Myocardial strain characteristics in ST-segment elevation myocardial infarction patients with left ventricular thrombus: a cardiac magnetic resonance imaging study
Introduction
Acute left ventricular thrombus (LVT) refers to the fresh thrombus formed within the left ventricle within 2–4 weeks after an acute myocardial infarction (1). LVT is a serious complication after ST-segment elevation myocardial infarction (STEMI) and is an independent predictor of long-term adverse cardiovascular events. Studies have shown that the risk of death and thrombotic complications such as systemic thromboembolism and cerebral stroke in patients with LVT is higher than that in patients without LVT (2,3) . In addition, when patients with LVT receive antithrombotic treatment, dangerous bleeding events may also occur (2,3). The optimization and popularization of reperfusion treatment programs for STEMI and the improvement of perioperative care have improved the survival rate of acute myocardial infarction and reduced the incidence of LVT (4). However, 4–46% of patients with acute myocardial infarction still develop LVT (4-6). Although the mechanism of LVT is not yet clear, previous studies have identified many risk factors, such as reduced left ventricular ejection fraction (LVEF), infarct size, obstruction of the left anterior descending branch, and aneurysm (6,7). Cardiac magnetic resonance (CMR) has high soft tissue resolution and myocardial tissue characteristics, which can clearly determine the presence and location of LVT (3,8). CMR multi-parametric imaging is applied in the diagnosis of myocardial diseases, such as T1 mapping, T2 mapping, and myocardial strain analysis based on tissue tracking technology (3). Myocardial strain analysis can accurately assess the global and segmental circumferential strain (CS), radial strain (RS), and longitudinal strain (LS) of the myocardium. This study analyzed the myocardial strain characteristics of patients with STEMI without left ventricular aneurysm, analyzing the mechanical information of myocardial movement in patients with LVT. We present this article in accordance with the STROBE reporting checklist (available at https://qims.amegroups.com/article/view/10.21037/qims-24-1951/rc).
Methods
The study was conducted in accordance with the Declaration of Helsinki (as revised in 2013). The study was approved by institutional ethics board of Tianjin Chest Hospital (No. 2023YS-025-1) and informed consent was provided by all the patients.
Data source and study population
A total of 310 STEMI patients admitted to the emergency department and cardiology department of Tianjin Chest Hospital from January 2021 to June 2024 were collected in this study (Figure 1), and 282 patients who underwent CMR examination within 1 week after percutaneous coronary intervention (PCI) were included. A total of 40 patients with previous myocardial infarction were excluded, 29 of whom had undergone PCI and 11 of whom had undergone coronary artery bypass grafting. A further 38 patients with ventricular aneurysm were also excluded from this study. In addition, 5 patients with poor image quality due to poor cooperation were excluded.
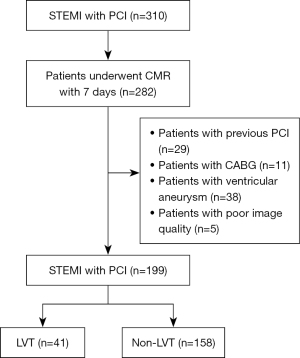
CMR scan
CMR examinations in all patients were performed using 3.0 T magnetic resonance imaging (MRI) scans (Ingenia, Philips Healthcare, Best, The Netherlands) using a 32-channel body phased array coil. The patient was lying on their back, connected to R-wave trigger vector electrocardiogram (ECG) gating on the chest and placed with a body coil. Scan protocols included cardiac cine and myocardial delayed enhancement, short axis (8–12 layers), two-chamber (3 layers), and four-chamber (3 layers). Short axis cine and late gadolinium enhancement (LGE) sequences covered the left ventricle. All sequences were acquired at end-expiratory breath hold.
