Ultrasound cut-off values for muscle thickness, cross-sectional area, and echo intensity in Caucasian adults: a narrative review
Introduction
Background
The aging process is inevitably accompanied by the loss of muscle mass, a phenomenon well-documented in literature (1-4). When this decline in muscle mass or quality coexists with the loss of muscle function, it manifests as sarcopenia (1-4). The implications of sarcopenia extend beyond mere physical changes, encompassing increased risks of cardiovascular diseases, mobility disorders, compromised daily living activities, falls and fractures, loss of independence, and even mortality (1-7). If left untreated, sarcopenia imposes substantial personal, social, and economic burdens (8). Recognizing its significant impact, the European Working Group on Sarcopenia in Older People 2 (EWGSOP2) recommends routine screening for older individuals to prevent sarcopenia (9). However, the challenge lies in the diagnosis and prediction of sarcopenia, given the lack of clarity regarding variables, measurement methods, reference values, and the evaluation of therapeutic effectiveness (9).
The loss of muscle mass, as a part of the sarcopenia definition, can be assessed through magnetic resonance imaging (MRI) and computed tomography (CT) scans, considered gold standards but constrained by high costs, immobility requirements, the need for specialized personnel, and a dearth of reference values (9,10). In contrast, ultrasound emerges as a promising, practical alternative, boasting portability, speed, ease of use, and radiation-free applicability (11). Its validity and reliability have been confirmed through comparisons with MRI and CT scans (11-13). There is also interest in incorporating muscle quality by ultrasound, assessed through echo intensity, pennation angle, and fascicle length (14-16). Echo intensity is the most commonly used method in the literature for assessing muscle quality.
Ultrasound’s ability to regionally measure muscles offers potential diagnostic use (11,17). However, the absence of reference values poses a challenge in diagnosing low muscle mass and sarcopenia (17-19). While physical testing remains crucial for sarcopenia diagnosis, regional muscle measurements exhibit stronger correlations with total body muscle mass measurements (18). Notably, not all patients can perform these physical tests, as demonstrated in conditions such as rheumatoid arthritis, where joint deformities and destruction impact test outcomes (15). In such cases, regional muscle measurements become pivotal for accurate diagnosis and preventive interventions (15,18).
Objectives
This review is dedicated to presenting a comprehensive overview of reference values, specifically cut-off values, associated with ultrasound muscle measurements for predicting and diagnosing low muscle mass and low muscle quality, in the context of sarcopenia. Our objective is to delve into the detailed exploration of cut-off values for individual muscles and contribute to the refinement and standardization of ultrasound muscle measurements. We present this article in accordance with the Narrative Review reporting checklist (available at https://qims.amegroups.com/article/view/10.21037/qims-24-2122/rc).
Methods
We conducted a comprehensive search across multiple databases, including PubMed, Web of Science, and Scopus, to gather pertinent articles. Our primary aim was to identify articles that assessed reference, cut-off, minimum, or normal values for skeletal muscle parameters using ultrasound. Specifically, we focused on cut-off values for muscle thickness, cross-sectional area (CSA), and echo intensity. The examined muscles had to be skeletal muscles that contribute to movement or are used for diagnosing low muscle mass or sarcopenia. To ensure the relevance of our findings to human Caucasian adults, we excluded studies involving other ethnicities. Only English-language articles published until April 2024 were included in this review. A summary of the search strategy can be found in Table 1.
Table 1
Items | Specification |
---|---|
Date of search | April 09, 2024 |
Databases and other sources searched | PubMed, Web of Science, and Scopus |
Search terms used | Reference OR cut-off OR minimum OR normal values AND skeletal muscle OR muscle thickness OR cross-sectional area OR echo intensity AND ultrasound |
Timeframe | Until April 2024 |
Inclusion and exclusion criteria | Inclusion: skeletal muscles that contribute to movement or are used for diagnosing low muscle mass or sarcopenia; human Caucasian adults; English-language articles |
Exclusion: no ultrasound measured; non-skeletal muscles (such as the diaphragm or cardiac muscle); measurements with other parameters (such as abdominal circumference or muscle strength); reliability studies | |
Selection process | J.V.d.B. and S.H. screened the titles and abstracts of these articles independently |
A total of 975 articles were initially identified and imported into Rayyan (20), an online review tool. Following the identification process that involved removing 138 duplicate articles, 837 unique articles remained for further evaluation. The flow chart of the study selection is shown in Figure 1. Two independent researchers, J.V.d.B. and S.H., screened the titles and abstracts of these articles. Exclusion criteria comprised studies where muscle measurements were not measured with ultrasound, studies into non-skeletal muscles (such as the diaphragm or cardiac muscle), combinations of muscle measurements with other parameters (such as abdominal circumference or muscle strength), and research focusing on reliability rather than cut-off values. Consequently, 789 articles were excluded from the analysis. The remaining 48 articles underwent a thorough assessment of their full text by the same researchers to ensure their alignment with the study’s objectives. The articles underwent thorough evaluation using the Pretorius and Keating assessment tool to ensure their quality and reliability for ultrasound analysis (21). The Pretorius and Keating assessment tool is designed to score the quality of a study by assessing the methodology of ultrasound muscle measurement (21). The checklist includes ten questions that can be answered with ‘yes’, ‘no’, or ‘not stated/not clear’ (21). For every ‘yes’, the study gets 1 point (21). In case of ‘no’ or ‘not stated/not clear’, the study gets 0 points (21). A score of 7 points or higher indicates that the method is of high quality (21). A score lower than 7 indicates moderate quality. The quality assessment was performed by two independent authors and a discussion was held in case of disagreement on the score. To facilitate meaningful comparisons, we have opted to round all cut-off values in the text to one decimal place. This approach not only enhances the overall coherence of the findings but also deems two decimal places as potentially extraneous for clinical relevance. The effective values shown in the tables and figures are without rounding.
Results
Of the 48 full texts included, seventeen (22-38) were ultimately included in this review because they met the inclusion criteria. Table 2 presents the results of the quality assessment conducted using the Pretorius and Keating tool. Thirteen of the seventeen articles achieved high quality (22-34). The four studies that scored only 6 out of 10 mainly lacked the detailed description of the method, the description of the scanning point, and the transducer position (35-38). Table 3 offers detailed insights into the participants, ultrasound devices, and statistical methodologies employed across these studies. The most commonly used samples in the included articles consisted of healthy participants (22,23,26-29,32,34,35,37). Participants who were not considered healthy had sarcoidosis (24), head and neck cancer (25), systemic sclerosis (31), nephrology conditions (33), or were from the geriatric clinic (30,36,38). The most frequently used ultrasound brand was General Electric (23,24,28,31,34-36,38). Additionally, brands such as Sonos (22), Mindray (25), Aloka (26), Clarus (27), Hitachi (33), Acuson (34), and Esaote (30) were also used. The frequencies used ranged between 5 and 16 MHz (22-38). The ultrasound settings were actually not described in the majority of the articles (24,25,28-32,35-38). The most commonly used static method to determine the cut-off values was the receiver operating characteristic (ROC) method (23-25,28,30,31,33,36,38). Additionally, distribution theory (26,35) and the −2 standard deviations (−2 SD) (22,27,29,32,34,37) techniques were also used.
