only been affected by fibrosis. In addition, the samples obtained by biopsy have limitations and may not accurately reflect the true situation of fibrosis in the transplanted kidney.
Conclusions
DTI has a higher diagnostic value than ASL in noninvasively differentiating the degree of fibrosis in RT. The FA value obtained by DTI can well reflect the degree of diffusion of water molecules in all directions. With the progression of fibrosis, FA value can show statistically significant changes. Compared with the RBF value obtained by ASL, the medullary FA value has a stronger correlation with fibrosis score, and the diagnostic efficiency is higher.
Acknowledgments
None.
Footnote
Reporting Checklist: The authors have completed the STROBE reporting checklist. Available at https://qims.amegroups.com/article/view/10.21037/qims-24-1023/rc
Funding: This work was supported by
Conflicts of Interest: All authors have completed the ICMJE uniform disclosure form (available at https://qims.amegroups.com/article/view/10.21037/qims-24-1023/coif). The authors have no conflicts of interest to declare.
Ethical Statement: The authors are accountable for all aspects of the work in ensuring that questions related to the accuracy or integrity of any part of the work are appropriately investigated and resolved. The study was conducted in accordance with the Declaration of Helsinki (as revised in 2013) and approved by the Ethics Review Board of The First Affiliated Hospital of Soochow University (2022) (No. 412). Informed consent was provided by all individual participants. for participants under 18 years, informed consent was provided by their legal guardians
Open Access Statement: This is an Open Access article distributed in accordance with the Creative Commons Attribution-NonCommercial-NoDerivs 4.0 International License (CC BY-NC-ND 4.0), which permits the non-commercial replication and distribution of the article with the strict proviso that no changes or edits are made and the original work is properly cited (including links to both the formal publication through the relevant DOI and the license). See: https://creativecommons.org/licenses/by-nc-nd/4.0/.
References
- Echeverria-Chasco R, Martin-Moreno PL, Aramendía-Vidaurreta V, Garcia-Ruiz L, Mora-Gutiérrez JM, Vidorreta M, Villanueva A, Cano D, Bastarrika G, Garcia-Fernandez N, Fernández-Seara MA. Diagnostic and Prognostic Potential of Multiparametric Renal MRI in Kidney Transplant Patients. J Magn Reson Imaging 2024;60:1650-63. [Crossref] [PubMed]
- Gaston RS, Fieberg A, Hunsicker L, Kasiske BL, Leduc R, Cosio FG, Gourishankar S, Grande J, Mannon RB, Rush D, Cecka JM, Connett J, Matas AJ. Late graft failure after kidney transplantation as the consequence of late versus early events. Am J Transplant 2018;18:1158-67. [Crossref] [PubMed]
- Bane O, Lewis SC, Lim RP, Carney BW, Shah A, Fananapazir G. Contemporary and Emerging MRI Strategies for Assessing Kidney Allograft Complications: Arterial Stenosis and Parenchymal Injury, From the AJR Special Series on Imaging of Fibrosis. AJR Am J Roentgenol 2024;222:e2329418. [Crossref] [PubMed]
- Keith DS, Vranic G, Nishio-Lucar A. Graft Function and Intermediate-Term Outcomes of Kidney Transplants Improved in the Last Decade: Analysis of the United States Kidney Transplant Database. Transplant Direct 2017;3:e166. [Crossref] [PubMed]
- Roufosse C, Simmonds N, Clahsen-van Groningen M, Haas M, Henriksen KJ, Horsfield C, Loupy A, Mengel M, Perkowska-Ptasińska A, Rabant M, Racusen LC, Solez K, Becker JU A. 2018 Reference Guide to the Banff Classification of Renal Allograft Pathology. Transplantation 2018;102:1795-814. [Crossref] [PubMed]