The parameters of execution sequence were as follows: (I) cine sequence: balanced steady state free precession (b-SSFP) sequence, repetition time (TR) =2.8 ms, echo time (TE) =1.4 ms, slice thickness =8 mm, slice spacing =2 mm, field of view (FOV) =300 mm × 300 mm, acquisition voxel =2 mm × 1.6 mm, reconstruction voxel =1 mm × 1 mm, flip angle 45°, parallel acquisition acceleration factor 1.5, acquisition bandwidth 1,775.6 Hz; (II) T2-weighted image shot time inversion recovery (T2-STIR) sequence: turbo spin echo (TSE) sequence, TR =2 beat (bpm <75) or 3 beat (bpm ≥75), TE =75 ms, slice thickness =8 mm, slice spacing =2 mm, FOV =300 mm × 300 mm, acquisition voxel =1.4 mm × 1.75 mm, reconstruction voxel =0.88 mm × 0.88 mm, flip angle 90°, parallel acquisition acceleration factor 1.5, acquisition bandwidth 561.8 Hz; (III) LGE sequence: Phase-sensitive inversion recovery (PSIR) sequence was used. After contrast injection, scanning was delayed 10–15 minutes, TR =5.2 ms, TE =2.6 ms, FOV =300 mm × 300 mm, slice thickness =8 mm, slice spacing =2 mm, acquisition volume =1.6 mm × 1.9 mm, reconstruction voxel =0.9 mm × 0.9 mm, flip angle 15°, parallel acquisition acceleration factor 1.5, acquisition bandwidth 306.4 Hz. The contrast agent was injected with 0.2 mmol/kg gadolinium-diethylenetriamine pentaacetic acid (DTPA; Magnevist Bayer Healthcare, Berlin, Germany) followed by 15 mL of saline.
Image analysis
Cardiac function and myocardial strain analysis were performed using cvi42 (version 5.11.4, Circle Cardiovascular Imaging Inc., Calgary, Canada).
Cardiac function analysis
In the cardiac function module, analysis was conducted on the short-axis cine images, where the endocardial and epicardial contours of the left ventricle were automatically traced on the end-diastolic and end-systolic images, followed by manual correction to ensure accuracy. The software automatically calculated cardiac functional parameters such as left ventricular end-diastolic volume (LVEDV), left ventricular end-systolic volume (LVESV), left ventricular stroke volume (LVSV), LVEF, left ventricular myocardial mass index (LVMI), and cardiac output (CO). The average thickness of each myocardial segment was measured, and the thickening rate of each segment was calculated. The calculation formula was as follows: ventricular wall thickening rate = (systolic thickness − diastolic thickness)/diastolic thickness * 100%.
Myocardial strain analysis
In the tissue-tracking module, analysis was performed on the short-axis and three long-axis cine images. The software automatically traced the endocardial and epicardial contours and manually corrected them to ensure accuracy and determined the insertion points of the septum and the lower part of the interventricular septum on the short-axis plane for segmentation according to the recognized standard model. The software automatically tracked the displacement of the endocardial and epicardial contours, and manually adjusted the planes that were not accurately recognized. The long-axis of the left ventricle, from the base to the apex, was divided into three parts: the basal segment, the mid-cavity segment, and the apical segment. On the short-axis plane, the left ventricle was divided into 17 segments according to the American Heart Association (AHA) (9). Due to the partial volume effect, the 17th segment on the short-axis was not conducive to observation and has a larger measurement error, so it was excluded from the analysis. Segments 1–16 are as follows: the basal segment includes 6 myocardial segments (basal segment near the anterior wall of the interventricular septum, basal segment near the lower wall of the interventricular septum, basal segment of the anterior wall, basal segment of the lower wall, basal segment near the lateral wall of the interventricular septum, basal segment near the lower lateral wall), the mid-cavity segment includes 6 myocardial segments (mid-cavity segment near the anterior wall of the interventricular septum, mid-cavity segment near the lower wall of the interventricular septum, mid-cavity segment of the anterior wall, mid-cavity segment of the lower wall, mid-cavity segment near the lateral wall of the interventricular septum, mid-cavity segment near the lower lateral wall), and the apical segment includes 4 myocardial segments (apical segment of the interventricular septum, apical segment of the anterior wall, apical segment of the lower wall, apical segment of the lateral wall). The software automatically calculated the overall myocardial strain parameters and the strain parameters of each myocardial segment.
Definition of LVT
On CMR, LVT is defined as a mass within the LV cavity, which, if present, is judged by: (I) a mass that can be distinguished from the papillary muscles, trabeculations, and septal band; (II) a mass with low signal on T1-weighted image (T1WI) and gradient echo sequence images; and (III) no contrast filling on the delayed enhancement sequence.
Statistical analyses
Statistical analysis was performed using SPSS version 24.0 (IBM Corp., Armonk, NY, USA). Continuous data with a normal distribution were expressed as mean ± standard deviation. Continuous data that are not normally distributed were presented as medians and interquartile ranges. Categorical data were presented in frequency and percentage terms. Statistical comparisons between the two groups were performed using t-test (normal distribution), Mann-Whitney U test (non-normal distribution data), and chi-square test (categorical data). All statistical analyses were two-tailed and a P value of <0.05 was considered statistically significant.