Table 2
Author | Blind final assessor | Data for at least 80% of cohort | Inception cohort | Assessment methods defined | Adequate detail reported | Timeframe | Muscle state | RTUS scanning point | RTUS contact pressure | Transducer positioning | Total Score |
---|---|---|---|---|---|---|---|---|---|---|---|
Abraham et al. [2020] (35) | 1 | 1 | 1 | 1 | 0 | 0 | 1 | 0 | 1 | 0 | 6 |
Arts et al. [2010] (22) | 0 | 1 | 1 | 1 | 1 | 1 | 1 | 1 | 1 | 1 | 9 |
Barotsis et al. [2020] (23) | 0 | 1 | 1 | 1 | 1 | 1 | 1 | 1 | 1 | 1 | 9 |
Esme et al. [2022] (24) | 1 | 1 | 0 | 1 | 0 | 1 | 1 | 0 | 1 | 1 | 7 |
Fernández-Jiménez et al. [2024] (25) | 0 | 1 | 0 | 1 | 0 | 1 | 1 | 1 | 1 | 1 | 7 |
Maurits et al. [2003] (26) | 0 | 1 | 1 | 1 | 1 | 1 | 1 | 0 | 0 | 1 | 7 |
Minetto et al. [2016] (27) | 0 | 1 | 1 | 1 | 1 | 1 | 1 | 1 | 1 | 1 | 9 |
Neira Alvares et al. [2021] (28) | 0 | 1 | 1 | 1 | 0 | 1 | 0 | 1 | 1 | 1 | 7 |
Rankin et al. [2005] (37) | 0 | 1 | 1 | 1 | 0 | 1 | 1 | 1 | 0 | 1 | 7 |
Rustani et al. [2019] (30) | 0 | 1 | 1 | 0 | 0 | 1 | 1 | 1 | 1 | 1 | 7 |
Sari et al. [2021] (31) | 1 | 1 | 0 | 1 | 0 | 1 | 1 | 1 | 1 | 1 | 8 |
Stokes et al. [2005] (32) | 1 | 1 | 1 | 1 | 0 | 1 | 1 | 1 | 0 | 1 | 8 |
Wilkinson et al. [2021] (33) | 0 | 1 | 0 | 1 | 1 | 1 | 0 | 1 | 1 | 1 | 7 |
Wilson et al. [2019] (34) | 0 | 1 | 1 | 1 | 1 | 1 | 0 | 1 | 1 | 1 | 8 |
Kuyumcu et al. [2016] (36) | 1 | 1 | 1 | 0 | 0 | 1 | 1 | 0 | 1 | 0 | 6 |
Sengul Aycicek et al. [2021] (38) | 1 | 1 | 1 | 0 | 0 | 1 | 1 | 0 | 1 | 0 | 6 |
Rankin et al. [2006] (29) | 0 | 1 | 1 | 1 | 0 | 1 | 1 | 1 | 0 | 0 | 6 |
RTUS, real time ultrasound.
Table 3
Author | Participants | Ultrasound device | Transducer | Ultrasound settings | Statistical method |
---|---|---|---|---|---|
Abraham et al. [2020] (35) | 65 healthy participants (63% women), mix of different ethnicities, 21–82 y | General Electric LOGIQ S7 Expert, Toronto, Canada | 15 MHz | Not described | Distribution theory: P5 |
Arts et al. [2010] (22) | 95 healthy Western European participants (48 women); 17–90 y | Sonos 2000 Phased Array Imaging System; Hewlett-Packard, Andover, Massachusetts | 7.5 MHz phased array | Gain: 86 dB; compression: 70 dB; depth: 4 cm | Normal values with 2 SD below or above the mean |
Barotsis et al. [2020] (23) | 94 consecutive individuals (67 women), Greece; 75.6±6.6 y | GE Logiq P9 | ML6–15 linear array | Gain: 50 dB; dynamic range: 66 dB; frequency: 10 MHz | ROC to predict sarcopenia |
Esme et al. [2022] (24) | 40 patients with sarcoidosis (31 women), Turkey; 52.3±12.5 y | GE Healthcare Logiq S7 R3 Expert | 9–12 MHz | Not described | ROC curves to predict sarcopenia |
Fernández-Jiménez et al. [2024] (25) | 494 patients with head and neck cancer (22% women), Spain; 63.9±10.1 y | Mindray Z60, Madrid, Spain | 10–12 MHz | Not described | ROC to predict survival in cancer patients |
Maurits et al. [2003] (26) | 145 healthy participants for MT (69 women); 68 healthy participants for EI (35 women); The Netherlands; 20–94 y | Aloka SSD-2000 | 7.5 MHz | Gain: 75 dB; dynamic range: C04 | Distribution theory: P10 and P90 |
Minetto et al. [2016] (27) | 60 younger adults (30 women); Italy; 26±3 y | ClarUs, Telemed, Vilnius, Lithuania | 5–12 MHz linear array | Gain: 50%; depth range, 3–6 cm | 2 SD below the mean |
Neira Álvares et al. [2021] (28) | 57 community-dwelling participants (33 women); Spain; 78.9 [74.9–81.9] y | General Electric Logic F6 | 11.5 MHz linear | Not described | ROC curves to predict low muscle mass |
Rankin et al. [2005] (37) | 99 sedentary or moderately active participants (53 women); United Kingdom; 18–72 y | ALOKA SSD 1200 | 5 MHz convex and 7.5 MHz linear | Not described | 2 SD below the mean |
Rustani et al. [2019] (30) | 119 patients from Italian hospital, Sarcopenic and non-sarcopenic; 82.8±7 y | MyLab 25GoldEsaote | 5–7.5 MHz | Not described | ROC curves to predict sarcopenia |
Sari et al. [2021] (31) | 93 patients with systemic sclerosis (86 women); Turkey; 52.6 [40.8–58.8] y | Logiq S7 R3 Expert, GE Healthcare, Chicago, IL, USA | 9–12 MHz linear | Not described | ROC curves to predict low muscle mass |
Stokes et al. [2005] (32) | 120 sedentary or moderately active participants (68 women); United Kingdom; 20–69 y | ALOKA SSD 1200 | 5 MHz convex | Not described | 2 SD below the mean |
Wilkinson et al. [2021] (33) | 113 nephrology outpatients (59 women); 86% white British ethnic background 62.0±14.1 y | EUB-6500; Hitachi Medical Systems, Twinsburg, OH, USA | 7.5 MHz linear | Gain: 58 dB | ROC curves to predict low muscle mass |
Wilson et al. [2019] (34) | 113 healthy younger adults (54 women); mix ethnicity (87% white); <35 y | Acuson Antares Premium Edition, Siemens, Mountainview, CA, USA | 7.27 MHz linear array | Gain: −3 Db; magnification factor: ×1; depth: 5–7 cm | 2 SD below the mean |
Voluson-i, GE Healtycare, Milwaukee, WI, USA | 15–16 MHz | ||||
Kuyumcu et al. [2016] (36) | 100 elderly from outpatient clinic (59 women); Turkey; 73.08±6.18 y | Logiq P5, General Electrics Medical Systems | 5–12 MHz linear | Not described | ROC to predict sarcopenia |
Sengul Aycicek et al. [2021] (38) | 136 Turkish patients from geriatric outpatient clinic (66% women); 74 [64–93] y | LOGİQ 200 PRO, General Electrics Medical Systems | 5–12 MHz linear | Not described | ROC to predict low muscle mass |
Rankin et al. [2006] (29) | 123 moderately active, healthy participants (68 women); London, United Kingdom; 20–72 y | ALOKA, Mitaka-shi, Tokyo, Japan, SSD 1200 | 5 MHz | Not described | 2SD below the mean |
EI, echo intensity; GE, general electric; MT, muscle thickness; P, percentile; ROC, receiver operating characteristic; SD, standard deviation; Y, years old.