- Zhan T, Lou A. Comparison of outcomes of an 18-gauge vs 16-gauge ultrasound-guided percutaneous renal biopsy: a systematic review and meta-analysis. Ren Fail 2023;45:2257806. [Crossref] [PubMed]
- Rajiah PS, Suman G, Vijay K, Venugopal N, Mansoori B, Chalian M, Agarwal AK. Multisystem Imaging Manifestations of Kidney Failure. Radiographics 2024;44:e230124. [Crossref] [PubMed]
- Jiang B, Li J, Wan J, Tian Y, Wu P, Xu R, Yu Y, Wang X, Hu L, Zhu M. Arterial spin labeling combined with T1 mapping for assessment of kidney function and histopathology in patients with long-term renal transplant survival after kidney transplantation. Quant Imaging Med Surg 2024;14:2415-25. [Crossref] [PubMed]
- Wypych-Klunder K, Adamowicz A, Lemanowicz A, Szczęsny W, Włodarczyk Z, Serafin Z. Diffusion-weighted MR imaging of transplanted kidneys: Preliminary report. Pol J Radiol 2014;79:94-8. [Crossref] [PubMed]
- Baues M, Dasgupta A, Ehling J, Prakash J, Boor P, Tacke F, Kiessling F, Lammers T. Fibrosis imaging: Current concepts and future directions. Adv Drug Deliv Rev 2017;121:9-26. [Crossref] [PubMed]
- Buchanan CE, Mahmoud H, Cox EF, McCulloch T, Prestwich BL, Taal MW, Selby NM, Francis ST. Quantitative assessment of renal structural and functional changes in chronic kidney disease using multi-parametric magnetic resonance imaging. Nephrol Dial Transplant 2020;35:955-64. [Crossref] [PubMed]
- Li J, An C, Kang L, Mitch WE, Wang Y. Recent Advances in Magnetic Resonance Imaging Assessment of Renal Fibrosis. Adv Chronic Kidney Dis 2017;24:150-3. [Crossref] [PubMed]
- Jiang K, Ferguson CM, Lerman LO. Noninvasive assessment of renal fibrosis by magnetic resonance imaging and ultrasound techniques. Transl Res 2019;209:105-20. [Crossref] [PubMed]
- Morrell GR, Zhang JL, Lee VS. Magnetic Resonance Imaging of the Fibrotic Kidney. J Am Soc Nephrol 2017;28:2564-70. [Crossref] [PubMed]
- Leung G, Kirpalani A, Szeto SG, Deeb M, Foltz W, Simmons CA, Yuen DA. Could MRI Be Used To Image Kidney Fibrosis? A Review of Recent Advances and Remaining Barriers. Clin J Am Soc Nephrol 2017;12:1019-28. [Crossref] [PubMed]
- Yu YM, Wang W, Wen J, Zhang Y, Lu GM, Zhang LJ. Detection of renal allograft fibrosis with MRI: arterial spin labeling outperforms reduced field-of-view IVIM. Eur Radiol 2021;31:6696-707. [Crossref] [PubMed]
- Loupy A, Haas M, Roufosse C, Naesens M, Adam B, Afrouzian M, et al. The Banff 2019 Kidney Meeting Report (I): Updates on and clarification of criteria for T cell- and antibody-mediated rejection. Am J Transplant 2020;20:2318-31. [Crossref] [PubMed]
- Wang W, Yu Y, Wen J, Zhang M, Chen J, Cheng D, Zhang L, Liu Z. Combination of Functional Magnetic Resonance Imaging and Histopathologic Analysis to Evaluate Interstitial Fibrosis in Kidney Allografts. Clin J Am Soc Nephrol 2019;14:1372-80. [Crossref] [PubMed]
- Bane O, Hectors SJ, Gordic S, Kennedy P, Wagner M, Weiss A, Khaim R, Yi Z, Zhang W, Delaney V, Salem F, He C, Menon MC, Lewis S, Taouli B. Multiparametric magnetic resonance imaging shows promising results to assess renal transplant dysfunction with fibrosis. Kidney Int 2020;97:414-20. [Crossref] [PubMed]
- Xu X, Palmer SL, Lin X, Li W, Chen K, Yan F, Li X. Diffusion-weighted imaging and pathology of chronic kidney disease: initial study. Abdom Radiol (NY) 2018;43:1749-55. [Crossref] [PubMed]
- Woo S, Cho JY, Kim SY, Kim SH. Intravoxel incoherent motion MRI-derived parameters and T2* relaxation time for noninvasive assessment of renal fibrosis: An experimental study in a rabbit model of unilateral ureter obstruction. Magn Reson Imaging 2018;51:104-12. [Crossref] [PubMed]