Results
Comparison of general clinical data
In this study, 199 patients were included, with 41 cases of LVT and 158 cases without thrombus. The basic information and clinical characteristics of the two groups of patients are shown in Table 1. There were no significant differences in the average age, height, weight, and body mass index (BMI) between the two groups. The prevalence of hypertension, hyperlipidemia, diabetes, and the proportion of smokers were also not different between the two groups. The laboratory test results of creatinine, hemoglobin, and hematocrit in both groups showed no significant differences. The B-type natriuretic peptide (BNP) level in the thrombus group was significantly higher than that in the non-thrombus group (P<0.001).
Table 1
Characteristics | LVT (+) group (n=41) | LVT (−) group (n=158) | t/χ2 | P value |
---|---|---|---|---|
Age (years) | 57.98±13.77 | 60.17±11.72 | −1.030 | 0.353 |
Male | 32 (78.05) | 125 (79.11) | 0.022† | 0.882 |
Height (cm) | 166.39±6.25 | 164.40±6.79 | 1.701 | 0.627 |
Weight (kg) | 58.56±6.39 | 57.31±7.19 | 1.014 | 0.355 |
BMI (kg/m2) | 21.21±2.58 | 21.28±2.99 | −0.150 | 0.215 |
Hypertension | 24 (58.54) | 75 (47.47) | 1.595† | 0.207 |
Hyperlipidemia | 26 (63.41) | 77 (39.87) | 2.81† | 0.094 |
Diabetes mellitus | 22 (53.66) | 79 (50.00) | 0.174† | 0.676 |
Smoking | 22 (53.66) | 66 (41.77) | 1.865† | 0.172 |
BNP (pg/mL) | 1,533.37±1,274.08 | 770.53±757.06 | 4.908 | <0.001 |
Creatinine (mg/dL) | 0.81±0.12 | 0.84±0.12 | −1.262 | 0.209 |
Hemoglobin (g/dL) | 12.89±1.66 | 12.41±1.52 | 1.767 | 0.079 |
Hematocrit (%) | 39.70±4.67 | 39.27±5.39 | 0.475 | 0.635 |
Data are presented as n (%) or mean ± standard deviation. †, the chi-square tests. BMI, body mass index; BNP, B-type natriuretic peptide; LVT, left ventricular thrombus.
Cardiac function parameters of the two groups
The LVEF of patients in the thrombus group was significantly lower than that in the non-thrombus group. The left ventricular volume of patients in the thrombus group was higher than that in the non-thrombus group during both systole and diastole. There were no significant differences in heart rate, LVSV, LVMI, and the maximum thickness of the interventricular septum between the two groups. When analyzed by segment, the myocardial thickening rate at the apex of the heart in patients with thrombus was lower than that in the non-thrombus group, with significantly reduced thickening rates in segments 13, 14, and 16 (P<0.001). Although there were no statistical differences in other segments, the myocardial thickening rates in the thrombus group were all lower than those in the non-thrombus group, as shown in Table 2.
Table 2
Characteristics | LVT(+) group (n=41) | LVT(−) group (n=158) | t | P value |
---|---|---|---|---|
Heart rate (bpm) | 75.22±16.18 | 73.54±11.34 | 0.769 | 0.443 |
LVEF (%) | 28.51±16.21 | 37.05±17.69 | −2.801 | 0.006 |
LVEDV (mL) | 261.23±126.68 | 216.78±122.76 | 2.052 | 0.041 |
LVESV (mL) | 196.16±122.63 | 147.29±111.41 | 2.451 | 0.015 |
LVSV (mL) | 62.14±24.61 | 69.49±34.6 | −1.278 | 0.203 |
Cardiac output (L/min) | 4.67±2.05 | 5.08±2.7 | −0.896 | 0.371 |
LVMI (g) | 162.11±68.86 | 149.99±67.97 | 1.014 | 0.312 |
Maximum inter-ventricular septal thickness (mm) | 10.99±2.57 | 11.63±3.52 | −1.082 | 0.281 |
Myocardial thickening rate of each segment | ||||
1 | 18.84±25.56 | 14.17±20.84 | 1.217 | 0.225 |
2 | 3.39±18.58 | −3.31±21.62 | 1.816 | 0.071 |
3 | −0.34±15.85 | −6.32±19.86 | 1.785 | 0.076 |
4 | 11.60±20.55 | 5.97±22.55 | 1.454 | 0.148 |
5 | 21.93±24.94 | 22.01±24.05 | −0.016 | 0.987 |
6 | 28.99±27.13 | 29.68±25.34 | −0.152 | 0.879 |
7 | 40.09±64.22 | 46.67±39.71 | −0.820 | 0.413 |
8 | 24.64±30.33 | 35.28±36.72 | −1.709 | 0.089 |
9 | 23.23±25.22 | 28.83±44.27 | −0.777 | 0.438 |
10 | 33.12±67.41 | 33.72±41.57 | −0.071 | 0.943 |
11 | 37.25±61.71 | 41.45±38.13 | −0.546 | 0.586 |
12 | 38.76±64.05 | 47.47±40.38 | −1.077 | 0.283 |
13 | 21.84±23.39 | 51.15±46.18 | −3.931 | <0.001 |
14 | 23.84±18.38 | 48.19±38.85 | −3.896 | <0.001 |
15 | 31.27±32.97 | 41.59±35.10 | −1.698 | 0.091 |
16 | 25.73±25.53 | 50.53±41.27 | −3.665 | <0.001 |
Data are presented as mean ± standard deviation. CMR, cardiac magnetic resonance; EDV, end-diastolic volume; ESV, end-systolic volume; LV, left ventricular; LVEF, left ventricular ejection fraction; LVT, left ventricular thrombus; MI, mass index; SV, stroke volume.