Figure 2 presents a comprehensive overview of the measurement techniques and cut-off values specifically designed for evaluating the muscles in the arm and hand. These muscles can be assessed in either the supine (22,35) or prone (26) position, with scans taken in the transverse or cross-sectional plane (22,26,35). To determine the muscle thickness of the elbow flexors, which include the biceps brachii and brachialis muscles, as well as the biceps brachii muscle alone, measurements can be taken either two-thirds between the acromion and the elbow crease (22) or at the maximum circumference of the muscle (26). Muscle thickness is defined as the distance between the superficial aponeurosis and the humerus, while echo intensity is defined within the region of interest, excluding surrounding fascia or bone (22,26). Cut-off values have been defined according to factors such as sex (22,26,35), age (22,26), side (22), and weight (26). The diversity in cut-off value classifications reveals a spectrum; for men, cut-off values for muscle thickness range between 1.6 and 3.4 cm, while for women, the range is from 1.1 to 2.6 cm (22,26,35). Turning attention to echo intensity of the biceps brachii muscle, the cut-off values exhibit a broader span, varying between 36 and 156 arbitrary units (AU) (22,26). For the flexor muscles of the forearm (22) and the hand muscles (35), there is only one study that has determined cut-off values. The muscle thickness and echo intensity in the forearm are measured for the flexor group as a whole, without targeting a specific muscle. In contrast, the hand has separate cut-off values for the first dorsal interosseous, abductor pollicis brevis, and abductor digiti minimi muscles.
Figure 3 provides insights into the measuring method and cut-off values for the abdominal muscles. In the examination of participants, a supine position was adopted (24,29,31). The scans were executed in the transverse plane (24,31), with muscle thickness measured between the superficial and deep fascia (24,31) and the CSA measured tracing the inside of the muscle fascia (24,31). The rectus abdominis muscle was measured 2 cm above and lateral to the umbilicus (24,29,31). The study looked into the thickness of the rectus abdominis muscle, examining differences between males and females as well as differences between the right and left sides (29). The identified cut-off values for rectus abdominis muscle thickness range between 0.6 and 0.7 cm for women and between 0.6 and 0.8 cm for men (24,29,31). As for the cut-off value for the CSA, only one study determined cut-off values (29). For the lateral abdominal muscles, four distinct measuring sites were employed: (I) halfway between the twelfth rib and the iliac crest; (II) directly below the twelfth rib; (III) halfway along a line joining the anterior superior iliac spine (ASIS) to just below the ribcage in the mid-axillary line; and (IV) lateral to the rectus abdominis muscle at the level of the umbilicus (24,29,31). Examining the obliquus externus abdominis muscle, the cut-off ranges between 0.2 and 0.5 cm (24,29), depending on predictions for either a decrease in fat-free mass or sarcopenia (24,29), or sex (29). The cut-off value for the obliquus internus abdominis is determined only by one study (29). Investigating the transversus abdominis muscle, the cut-off value is dependent on the prediction method (24), side and sex (29) and ranges between 0.2 and 0.5 cm.
For the lumbar multifidus (32), masseter (23), geniohyoid (23), sternocleidomastoid (22), and posterior neck muscles (37), there was only one study with cut-off values for each muscle. The measurement method and cut-off values are shown in Figure 4. The lumbar multifidus, masseter, geniohyoid, and sternocleidomastoid muscles are each measured individually, while the posterior neck muscles are measured as a group.
Figure 5 provides an overview of the measuring methods and cut-off values for the quadriceps muscle. The determination of cut-off values for quadriceps muscle thickness, which includes the rectus femoris and vastus intermedius muscles, involved three distinct participant positions: supine (22-24,27,30,35), sitting (34), and prone (26). Additionally, three different measurement sites were employed: midpoint between the ASIS and superior border of the patella (22-24,27,33), midpoint between the greater trochanter femoris and the lateral condyle of the femur (26,30,34), and the one third point between the superior border of the patella and the ASIS (25). The scan planes varied between transverse (22,23,25,26,33,34) and longitudinal (23,26,27) in the referenced articles. For the quadriceps muscle, in the supine position, with the measurement site halfway between the ASIS and the patella, cut-off values for men ranged from 1.6 to 3.5 cm, and for women, they varied between 1.3 and 2.8 cm, contingent on age (22,23). In the sitting (34) and prone position (26), only one study in each position established a cut-off value. For the rectus femoris muscle alone, supine measurements halfway between the ASIS and the patella yielded cut-off values between 1.5 and 2.0 cm for men, and 1.5 and 1.6 cm for women (23,27). When measured between the greater trochanter and the lateral condyle of the femur, only one study established cut-off values (30). Regarding CSA, discrepancies exist among studies, with cut-off values ranging from 0.1 to 8.9 cm2 for men and 0.1 to 5.7 cm2 for women (24,25,33). Echo intensity of the rectus femoris muscle, as indicated by Arts et al. [2010] (22) and Maurits et al. [2003] (26), is influenced by age, with Arts et al. [2010] (22) considering sex as an influencing factor as well. Cut-off values range between 31 and 139.8 AU for men and 36 and 139.8 AU for women (22,26). For the vastus intermedius (23) and vastus lateralis muscles (27), there was only one study to establish cut-off values, which is shown in Figure 5.
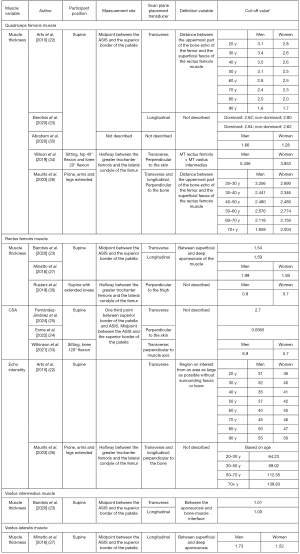
Figure 6 provides an overview of the measurement methodology and associated cut-off values for the muscles of the lower leg and foot. The assessment of the gastrocnemius muscle is consistently conducted in the prone position (23,24,27,28,31,36,38), with variations in scan planes observed across studies, including both longitudinal (23,27,28) and transverse (23,28,31) orientations. The scan focuses on capturing the muscle thickness between the superficial and deep fascia layers (23,24,27,28,31,36,38). Cut-off values for the gastrocnemius muscle are contingent upon several factors, including participant position, scan plane, measurement site, dominance, method of determination, and sex and range from 1.2 to 1.9 cm for men and women (23,24,27,28,31,36,38). The assessment of the tibialis anterior muscle involves a supine position, with measurements taken at the one-fourth point between the inferior aspect of the patella and the lateral malleolus (22,27,35). This measurement can be executed in either the transverse (22,35) or longitudinal plane (22,27). The muscle thickness is defined as the distance between the superficial fascia and the interosseous membrane and exhibit variations based on sex and age. For men, the values range between 1.7 and 2.3 cm, while for women, they vary from 1.6 to 2.2 cm (22,27,35). Echo intensity cut-off values were only established by one study (22). For the foot muscles, only one study established cut-off values for the abductor hallucis brevis and extensor digitorum brevis muscles, each measured individually.