- Yu YM, Ni QQ, Wang ZJ, Chen ML, Zhang LJ. Multiparametric Functional Magnetic Resonance Imaging for Evaluating Renal Allograft Injury. Korean J Radiol 2019;20:894-908. [Crossref] [PubMed]
- Mao W, Zhou J, Zeng M, Ding Y, Qu L, Chen C, Ding X, Wang Y, Fu C, Gu F. Intravoxel incoherent motion diffusion-weighted imaging for the assessment of renal fibrosis of chronic kidney disease: A preliminary study. Magn Reson Imaging 2018;47:118-24. [Crossref] [PubMed]
- Razek AAKA, Al-Adlany MAAA, Alhadidy AM, Atwa MA, Abdou NEA. Diffusion tensor imaging of the renal cortex in diabetic patients: correlation with urinary and serum biomarkers. Abdom Radiol (NY) 2017;42:1493-500. [Crossref] [PubMed]
- Hueper K, Khalifa AA, Bräsen JH, Vo Chieu VD, Gutberlet M, Wintterle S, Lehner F, Richter N, Peperhove M, Tewes S, Weber K, Haller H, Wacker F, Gwinner W, Gueler F, Hartung D. Diffusion-Weighted imaging and diffusion tensor imaging detect delayed graft function and correlate with allograft fibrosis in patients early after kidney transplantation. J Magn Reson Imaging 2016;44:112-21. [Crossref] [PubMed]
- Inoue T, Kozawa E, Okada H, Inukai K, Watanabe S, Kikuta T, Watanabe Y, Takenaka T, Katayama S, Tanaka J, Suzuki H. Noninvasive evaluation of kidney hypoxia and fibrosis using magnetic resonance imaging. J Am Soc Nephrol 2011;22:1429-34. [Crossref] [PubMed]
- Zhao J, Wang ZJ, Liu M, Zhu J, Zhang X, Zhang T, Li S, Li Y. Assessment of renal fibrosis in chronic kidney disease using diffusion-weighted MRI. Clin Radiol 2014;69:1117-22. [Crossref] [PubMed]
- Donnola SB, Piccone CM, Lu L, Batesole J, Little J, Dell KM, Flask CA. Diffusion tensor imaging MRI of sickle cell kidney disease: initial results and comparison with iron deposition. NMR Biomed 2018; [Crossref] [PubMed]
- Gaudiano C, Clementi V, Busato F, Corcioni B, Orrei MG, Ferramosca E, Fabbri E, Berardi P, Santoro A, Golfieri R. Diffusion tensor imaging and tractography of the kidneys: assessment of chronic parenchymal diseases. Eur Radiol 2013;23:1678-85. [Crossref] [PubMed]
- Hueper K, Gutberlet M, Rodt T, Gwinner W, Lehner F, Wacker F, Galanski M, Hartung D. Diffusion tensor imaging and tractography for assessment of renal allograft dysfunction-initial results. Eur Radiol 2011;21:2427-33. [Crossref] [PubMed]
- Notohamiprodjo M, Dietrich O, Horger W, Horng A, Helck AD, Herrmann KA, Reiser MF, Glaser C. Diffusion tensor imaging (DTI) of the kidney at 3 tesla-feasibility, protocol evaluation and comparison to 1.5 Tesla. Invest Radiol 2010;45:245-54. [Crossref] [PubMed]
- Cai YZ, Li ZC, Zuo PL, Pfeuffer J, Li YM, Liu F, Liu RB. Diagnostic value of renal perfusion in patients with chronic kidney disease using 3D arterial spin labeling. J Magn Reson Imaging 2017;46:589-94. [Crossref] [PubMed]
- Ren T, Wen CL, Chen LH, Xie SS, Cheng Y, Fu YX, Oesingmann N, de Oliveira A, Zuo PL, Yin JZ, Xia S, Shen W. Evaluation of renal allografts function early after transplantation using intravoxel incoherent motion and arterial spin labeling MRI. Magn Reson Imaging 2016;34:908-14. [Crossref] [PubMed]
- Selby NM, Blankestijn PJ, Boor P, Combe C, Eckardt KU, Eikefjord E, et al. Magnetic resonance imaging biomarkers for chronic kidney disease: a position paper from the European Cooperation in Science and Technology Action PARENCHIMA. Nephrol Dial Transplant 2018;33:ii4-ii14. [Crossref] [PubMed]
- Mendichovszky I, Pullens P, Dekkers I, Nery F, Bane O, Pohlmann A, et al. Technical recommendations for clinical translation of renal MRI: a consensus project of the Cooperation in Science and Technology Action PARENCHIMA. MAGMA 2020;33:131-40. [Crossref] [PubMed]