Myocardial strain parameters of the two groups
There were differences in the overall global circumferential strain (GCS), global radial strain (GRS), and global longitudinal strain (GLS) between the two groups. Differences were also observed in the CS, RS, and LS of the mid-cavity and apical segments. Except for RS, which showed no difference, the CS and LS of the basal segment also exhibited differences, as seen in Table 3. The strain parameters of each segment for both groups were analyzed separately, and the results are depicted in Table 3 and Figure 2.
Table 3
Characteristics | LVT (+) group (n=41) | LVT (−) group (n=158) | t | P value |
---|---|---|---|---|
Global (%) | ||||
GCS | −10.84±5.05 | −14.71±6.94 | 3.347 | 0.001 |
GRS | 19.31±12.05 | 26.62±16.22 | −2.699 | 0.008 |
GLS | −4.28±4.62 | −6.55±5.24 | 2.53 | 0.012 |
Basal (%) | ||||
BCS | −20.6±8.43 | −26.25±11.37 | 2.974 | 0.003 |
BRS | 20.22±10.13 | 19.05±8.66 | 0.742 | 0.459 |
BLS | −18.3±8.99 | −21.54±6.87 | 2.512 | 0.013 |
Mid-cavity (%) | ||||
MCS | −9.52±5.51 | −12.24±5.92 | 2.655 | 0.009 |
MRS | 30.05±18.42 | 44.72±26.06 | −3.388 | 0.001 |
MLS | −5.97±11.7 | −9.58±3.61 | 3.328 | 0.001 |
Apical (%) | ||||
ACS | −9.81±6.49 | −14.47±8.28 | 3.345 | 0.001 |
ARS | 32.3±17.21 | 50.56±25.69 | −4.304 | <0.001 |
ALS | −3.74±13.79 | −6.41±4.17 | 2.099 | 0.037 |
Data are presented as mean ± standard deviation. ACS, apical circumferential strain; ALS, apical longitudinal strain; ARS, apical radial strain; BCS, basal circumferential strain; BLS, basal longitudinal strain; BRS, basal radial strain; CMR, cardiac magnetic resonance; GCS, global circumferential strain; GLS, global longitudinal strain; GRS, global radial strain; LVT, left ventricular thrombus; MCS, mid-cavity circumferential strain; MLS, mid-cavity longitudinal strain; MRS, mid-cavity radial strain.
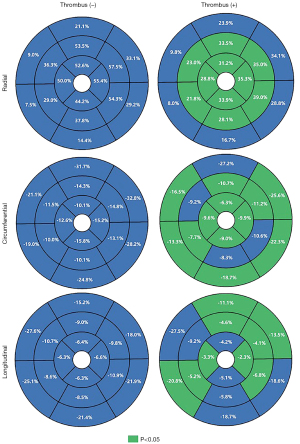
Reproducibility
A total of 30 patients were randomly selected to analyze the intra- and inter-observer variability of GRS, GCS, and GLS, expressed as intraclass correlation coefficients (ICCs). The ICCs for intra- and inter-observer variability were 0.978 [95% confidence interval (CI): 0.976–0.996] and 0.991 (95% CI: 0.985–0.997) for GRS, 0.973 (95% CI: 0.968–0.995) and 0.989 (95% CI: 0.965–0.994) for GCS, and 0.971 (95% CI: 0.958–0.992) and 0.972 (95% CI: 0.959–0.987) for GLS, respectively.