Discussion
This review offers a comprehensive overview of the cut-off values pertaining to muscle thickness, CSA and echo intensity as assessed through ultrasound. Upon evaluating the quality of the included articles, it was found that four out of seventeen exhibited questionable measurement quality, while the remaining thirteen demonstrated commendable research quality.
For several muscle groups including the muscle thickness of the forearm flexor, hand, lumbar multifidus, masseter, geniohyoid, sternocleidomastoid, posterior neck, vastus intermedius, vastus lateralis, and foot muscles, and echo intensity of the rectus abdominis and tibialis anterior muscles, only one study each provided cut-off values. This limitation precludes comparison with other studies, thereby necessitating reliance solely on the values reported in those individual articles. In contrast, for the muscle thickness of the obliquus internus and externus abdominis, and the echo intensity of the rectus femoris and biceps brachii muscle, two studies each presented cut-off values. Furthermore, three studies addressed the muscle thickness of the elbow flexor, rectus abdominis, rectus femoris, and tibialis anterior muscle, and the CSA of the rectus femoris while five studies tackled the muscle thickness of the quadriceps muscle, and an extensive seven studies investigated the muscle thickness of the gastrocnemius muscle. Despite the commendable quality, arriving at a consensus regarding the cut-off values proves challenging due to the multitude of factors influencing their determination: sample characteristics, ultrasound measurement method, and statistical method. The ensuing discussion delves deeper into these confounding factors to shed light on the complexities underlying the establishment of cut-off values.
Confounding factors on the cut-off values
Sample characteristics
Sex
One predominant factor frequently used to establish cut-off values is sex. Among the seventeen studies reviewed, eleven incorporate separate cut-off values for men and women (22,26,27,29,30,32-35,37,38). For the gastrocnemius muscle, although cut-off values were determined irrespective of sex, it was observed that a higher percentage of women in the study corresponded to lower cut-off values for muscle thickness in the gastrocnemius muscle. This observation is further supported by the examination of the rectus abdominis muscle, revealing that a cut-off value, based on a group of men and women, is lower compared to a cut-off value specifically intended for men (24,29,31). Rankin et al. [2006] (29) demonstrated that the rectus abdominis muscle tends to be thicker in men compared to women.
Existing literature corroborates the documented differences in muscle thickness and CSA between men and women (39-42). Therefore, it is recommended to adhere to sex-specific cut-off values, recognizing the importance of accounting for sex variations in the assessment of muscle characteristics.
Age
Furthermore, age emerges as a potential explanatory factor for variations in cut-off values, as indicated in two out of the seventeen studies reviewed (22,26). However, it is crucial to underscore that the sample size used to establish cut-offs must be sufficiently large. A limitation observed in the study by Abraham et al. [2020] (35) is the small sample size, preventing the creation of age categories. Consequently, this study only provides a cut-off value based on sex, lacking the additional nuance of age-specific distinctions. Notably, in the case of the tibialis anterior muscle, cut-off values derived from a sample of 20 years old (27) are higher compared to those established from samples with diverse age categories (22,35).
Existing literature supports the notion that muscle thickness and CSA are influenced by age (40,43-46). Age is an important factor in the reference values as muscle thickness starts to decrease from the age of 42 years in women and 44 years in men (18). To enhance the practical applicability of cut-off values in the future, we recommend undertaking more extensive research to systematically explore age-dependent variations in these values.
Body weight
Body weight as a differentiating factor for cut-off values was only employed in the study by Maurits et al. [2003] (26). Arts et al. [2010] (22) suggest that weight accounts for 5% to 25% of the variation in muscle thickness, acknowledging its potential impact. However, the inclusion of weight in cut-off values is a topic of debate, particularly in neuromuscular disorders where weight loss is often attributed to muscle loss (22). This raises concerns that incorporating weight in cut-off values could yield false-negative outcomes in such cases (22).
Existing literature indicates that muscle thickness exhibits little to no correlation with body mass index (BMI) (47,48). Given the limited evidence supporting a substantial influence of weight on muscle thickness and CSA, the temporary adoption of cut-off values based on age and sex is recommended, rather than incorporating weight as a determinant.
Dominant side
Among the seventeen articles reviewed, four studies indicate potential differences in cut-off values based on dominance (22,23,29,36). The relevance of functional asymmetry in muscle ultrasound values has been a topic of discussion (49). Consequently, it is advised to explicitly specify whether the muscle measurements were taken on the dominant or non-dominant side in order to accurately interpret and apply cut-off values (43). Notably, the right-left difference in the arm muscles can also be interpreted as the distinction between the dominant and non-dominant arm, especially considering that only five out of the 95 participants in the sample by Arts et al. [2010] (22) were left-handed. The differences between the right and left or dominant and non-dominant sides in the included articles are below or just around 10%, which raises the question whether this is a real difference or a measurement error (50).
Health status of the participants
In some studies, healthy participants were used to determine a cut-off value for the muscles (22,26,27,29,32,34,35,37). In other studies, patients diagnosed with low muscle mass or sarcopenia were used to determine which cut-off value could be associated with these diagnoses (23-25,28,30,31,33,36,38).
For the rectus abdominis muscle thickness, cut-off values based on healthy participants (29) can be compared with those based on the diagnosis of sarcopenia (24) and low muscle mass (31). The cut-off value based on sarcopenia is slightly lower than those based on low muscle mass and healthy participants. Regardless of sex, it is observed that the cut-off value based on low muscle mass corresponds with the value based on healthy participants.
For the quadriceps muscle thickness, it is observed that the cut-off value is mainly influenced by age-related differences, as the cut-off value based on sarcopenia (23) corresponds with the value for young healthy participants (22).
For the gastrocnemius muscle, it is even seen that the cut-off values based on sarcopenia (23,24,36) are slightly higher compared to the cut-off values based on healthy participants (27). Most of the cut-off values based on low muscle mass (31,38) are similar to the cut-off values based on healthy participants.
The health status of the participants appears to have little impact on the determination of the cut-off values.
Ultrasound measurement method
Measurement site and patient positioning
The measurement site emerges as a critical variable influencing the interpretation of cut-off values in five distinct muscles: the elbow flexor muscles, lumbar multifidus muscle, quadriceps muscle, rectus femoris muscle, and gastrocnemius muscle. Some studies suggest measuring the elbow flexor and gastrocnemius muscles at their maximum bulk (23,24,26,31,36,38). However, the lack of a precisely defined maximum bulk location poses challenges for reproducibility, leading to discrepancies in cut-off values compared to those established with clearly defined measurement sites (22,27,28). Abraham et al. [2020] (35) further contribute to this challenge by not detailing the measurement site, rendering their muscle measurements impossible to reproduce. In the case of the quadriceps muscle, the distinction between two measurement sites—one halfway between the ASIS and the patella, and the other halfway between the greater trochanter and lateral femur condyle—are observed (22,23,26,34). The influence of the measurement site appears to be less pronounced, with Arts et al. [2010] (22), Barotsis et al. [2020] (23), and Maurits et al. [2003] (26) displaying little to no difference in cut-off values. Wilson et al.’s [2019] (34) higher cut-off values may be attributed to the measurement being conducted in a sitting position, emphasizing the potential impact of participant position over measurement site on quadriceps muscle thickness. In case of the lateral abdominal muscles, it is suggested that halfway between the twelfth rib and the iliac crest, halfway along a line joining the ASIS to just below the ribcage in the mid-axillary line, and lateral to the rectus abdominis muscle at the level of the umbilicus essentially describe the same anatomical site, advocating for their unified adoption over measurements directly below the twelfth rib. Notably, measurements directly below the twelfth rib yield lower cut-off values (29). The reason is probably because closer to the attachment, the muscle is thinner. At the level of the muscle belly, the muscle is thicker. To ensure optimal accuracy and consistency measuring the muscles of the arm and hand, adopting a supine position for participants or patients is recommended, with arms fully extended and relaxed (22,35). Conversely, measuring in a prone position (26) appears challenging for assessing the elbow flexor muscles effectively. Selecting the measurement site at the two-thirds point between the acromion and the elbow crease has been consistently described as the most reliable point (22). However, uncertainties arise regarding the determination of the maximum circumference of the muscle (26), as guidelines for this aspect remain unclear. To obtain precise measurements, ultrasound scans should be taken transversely, allowing for the assessment of muscle thickness between the superficial aponeurosis and the humerus (22,26,35). Notably, when measuring the muscle thickness of the elbow flexors, it is advisable to measure both the biceps brachii and brachialis muscles together (22,26,35).
Recognizing the lack of consistency in current ultrasound muscle assessments due to diverse measuring sites, Perkisas et al. [2018] (43) advocate for standardization in ultrasound muscle measurements. Their consensus proposal, particularly for muscles in the arms and legs, underscores the necessity of establishing uniform guidelines to enhance the practical utility of ultrasound in muscle assessments (43).
Ultrasound settings
Significant variations in cut-off values for echo intensity are observed for the biceps brachii and lateral abdominal muscles. These differences can be attributed to disparities in ultrasound settings. Arts et al. [2010] (22) and Maurits et al. [2003] (26) employed distinct gain settings, with Arts et al. [2010] (22) using a gain of 86 dB, while Maurits et al. [2003] (26) opted for 75 dB. Additionally, Arts et al. [2010] (22) set a depth of 4 cm, whereas Maurits et al. [2003] (26) did not specify the depth. Previous research underscores the influence of ultrasound settings, including gain and depth, on echo intensity results (51,52). Furthermore, the dynamic range in Maurits et al. [2003] (26) was set to C04, although this detail was not described in Arts et al. [2010] (22). Previous research into the impact of dynamic range settings on echo intensity revealed notable effects on the rectus femoris and rectus abdominis muscles (53). In addition, the echo intensity is influenced by the ultrasound device, the settings of the device and the orientation of the transducer (15). Consequently, we emphasize the need for better standardization and description of the ultrasound settings in echo intensity measurements. The lack of comprehensive information on current ultrasound settings precludes the establishment of guidelines for appropriate ultrasound configuration and corresponding cut-off values for echo intensity. Addressing this gap is essential for advancing the reliability and comparability of echo intensity measurements across studies, facilitating meaningful interpretations in the assessment of muscle characteristics.
Statistical method
The choice of statistical method for determining cut-off values has demonstrated varying impacts on different muscles. The predominant method employed across the included articles was the ROC analysis, which established the relation between cut-off values and low muscle mass or sarcopenia. Most studies defined low muscle mass using cut-off values based on bioelectrical impedance analysis (BIA) results (31,33,38). Only Neira Álvarez et al. [2021] (28) defined low muscle mass based on the cut-off value for calf circumference. Sarcopenia was also not defined consistently across all articles. The definitions used were based on cut-off values for BIA (24), BIA and handgrip strength (36), dual-energy X-ray absorptiometry (DXA) and handgrip strength (23), and handgrip strength, circumferences, and a walk test (30). The ROC method was used in half of the studies (23-25,28,30,31,33,36,38). Following closely behind, the second most prevalent approach was the utilization of −2 SD below the mean, implemented in six out of the seventeen articles (22,26,27,29,32,37). Additionally, the distribution method based on percentiles 5, 10, and 90 was employed in two of the seventeen studies (26,35). In the case of the elbow flexor muscles, cut-off values based on −2 SD (22) align closely with those derived from percentile 5 (35), but when determined based on percentile 10 (26), the cut-off values are higher. For the transversus abdominis muscle, various methods, including an ROC curve to predict fat-free mass (24), an ROC curve to predict low muscle mass (31), and −2 SD (29), yield similar cut-off values. However, the cut-off value based on an ROC curve to predict sarcopenia (24) is higher, emphasizing the nuanced distinctions in predicting different aspects of body composition. The predictive nature of low fat-free mass differs from predicting sarcopenia, given that fat-free mass encompasses bone mineral mass and connective tissue in addition to muscle mass (54,55). In contrast, the statistical method employed to determine cut-off values for the rectus abdominis and obliquus externus abdominis muscles does not seem to significantly influence the outcomes. Whether based on an ROC curve to predict fat-free mass (24), an ROC curve to predict sarcopenia (24), or −2 SD (29), the cut-off values remain similar. Similarly, for the quadriceps muscle, cut-off values derived from −2 SD (22), an ROC curve to predict sarcopenia (23), and percentile 10 (26) exhibit similarities, while those based on percentile 5 (35) are lower. However, it remains uncertain whether this discrepancy is attributable to the statistical or measurement method, as the measurement method was not described in the study by Abraham et al. [2020] (35).
For the gastrocnemius muscle, it is hypothesized that sex and measurement method exert more influence on cut-off values than the statistical method. Meanwhile, when the cut-off value for the muscle thickness of the tibialis anterior muscle is determined based on −2 SD (22) and the 5th percentile (35), the results are the same. However, In the study by Minetto et al. [2016] (27), which also used −2 SD, the cut-off values are higher compared to those in the studies of Arts et al. [2010] (22) and Abraham et al. [2020] (35). This is likely due to the sample used by Minetto et al. [2016] (27), as the age of the participants in their study was much lower compared to the participants in Arts et al. [2010] (22) and Abraham et al. [2020] (35).
Given the demonstrated impact of the methodology on cut-off values for certain muscles, it is imperative to ensure accurate and context-specific determination of these values. Although working with −2 SD can be effective, it is crucial to avoid reliance on calculations solely from a healthy young adult cohort (43). Instead, developing age-specific −2 SD cut-off values for each muscle within different age categories is recommended to enhance the precision and relevance of these measurements (23).