Discussion
In this study, we retrospectively analyzed the data of patients with STEMI without ventricular aneurysm with or without LVT. We found that left ventricular function and myocardial strain parameters were worse in patients with LVT than in those with LVT; myocardial thickening was generally lower in patients with LVT than in those without LVT, especially at the apical segment. It is highlighted that CMR technique provides a new observation index for LVT formation in STEMI patients.
LVT is a well-recognized complication after myocardial infarction, with higher risks, such as thromboembolism (2). Studies have reported a wide range of incidence rates of LVT in patients with STEMI, up to 15–57% (2,3). With the development of PCI technology, the incidence of LVT has gradually decreased (4). Additionally, the detection rate of LVT is related to the imaging method, examination time, and frequency of examination (3,10). In this study, patients with ventricular aneurysm were excluded for the following reasons: first, slow blood flow or reflux within the aneurysm, leading to blood stasis and promoting thrombus formation; second, the thin walls of the aneurysm lose normal function during contraction, and damage to the endothelial cells of the inner lining increase the risk of thrombus formation within the aneurysm; third, the irregularity of the left ventricle due to aneurysm formation also affects the accuracy of myocardial strain evaluation. However, studies showed that when LVT appeared after STEMI (6,8).
Therefore, after excluding patients with ventricular aneurysm in this study, a total of 199 patients were included, with 41 patients diagnosed with LVT, an incidence of 20.6%, indicating that even without aneurysm, LVT still has a high incidence.
Left ventricular dysfunction is considered the strongest independent predictor, mainly due to reduced LVEF (10). When LVEF is reduced, myocardial contractility decreases significantly, leading to impaired cardiac pumping function and susceptibility to LVT. Studies have shown that an LVEF of ≤40% can be used to predict the occurrence of LVT (11,12). Some studies on LVT samples have excluded patients with higher LVEF, only including patients with LVEF ≤50%, which may exclude some patients with abnormal myocardial strain but normal LVEF, and therefore, the LVEF value was not used as an exclusion criterion (2,12,13). Our analysis of cardiac function in the two groups of patients showed that the LVEF of both groups was less than 40%, and the LVEF of the LVT group (28.51%) was significantly lower than that of the group without LVT (37.05%). The enlargement of the left ventricular volume also increases the risk of LVT while also having the capacity to affect the systolic and diastolic function of the left ventricle, leading to a reduction in LVEF; in addition, the increased volume causes blood to linger in the cardiac chamber and slow blood flow, which is conducive to thrombus formation (14,15). LV remodeling can be categorized into mechanical factors and biochemical mechanisms. The geometry of the left ventricle and wall stress are key mechanical determinants of LV remodeling. According to Laplace’s law, wall stress in the left ventricle is related to intraventricular pressure, the radius of the left ventricle, and the thickness of the LV myocardial wall. During myocardial infarction, the myocardium in the affected area loses its normal contraction ability and becomes stretched, resulting in a reduced rate of myocardial thickening and thinning of the myocardium. Alterations in biochemical mechanisms, including changes in cardiomyocytes, the extracellular matrix, the collagen fiber network, and the sympathetic nervous system, may contribute to the damage of endocardial cells. Collectively, these factors can lead to the formation of LVT (16-18). Our study showed that the systolic and diastolic volumes of the left ventricle in patients with LVT were significantly increased, and the myocardial thickening rate in the apical segment was significantly reduced, consistent with the above theory.
CMR’s feature tracking technology can quantitatively evaluate LV function and detect subclinical myocardial abnormalities early, without the need for additional scanning sequences (19). Compared to LVEF, myocardial strain is more sensitive to local myocardial tissue and motion remodeling. In our study, the overall GRS, GCS, and GLS in patients with LVT were reduced. We compared the strain parameters of the two groups from the basal, mid-cavity, and apical segments, and except for the RS of the basal segment, which showed no difference between the two groups, other parameters showed significant differences. Our results further confirm that myocardial strain can evaluate myocardial function at the overall level, more sensitively and with more parameters than LVEF (20). In addition, unlike previous studies, we further analyzed the myocardial strain of the two groups of patients from each segment. Most studies have not performed segmental analysis of the LV myocardium due to software universality issues or other reasons (3,21). We performed a 16-segment strain analysis of the left ventricle, and the strain parameters of multiple segments showed significant differences, especially in segments 7–16, but the performance of each segment in RS, CS, and LS was not consistent, especially in segments 1–6. This may be related to the composition and movement pattern of the LV myocardium. The LV myocardium is a helical muscle tissue, surrounding the central axis of the left ventricle in the form of a helical ring (22). Myocardial fibers are arranged layer by layer, and the arrangement direction changes with each layer, that is: the endocardium and the myocardium below the epicardium show opposite helical shapes, and the middle layer of the myocardium is annular, a triple-twisted geometric shape similar to a Möbius strip (22-24). The special arrangement, contraction, relaxation, and twisting movement patterns of the myocardium determine that the heart movement is a complex three-dimensional, dynamic process. This complex structure of the left ventricle is manifested as radial, longitudinal, and circumferential movements, with the endocardial and epicardial myocardial fibers showing longitudinal movement, and the middle layer of the myocardium showing radial movement (25). The basal segment of the left ventricle is at the edge of the myocardium, and its myocardial strain analysis is more complex, which may affect the accuracy of myocardial strain, possibly presenting the reason why some parameters in segments 1–6 show no difference (3,20). This is also one of the reasons why we excluded LV aneurysm.
BNP is a vasculature-active hormone produced by ventricular myocytes. Stimulated by factors such as myocardial ischemia, necrosis, injury, ventricular wall tension, and excessive pressure load, myocardial cells synthesize and release BNP. In cases of acute myocardial infarction, elevated BNP levels can indicate the extent of myocardial injury. BNP serves as a sensitive marker for compromised heart function, with its levels being closely associated with the severity of heart failure (26). Although the relationship between BNP and thrombosis formation remains unclear, some studies have indicated that patients with thrombosis exhibit significantly higher BNP levels than those with atherosclerotic thrombosis (26,27). The findings of this study further affirm that BNP reflects myocardial injury and compromised heart function, and also suggest a potential link between BNP and thrombosis formation.
Limitations
Our study was a single-center retrospective study, and further prospective, large-sample studies are needed to verify the differences in myocardial strain parameters evaluated by CMR in patients with LVT. Secondly, this study primarily focused on the functional and strain parameters of CMR, and it is necessary to incorporate more laboratory, blood, and clinical information, such as changes in parameters such as BNP and creatine kinase isoenzyme-MB (CK-MB). Thirdly, we did not analyze the relationship between the location and severity of coronary artery lesions and LVT. Finally, we need further follow-up of patients with LVT to evaluate the changes in myocardial strain and cardiac function during the treatment of thrombus patients.
Conclusions
Our study shows that there is still a high risk of LVT in patients with STEMI without LV aneurysm. We performed a quantitative analysis of CMR for these patients, and the LVEF of patients with LVT was lower, and the GLS, GRS, and GLS of the left ventricle were significantly lower than those without LVT. Although CMR is not currently a routine examination and follow-up examination for patients with STEMI, it has high potential value in the diagnosis and prediction of LVT.
Acknowledgments
None.
Footnote
Reporting Checklist: The authors have completed the STROBE reporting checklist. Available at https://qims.amegroups.com/article/view/10.21037/qims-24-1951/rc
Funding: This work was supported by
Conflicts of Interest: All authors have completed the ICMJE uniform disclosure form (available at https://qims.amegroups.com/article/view/10.21037/qims-24-1951/coif). The authors have no conflicts of interest to declare.
Ethical Statement: The authors are accountable for all aspects of the work in ensuring that questions related to the accuracy or integrity of any part of the work are appropriately investigated and resolved. The study was conducted in accordance with the Declaration of Helsinki (as revised in 2013). The study was approved by institutional ethics board of Tianjin Chest Hospital (No. 2023YS-025-1) and informed consent was provided by all the patients.
Open Access Statement: This is an Open Access article distributed in accordance with the Creative Commons Attribution-NonCommercial-NoDerivs 4.0 International License (CC BY-NC-ND 4.0), which permits the non-commercial replication and distribution of the article with the strict proviso that no changes or edits are made and the original work is properly cited (including links to both the formal publication through the relevant DOI and the license). See: https://creativecommons.org/licenses/by-nc-nd/4.0/.