Limitations
This review exclusively incorporated research explicitly establishing cut-off values for muscle thickness, CSA, and echo intensity. The multitude of influencing factors on muscle measurements introduces a significant challenge. It is unclear which ones should be incorporated when establishing or using cut-offs. This limitation underscores the need for further research to elucidate the most pertinent influencing factors to enhance the precision and reliability of cut-off values. Another limitation of this review is a potential discrepancy in Figure 5, where the CSA cut-off values of the rectus femoris muscle are described. The CSA cut-off values deviate significantly from each other. While this is likely an error, it requires further clarification. We have revisited the source data and suspect that this value may not align with the intended result. Therefore, we recommend that this particular value be verified or updated to ensure the accuracy and consistency of the data. It is recommended to conduct more research primarily on the influence of age, the measurement method, and the positioning of the patient. If the cut-off values are determined based on −2 SD below the mean of a young sample, then in principle, the values of older individuals should fall within this −2 SD if they are healthy. It is important to standardize the measurement method and patient positioning to ensure that the measurement of muscles is clear and does not influence the interpretation of the results.
A final limitation is that this review, along with its cut-off values, is only applicable to a Caucasian population. Given the differences in body composition between ethnicities (56), further research is needed to establish reference values for muscles in other ethnic groups.
Summary: recommendations for clinical practice
This research serves as a comprehensive compilation of cut-off values for muscle thickness CSA, and echo intensity across various muscles, offering valuable insights for both research and practical applications. What clearly emerges from this review is that cut-off values need to be sex and age-specific. To apply the cut-off values in clinical practice, it is recommended to use the cut-off values for muscles that have been most extensively studied. The muscle thickness of the gastrocnemius muscle has been assessed up to seven times in the prone position (23,24,27,28,31,36,38) and has been linked to the prediction of low muscle mass (28,31,38) and sarcopenia (23,24,36).
Given the importance of sex-specific cut-off values, it is advised to measure the gastrocnemius muscle midway between the proximal and distal tendon insertions (27). For men, a cut-off value of 1.38 cm is recommended to identify those at risk, and a value of 1.23 cm is suggested to diagnose low muscle thickness. For women, values below 1.33 cm should be described as “at risk”, and values below 1.23 cm as indicative of low muscle thickness.
For the quadriceps muscle, it is recommended to measure in the supine position (22,23,35) and to use the cut-off values described by Arts et al. (22) for diagnosing low quadriceps muscle thickness, due to the availability of sex- and age-specific cut-off values.
Similarly, for the thickness of the elbow flexors, the sex- and age-specific cut-off values described by Arts et al. (22) are recommended for use.
For practical use of the rectus abdominis muscle thickness, the cut-off values provided by Rankin et al. (29) are recommended because they are sex-specific. However, since right-to-left differences are negligible, it is advised to use rounded cut-off values of 0.8 cm for men and 0.7 cm for women.
Lastly, the tibialis anterior muscle can also be used to diagnose low muscle thickness. For this muscle, the cut-off values provided by Arts et al. (22) are recommended, given their consideration of both sex and age. Muscles not mentioned here were either insufficiently studied or had cut-off values that varied significantly between studies, preventing the establishment of reliable consensus values.
This review represents an overview of muscle cut-off values providing a foundation for diagnosing conditions such as muscle atrophy, sarcopenia, and low muscle mass, offering clinicians a valuable tool for more precise assessments and facilitating early interventions. As our understanding of influencing factors and standardization improves, the potential for enhanced accuracy and applicability of muscle cut-off values in clinical settings is promising, paving the way for further advancements in the field of muscle assessment.
Acknowledgments
None.
Footnote
Reporting Checklist: The authors have completed the Narrative Review reporting checklist. Available at https://qims.amegroups.com/article/view/10.21037/qims-24-2122/rc
Funding: None.
Conflicts of Interest: All authors have completed the ICMJE uniform disclosure form (available at https://qims.amegroups.com/article/view/10.21037/qims-24-2122/coif). The authors have no conflicts of interest to declare.
Ethical Statement: The authors are accountable for all aspects of the work in ensuring that questions related to the accuracy or integrity of any part of the work are appropriately investigated and resolved.
Open Access Statement: This is an Open Access article distributed in accordance with the Creative Commons Attribution-NonCommercial-NoDerivs 4.0 International License (CC BY-NC-ND 4.0), which permits the non-commercial replication and distribution of the article with the strict proviso that no changes or edits are made and the original work is properly cited (including links to both the formal publication through the relevant DOI and the license). See: https://creativecommons.org/licenses/by-nc-nd/4.0/.
References
- Cruz-Jentoft AJ, Baeyens JP, Bauer JM, Boirie Y, Cederholm T, Landi F, Martin FC, Michel JP, Rolland Y, Schneider SM, Topinková E, Vandewoude M, Zamboni MEuropean Working Group on Sarcopenia in Older People. Sarcopenia: European consensus on definition and diagnosis: Report of the European Working Group on Sarcopenia in Older People. Age Ageing 2010;39:412-23. [Crossref] [PubMed]
- Beaudart C, Zaaria M, Pasleau F, Reginster JY, Bruyère O. Health Outcomes of Sarcopenia: A Systematic Review and Meta-Analysis. PLoS One 2017;12:e0169548. [Crossref] [PubMed]
- Lauretani F, Russo CR, Bandinelli S, Bartali B, Cavazzini C, Di Iorio A, Corsi AM, Rantanen T, Guralnik JM, Ferrucci L. Age-associated changes in skeletal muscles and their effect on mobility: an operational diagnosis of sarcopenia. J Appl Physiol (1985) 2003;95:1851-60. [Crossref] [PubMed]
- Rolland Y, Czerwinski S, Abellan Van Kan G, Morley JE, Cesari M, Onder G, Woo J, Baumgartner R, Pillard F, Boirie Y, Chumlea WM, Vellas B. Sarcopenia: its assessment, etiology, pathogenesis, consequences and future perspectives. J Nutr Health Aging 2008;12:433-50. [Crossref] [PubMed]
- Abramowitz MK, Hall CB, Amodu A, Sharma D, Androga L, Hawkins M. Muscle mass, BMI, and mortality among adults in the United States: A population-based cohort study. PLoS One 2018;13:e0194697. [Crossref] [PubMed]
- Shephard RJ. Physical activity and reduction of health risks: how far are the benefits independent of fat loss? J Sports Med Phys Fitness 1994;34:91-8.