References
- Albaeni A, Chatila K, Beydoun HA, Beydoun MA, Morsy M, Khalife WI. In-hospital left ventricular thrombus following ST-elevation myocardial infarction. Int J Cardiol 2020;299:1-6. [Crossref] [PubMed]
- Cruz Rodriguez JB, Okajima K, Greenberg BH. Management of left ventricular thrombus: a narrative review. Ann Transl Med 2021;9:520. [Crossref] [PubMed]
- Shi RY, Chen BH, Wu CW, Wesemann L, Hu J, Xu JR, Zhou Y, Tao Q, Wu LM. Left ventricular thrombus after acute ST-segment elevation myocardial infarction: multi-parametric cardiac magnetic resonance imaging with long-term outcomes. Int J Cardiovasc Imaging 2022;38:2373-84. [Crossref] [PubMed]
- Fabris E, Selvarajah A, Tavenier A, Hermanides R, Kedhi E, Sinagra G, Van't Hof A. Complementary Pharmacotherapy for STEMI Undergoing Primary PCI: An Evidence-Based Clinical Approach. Am J Cardiovasc Drugs 2022;22:463-74. [Crossref] [PubMed]
- Robinson AA, Jain A, Gentry M, McNamara RL. Left ventricular thrombi after STEMI in the primary PCI era: A systematic review and meta-analysis. Int J Cardiol 2016;221:554-9. [Crossref] [PubMed]
- Sacoransky E, Yu Jia Ke D, Dave P, Alexander B, El Sherbini A, Abunassar J, Abuzeid W. Incidence of left ventricular thrombus following STEMI in the modern era via multimodality imaging: A systematic review and meta-analysis. Int J Cardiol Heart Vasc 2024;52:101396. [Crossref] [PubMed]
- Kaolawanich Y, Boonyasirinant T. Usefulness of apical area index to predict left ventricular thrombus in patients with systolic dysfunction: a novel index from cardiac magnetic resonance. BMC Cardiovasc Disord 2019;19:15. [Crossref] [PubMed]
- Hooks M, Okasha O, Velangi PS, Nijjar PS, Farzaneh-Far A, Shenoy C. Left ventricular thrombus on cardiovascular magnetic resonance imaging in non-ischaemic cardiomyopathy. Eur Heart J Cardiovasc Imaging 2021;22:1425-33. [Crossref] [PubMed]
- Cerqueira MD, Weissman NJ, Dilsizian V, Jacobs AK, Kaul S, Laskey WK, Pennell DJ, Rumberger JA, Ryan T, Verani MSAmerican Heart Association Writing Group on Myocardial Segmentation and Registration for Cardiac Imaging. Standardized myocardial segmentation and nomenclature for tomographic imaging of the heart. A statement for healthcare professionals from the Cardiac Imaging Committee of the Council on Clinical Cardiology of the American Heart Association. Circulation 2002;105:539-42. [Crossref] [PubMed]
- Camaj A, Fuster V, Giustino G, Bienstock SW, Sternheim D, Mehran R, Dangas GD, Kini A, Sharma SK, Halperin J, Dweck MR, Goldman ME. Left Ventricular Thrombus Following Acute Myocardial Infarction: JACC State-of-the-Art Review. J Am Coll Cardiol 2022;79:1010-22. [Crossref] [PubMed]
- Chaosuwannakit N, Makarawate P. Left Ventricular Thrombi: Insights from Cardiac Magnetic Resonance Imaging. Tomography 2021;7:180-8. [Crossref] [PubMed]
- Alhassan DA, Waheed KB, Sharif MN, Ul Hassan MZ, Ghaffar F, Salem KS, Said EFM, Altalaq BM, Qarmash AO, Arulanantham ZJ. Detection of Left Ventricular Thrombi on Cardiac Magnetic Resonance Viability Studies. J Saudi Heart Assoc 2020;32:368-76. [Crossref] [PubMed]
- Bulluck H, Chan MHH, Paradies V, Yellon RL, Ho HH, Chan MY, Chin CWL, Tan JW, Hausenloy DJ. Incidence and predictors of left ventricular thrombus by cardiovascular magnetic resonance in acute ST-segment elevation myocardial infarction treated by primary percutaneous coronary intervention: a meta-analysis. J Cardiovasc Magn Reson 2018;20:72. [Crossref] [PubMed]
- Lipke C, Katoh M, Franke A, Krombach G, Buecker A, Kühl HP. The value of non-contrast harmonic transthoracic echocardiography for the detection of left ventricular thrombi in patients with cardiomyopathy: comparison with contrast-enhanced magnetic resonance imaging. Int J Cardiovasc Imaging 2007;23:479-87. [Crossref] [PubMed]
- Guo X, Chen J, Xu S, Wang C, Hu T, Guan Q, Yang X, Ye J, Li X, Sun B, Yu D, Dong H. Association between the insufficient improvement of the quantitative flow ratio and worsening outcomes in ST-segment elevated myocardial infarction: a multicentre prospective cohort study. Quant Imaging Med Surg 2024;14:2828-39. [Crossref] [PubMed]
- Levine GN, McEvoy JW, Fang JC, Ibeh C, McCarthy CP, Misra A, Shah ZI, Shenoy C, Spinler SA, Vallurupalli S, Lip GYHAmerican Heart Association Council on Clinical Cardiology. Council on Cardiovascular and Stroke Nursing; and Stroke Council. Management of Patients at Risk for and With Left Ventricular Thrombus: A Scientific Statement From the American Heart Association. Circulation 2022;146:e205-23. [Crossref] [PubMed]
- Reindl M, Lechner I, Holzknecht M, Tiller C, Fink P, Oberhollenzer F, Mayr A, Troger F, Pamminger M, Henninger B, Theurl M, Klug G, Brenner C, Bauer A, Metzler B, Reinstadler SJ. Improved detection of echocardiographically occult left ventricular thrombi following ST-elevation myocardial infarction. Eur Heart J Acute Cardiovasc Care 2023;12:703-10. [Crossref] [PubMed]
- Leancă SA, Crișu D, Petriș AO, Afrăsânie I, Genes A, Costache AD, Tesloianu DN, Costache II. Left Ventricular Remodeling after Myocardial Infarction: From Physiopathology to Treatment. Life (Basel) 2022;12:1111. [Crossref] [PubMed]
- Yang W, Xu J, Zhu L, Zhang Q, Wang Y, Zhao S, Lu M. Myocardial Strain Measurements Derived From MR Feature-Tracking: Influence of Sex, Age, Field Strength, and Vendor. JACC Cardiovasc Imaging 2024;17:364-79. [Crossref] [PubMed]
- Gröschel J, Kuhnt J, Viezzer D, Hadler T, Hormes S, Barckow P, Schulz-Menger J, Blaszczyk E. Comparison of manual and artificial intelligence based quantification of myocardial strain by feature tracking-a cardiovascular MR study in health and disease. Eur Radiol 2024;34:1003-15. [Crossref] [PubMed]
- Zhao Y, Cui J, Zhang X, Li J, Yang J, Li T. Right ventricular function and determining factors of dysfunction in ST-segment-elevation myocardial infarction: a cross-sectional study with cardiac magnetic resonance imaging (MRI). Quant Imaging Med Surg 2024;14:6895-907. [Crossref] [PubMed]
- Poveda F, Gil D, Martí E, Andaluz A, Ballester M, Carreras F. Helical structure of the cardiac ventricular anatomy assessed by diffusion tensor magnetic resonance imaging with multiresolution tractography. Rev Esp Cardiol (Engl Ed) 2013;66:782-90. [Crossref] [PubMed]
- Antúnez Montes OY. Anatomical Correlation of the Helical Structure of the Ventricular Myocardium Through Echocardiography. Rev Esp Cardiol (Engl Ed) 2020;73:153-60. [Crossref] [PubMed]
- Antúnez-Montes OY, Kocica MJ, Olavarria AS, Corno AF, Millan RA, Rosales CI, Sanchez Aparicio HE. Helical structure of the ventricular myocardium. A narrative review of cardiac mechanics. Echocardiography 2023;40:161-73. [Crossref] [PubMed]
- Kocica MJ, Corno AF, Carreras-Costa F, Ballester-Rodes M, Moghbel MC, Cueva CN, Lackovic V, Kanjuh VI, Torrent-Guasp F. The helical ventricular myocardial band: global, three-dimensional, functional architecture of the ventricular myocardium. Eur J Cardiothorac Surg 2006;29:S21-40. [Crossref] [PubMed]
- Netala VR, Teertam SK, Li H, Zhang Z. A Comprehensive Review of Cardiovascular Disease Management: Cardiac Biomarkers, Imaging Modalities, Pharmacotherapy, Surgical Interventions, and Herbal Remedies. Cells 2024;13:1471. [Crossref] [PubMed]
- Kerr B, Brandon L. Atrial Fibrillation, thromboembolic risk, and the potential role of the natriuretic peptides, a focus on BNP and NT-proBNP - A narrative review. Int J Cardiol Heart Vasc 2022;43:101132. [Crossref] [PubMed]