- Srikanthan P, Karlamangla AS. Muscle mass index as a predictor of longevity in older adults. Am J Med 2014;127:547-53. [Crossref] [PubMed]
- Mijnarends DM, Luiking YC, Halfens RJG, Evers SMAA, Lenaerts ELA, Verlaan S, Wallace M, Schols JMGA, Meijers JMM. Muscle, Health and Costs: A Glance at their Relationship. J Nutr Health Aging 2018;22:766-73. [Crossref] [PubMed]
- Cruz-Jentoft AJ, Bahat G, Bauer J, Boirie Y, Bruyère O, Cederholm T, Cooper C, Landi F, Rolland Y, Sayer AA, Schneider SM, Sieber CC, Topinkova E, Vandewoude M, Visser M, Zamboni M. Writing Group for the European Working Group on Sarcopenia in Older People 2 (EWGSOP2), and the Extended Group for EWGSOP2. Sarcopenia: revised European consensus on definition and diagnosis. Age Ageing 2019;48:601. [Crossref] [PubMed]
- Beaudart C, McCloskey E, Bruyère O, Cesari M, Rolland Y, Rizzoli R, et al. Sarcopenia in daily practice: assessment and management. BMC Geriatr 2016;16:170. [Crossref] [PubMed]
- Nijholt W, Scafoglieri A, Jager-Wittenaar H, Hobbelen JSM, van der Schans CP. The reliability and validity of ultrasound to quantify muscles in older adults: a systematic review. J Cachexia Sarcopenia Muscle 2017;8:702-12. [Crossref] [PubMed]
- Ticinesi A, Meschi T, Narici MV, Lauretani F, Maggio M. Muscle Ultrasound and Sarcopenia in Older Individuals: A Clinical Perspective. J Am Med Dir Assoc 2017;18:290-300. [Crossref] [PubMed]
- Ismail C, Zabal J, Hernandez HJ, Woletz P, Manning H, Teixeira C, DiPietro L, Blackman MR, Harris-Love MO. Diagnostic ultrasound estimates of muscle mass and muscle quality discriminate between women with and without sarcopenia. Front Physiol 2015;6:302. [Crossref] [PubMed]
- Kawai H, Kera T, Hirayama R, Hirano H, Fujiwara Y, Ihara K, Kojima M, Obuchi S. Morphological and qualitative characteristics of the quadriceps muscle of community-dwelling older adults based on ultrasound imaging: classification using latent class analysis. Aging Clin Exp Res 2018;30:283-91. [Crossref] [PubMed]
- Tada M, Yamada Y, Mandai K, Hidaka N. Screening for sarcopenia and obesity by measuring thigh muscle and fat thickness by ultrasound in patients with rheumatoid arthritis. Osteoporos Sarcopenia 2021;7:81-7. [Crossref] [PubMed]
- Perkisas S, Bastijns S, Baudry S, Bauer J, Beaudart C, Beckwée D, et al. Application of ultrasound for muscle assessment in sarcopenia: 2020 SARCUS update. Eur Geriatr Med 2021;12:45-59. [Crossref] [PubMed]
- Andreoli A, Garaci F, Cafarelli FP, Guglielmi G. Body composition in clinical practice. Eur J Radiol 2016;85:1461-8. [Crossref] [PubMed]
- Kara M, Kaymak B, Ata AM, Özkal Ö, Kara Ö, Baki A, Şengül Ayçiçek G, Topuz S, Karahan S, Soylu AR, Çakır B, Halil M, Özçakar L. STAR-Sonographic Thigh Adjustment Ratio: A Golden Formula for the Diagnosis of Sarcopenia. Am J Phys Med Rehabil 2020;99:902-8. [Crossref] [PubMed]
- Abe T, Loenneke JP, Thiebaud RS, Ogawa M, Mitsukawa N. Age-related site-specific muscle loss in the thigh and zigzag walking performance in older men and women. Acta Physiol Hung 2014;101:488-95. [Crossref] [PubMed]
- Ouzzani M, Hammady H, Fedorowicz Z, Elmagarmid A. Rayyan-a web and mobile app for systematic reviews. Syst Rev 2016;5:210. [Crossref] [PubMed]
- Pretorius A, Keating JL. Validity of real time ultrasound for measuring skeletal muscle size. Physical Therapy Reviews 2008;13:415-26.
- Arts IM, Pillen S, Schelhaas HJ, Overeem S, Zwarts MJ. Normal values for quantitative muscle ultrasonography in adults. Muscle Nerve 2010;41:32-41. [Crossref] [PubMed]
- Barotsis N, Galata A, Hadjiconstanti A, Panayiotakis G. The ultrasonographic measurement of muscle thickness in sarcopenia. A prediction study. Eur J Phys Rehabil Med 2020;56:427-37. [Crossref] [PubMed]
- Eşme M, Karcıoğlu O, Öncel A, Ayçiçek GŞ, Deniz O, Ulaşlı SS, Köksal D, Doğu BB, Cankurtaran M, Halil M. Ultrasound Assessment of Sarcopenia in Patients With Sarcoidosis. J Ultrasound Med 2022;41:951-9. [Crossref] [PubMed]
- Fernández-Jiménez R, García-Rey S, Roque-Cuéllar MC, Fernández-Soto ML, García-Olivares M, Novo-Rodríguez M, González-Pacheco M, Prior-Sánchez I, Carmona-Llanos A, Muñoz-Jiménez C, Zarco-Rodríguez FP, Miguel-Luengo L, Boughanem H, García-Luna PP, García-Almeida JM. Ultrasound Muscle Evaluation for Predicting the Prognosis of Patients with Head and Neck Cancer: A Large-Scale and Multicenter Prospective Study. Nutrients 2024;16:387. [Crossref] [PubMed]
- Maurits NM, Bollen AE, Windhausen A, De Jager AE, Van Der Hoeven JH. Muscle ultrasound analysis: normal values and differentiation between myopathies and neuropathies. Ultrasound Med Biol 2003;29:215-25. [Crossref] [PubMed]
- Minetto MA, Caresio C, Menapace T, Hajdarevic A, Marchini A, Molinari F, Maffiuletti NA. Ultrasound-Based Detection of Low Muscle Mass for Diagnosis of Sarcopenia in Older Adults. PM R 2016;8:453-62. [Crossref] [PubMed]
- Neira Álvarez M, Vázquez Ronda MA, Soler Rangel L, Thuissard-Vasallo IJ, Andreu-Vazquez C, Martinez Martin P, Rábago Lorite I, Serralta San Martín G. Muscle Assessment by Ultrasonography: Agreement with Dual-Energy X-Ray Absorptiometry (DXA) and Relationship with Physical Performance. J Nutr Health Aging 2021;25:956-63. [Crossref] [PubMed]
- Rankin G, Stokes M, Newham DJ. Abdominal muscle size and symmetry in normal subjects. Muscle Nerve 2006;34:320-6. [Crossref] [PubMed]
- Rustani K, Kundisova L, Capecchi PL, Nante N, Bicchi M. Ultrasound measurement of rectus femoris muscle thickness as a quick screening test for sarcopenia assessment. Arch Gerontol Geriatr 2019;83:151-4. [Crossref] [PubMed]
- Sari A, Esme M, Aycicek GS, Armagan B, Kilic L, Ertenli AI, Halil MG, Akdogan A. Evaluating skeletal muscle mass with ultrasound in patients with systemic sclerosis. Nutrition 2021;84:110999. [Crossref] [PubMed]
- Stokes M, Rankin G, Newham DJ. Ultrasound imaging of lumbar multifidus muscle: normal reference ranges for measurements and practical guidance on the technique. Man Ther 2005;10:116-26. [Crossref] [PubMed]
- Wilkinson TJ, Gore EF, Vadaszy N, Nixon DGD, Watson EL, Smith AC. Utility of Ultrasound as a Valid and Accurate Diagnostic Tool for Sarcopenia: Sex-Specific Cutoff Values in Chronic Kidney Disease. J Ultrasound Med 2021;40:457-67. [Crossref] [PubMed]
- Wilson DV, Moorey H, Stringer H, Sahbudin I, Filer A, Lord JM, Sapey E. Bilateral Anterior Thigh Thickness: A New Diagnostic Tool for the Identification of Low Muscle Mass? J Am Med Dir Assoc 2019;20:1247-1253.e2. [Crossref] [PubMed]
- Abraham A, Drory VE, Fainmesser Y, Lovblom LE, Bril V. Quantitative sonographic evaluation of muscle thickness and fasciculation prevalence in healthy subjects. Muscle Nerve 2020;61:234-8. [Crossref] [PubMed]
- Kuyumcu ME, Halil M, Kara Ö, Çuni B, Çağlayan G, Güven S, Yeşil Y, Arık G, Yavuz BB, Cankurtaran M, Özçakar L. Ultrasonographic evaluation of the calf muscle mass and architecture in elderly patients with and without sarcopenia. Arch Gerontol Geriatr 2016;65:218-24. [Crossref] [PubMed]
- Rankin G, Stokes M, Newham DJ. Size and shape of the posterior neck muscles measured by ultrasound imaging: normal values in males and females of different ages. Man Ther 2005;10:108-15. [Crossref] [PubMed]
- Sengul Aycicek G, Ozsurekci C, Caliskan H, Kizilarslanoglu MC, Tuna Dogrul R, Balci C, Unsal P, Esme M, Yavuz BB, Cankurtaran M, Halil MG. Ultrasonography versus bioelectrical impedance analysis: which predicts muscle strength better? Acta Clin Belg 2021;76:204-8. [Crossref] [PubMed]
- Abe T, Bell ZW, Wong V, Spitz RW, Yamada Y, Song JS, Loenneke JP. Skeletal muscle size distribution in large-sized male and female athletes. Am J Hum Biol 2021;33:e23473. [Crossref] [PubMed]
- Stock MS, Oranchuk DJ, Burton AM, Phan DC. Age-, sex-, and region-specific differences in skeletal muscle size and quality. Appl Physiol Nutr Metab 2020;45:1253-60. [Crossref] [PubMed]
- Fischer A, Hertwig A, Hahn R, Anwar M, Siebenrock T, Pesta M, Liebau K, Timmermann I, Brugger J, Posch M, Ringl H, Tamandl D, Hiesmayr MUSVALID Collaboration Group. Validation of bedside ultrasound to predict lumbar muscle area in the computed tomography in 200 non-critically ill patients: The USVALID prospective study. Clin Nutr 2022;41:829-37. [Crossref] [PubMed]
- Kanehisa H, Ikegawa S, Fukunaga T. Comparison of muscle cross-sectional area and strength between untrained women and men. Eur J Appl Physiol Occup Physiol 1994;68:148-54. [Crossref] [PubMed]
- Perkisas S, Baudry S, Bauer J, Beckwée D, De Cock AM, Hobbelen H, Jager-Wittenaar H, Kasiukiewicz A, Landi F, Marco E, Merello A, Piotrowicz K, Sanchez E, Sanchez-Rodriguez D, Scafoglieri A, Cruz-Jentoft A, Vandewoude M. Application of ultrasound for muscle assessment in sarcopenia: towards standardized measurements. Eur Geriatr Med 2018;9:739-57. [Crossref] [PubMed]
- Distefano G, Goodpaster BH. Effects of Exercise and Aging on Skeletal Muscle. Cold Spring Harb Perspect Med 2018;8:a029785. [Crossref] [PubMed]
- Park SY, Yoon KH, Hwang SH, Ko TS, Lee HS. Aging-related changes in knee flexor muscle strength and cross-sectional area. Medicine (Baltimore) 2022;101:e31104. [Crossref] [PubMed]
- Fujiwara K, Asai H, Toyama H, Kunita K, Yaguchi C, Kiyota N, Tomita H, Jacobs JV. Changes in muscle thickness of gastrocnemius and soleus associated with age and sex. Aging Clin Exp Res 2010;22:24-30. [Crossref] [PubMed]
- Usgu S, Ramazanoğlu E, Yakut Y. The Relation of Body Mass Index to Muscular Viscoelastic Properties in Normal and Overweight Individuals. Medicina (Kaunas) 2021.
- Al-Qahtani M, Altuwaijri O, Altaf M, Al-Enezi M, Abulmeaty M, Javed R. Influence of body mass index and weight lifting on bicep brachii muscle and distal bicep tendon stiffness evaluated using ultrasound elastography. BMC Med Imaging 2020;20:129. [Crossref] [PubMed]
- Koda H, Kai Y, Murata S, Osugi H, Anami K, Fukumoto T, Imagita H. Relationship Between Muscle Strength Asymmetry and Body Sway in Older Adults. J Aging Phys Act 2018;26:457-61. [Crossref] [PubMed]
- Van den Broeck J, Héréus S, Cattrysse E, Raeymaekers H, De Maeseneer M, Scafoglieri A. Reliability of Muscle Quantity and Quality Measured With Extended-Field-of-View Ultrasound at Nine Body Sites. Ultrasound Med Biol 2023;49:1544-9. [Crossref] [PubMed]
- Girts RM, Harmon KK, Pagan JI, Alberto A, Hernandez MG, Stock MS. The influence of ultrasound image depth and gain on skeletal muscle echo intensity. Appl Physiol Nutr Metab 2022;47:839-46. [Crossref] [PubMed]
- Varanoske AN, Coker NA, Johnson BD, Belity T, Wells AJ. Influence of muscle depth and thickness on ultrasound echo intensity of the vastus lateralis. Acta Radiol 2021;62:1178-87. [Crossref] [PubMed]
- Scafoglieri A, Van den Broeck J, Bartocci P, Cattrysse E, Jager-Wittenaar H, Gonzalez MC. Skeletal Muscle Echo Intensity Values Differ Significantly across Ultrasound Parameter Settings. Life (Basel) 2024;14:291. [Crossref] [PubMed]
- Jensen B, Braun W, Geisler C, Both M, Klückmann K, Müller MJ, Bosy-Westphal A. Limitations of Fat-Free Mass for the Assessment of Muscle Mass in Obesity. Obes Facts 2019;12:307-15. [Crossref] [PubMed]
- Walowski CO, Braun W, Maisch MJ, Jensen B, Peine S, Norman K, Müller MJ, Bosy-Westphal A. Reference Values for Skeletal Muscle Mass - Current Concepts and Methodological Considerations. Nutrients 2020;12:755. [Crossref] [PubMed]
- Guo M, Diaz-Canestro C, Pugliese NR, Paneni F, Montero D. Lean body mass and the cardiorespiratory phenotype: An ethnic-specific relationship in Hans Chinese women and men. J Cachexia Sarcopenia Muscle 2024;15:963-74. [Crossref] [PubMed]