Contrast-enhanced ultrasound for the prediction of intraoperative blood loss in patients undergoing resection for carotid body paraganglioma
Introduction
Carotid body paraganglioma (CBP) is a rare tumor that originates from neural nonchromaffin cells. It is typically localized near the carotid bifurcation and is characterized by a rich vascular network within the lesion and a capsule supplied by many feeding arteries (1). Surgical removal of the tumor is the most appropriate treatment. However, the related complications, including intraoperative blood loss (IBL), cannot be ignored due to the proximity to the extracranial arterial structures, cranial nerves, and the complex anatomy of head and neck region.
Conventional ultrasound (US) is a critical means to identifying CBP and, compared to computed tomography (CT) and magnetic resonance imaging (MRI), is more widely available, simpler, and more cost-effective; however, its sensitivity in detecting CBP blood flow is poor. Contrast-enhanced US (CEUS) can display the nuances of blood flow signal inside the tumor, thus enabling a more detailed analysis of the structure and blood supply source inside the tumor. Consequently, CEUS has been used in clinical practice as an additional diagnostic and differential diagnostic tool for the evaluation of the perfusion of different pathologies. In cases of CBP, CEUS can more ably discern the Shamblin type and vascularity and can better visualize the origins of feeding vessels than can color Doppler US (2). In the CEUS mode, CBPs display a fast wash-in and slow wash-out pattern, with a high area under the curve (AUC) in the time-intensity curve (TIC) analysis (2).
Previous studies have demonstrated that a higher Shamblin grade and tumor volume and a shorter distance of the tumor from the skull base are associated with IBL and cranial nerve injury in patients undergoing CBP resection (3-6). However, it remains unknown whether CEUS enhancement pattern and quantitative perfusion parameters are related to IBL in CBP resection.
The purpose of our study was to evaluate the potential of the CEUS enhancement pattern and quantitative perfusion parameters as imaging biomarkers for predicting IBL in CBP resection. We present this article in accordance with the STROBE reporting checklist (available at https://qims.amegroups.com/article/view/10.21037/qims-24-832/rc).
Methods
Study population
This study was conducted in accordance with the Declaration of Helsinki (as revised in 2013) and was approved by Ethics Committee of Qinghai University Affiliated Hospital (No. P-SL-20190057). Informed consent was obtained from all individual participants. From November 2020 to December 2021, we recruited 19 patients and formed a multidisciplinary treatment group to carry out centralized diagnosis, treatment, and follow-up for these patients. A total of ten consecutive patients who fulfilled the inclusion criteria were enrolled in this study. The inclusion criteria included all those with surgically confirmed CBP who had undergone preoperative CT angiography or digital subtraction angiography and CEUS scans of the neck at Qinghai University Affiliated Hospital. Patients with preoperative embolization or allergy to contrast media were excluded. Demographic information including age and gender, along with Shamblin classification (i.e., type I, type II, or type III), was collected.
IBL estimation
IBL was estimated by the attending surgeon after consultation with the staff anesthesiologist. It was calculated as the sum of blood volume in suction bottles (minus the amount of heparin water flushing fluid) and the blood-soaked gauze (minus their dry weight and divided by blood density).
CEUS
All US examinations were completed by an experienced radiologist (with 20 years of clinical experience in CEUS) who was blinded to the patients’ clinical data and performed with an Acuson S2000 US system (Siemens Healthineers, Erlangen, Germany) with 7.5- to 12-MHz 9L4 linear transducer, equipped with contrast pulse sequencing imaging software.
First, each patient underwent a complete baseline two-dimensional and color Doppler US examination of the CBP and the adjacent tissues. The maximum diameter of the CBP (Dmax) and the blood flow of the CBP were documented.
Second, contrast mode was activated after the optimal cross-section of the target lesion and the adjacent common carotid artery (CCA) was selected. Timing was initiated when the sulfur hexafluoride microbubble US contrast agent (SonoVue, Bracco, Milan, Italy) was injected intravenously as a 2.4-mL bolus, which was followed by a 5-mL normal sterile saline flush with a 20-gauge peripheral intravenous cannula. The patient was observed continuously for 5 minutes, and dynamic images were recorded for subsequent analysis.
Third, the following CEUS features were recorded: (I) the enhancement level of the CBP (hypo-, iso- or hyperenhancement) and (II) the enhancement pattern of the CBP compared with the surrounding normal tissues (homogeneous, heterogeneous without perfusion, and heterogeneous with perfusion, rated 1, 2, and 3, respectively) (Figure 1).
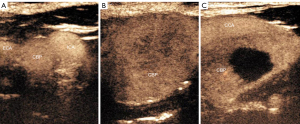
Finally, the region of interest (ROI) was placed at the solid part of the tumor and the adjacent CCA, and the TIC of the ROI was generated. The following quantitative perfusion parameters of CBP were recorded: peak intensity (PI), defined as the maximal signal intensity measured in the selected ROI (the PI of peripheral CBP or CCA was defined as 100%); time to peak (TP), defined as the time from the starting point to the PI of the curve; and AUC, defined as the area under the whole TIC; mean transit time (MTT; in seconds), defined as the time from the starting point to a PI drop of 50% of the curve. All parameters were measured three times and averaged.
Statistical analysis
Continuous data with a normal distribution are presented as the mean ± standard deviation (SD) and were analyzed with the independent samples t-test. Nonnormally distributed variables are reported as the median and interquartile range (IQR) and were analyzed with the Mann-Whitney test. Categorical variables are represented as frequencies and percentages and were analyzed with the Fisher exact test. Two models were constructed to predict IBL via stepwise multiple linear regression analysis. Model 1 was generated from basic characteristics including age, gender, location, Dmax, Adler grade, CBP Shamblin type, while Model 2 was generated from the CEUS enhancement pattern (homogeneous, heterogeneous without perfusion, and heterogeneous with perfusion) and quantitative perfusion parameters (PI, TP, AUC, and MTT). The predictive ability of these two models was assessed using the area under the receiver operating characteristic (ROC). P values <0.05 were considered to indicate statistical significance. All statistical analyses were processed using MedCalc version 16.8.4 (MedCalc Software, Ostend, Belgium) and SPSS version 19.0 (IBM Corp., Armonk, NY, USA) statistical software.
Results
Characteristics of included patients
A total of 10 patients with CBP were included in this study. The median age of the 10 patients was 57 (IQR, 51–66) years, and 8 were female. According to Shamblin classification, 3 tumors were type I, 4 were type II, and the remaining 3 were type III. The IBL ranged from 10 to 800 mL, with a median of 100 (IQR, 20–400) mL, and the samples were thus placed into the following groups according to the median IBL (100 mL): a <100 mL group and a ≥100 mL group. We also adopted two other grouping methods based on the actual IBL of the sample: a grouping of <50 and ≥50 mL and a grouping of <400 mL group and ≥400 mL. CBPs in the ≥50 mL group, ≥100 mL group, and ≥400 mL group were significantly larger than those in the <50 mL group, <100 mL group, and <400 mL group (P<0.05), respectively. Shamblin type I CBPs accounted for a higher proportion in the <50 mL group and the <100 mL group (P<0.05). Grade 1 CEUS enhancement pattern was more common in the <100 mL group, while grade 3 CEUS enhancement pattern was more common in the ≥100 mL group (P<0.05). A detailed overview of the basic characteristics and CBP CEUS parameters are provided in Table 1.
Table 1
Variables | Minor IBL | Moderate IBL | Major IBL | ||||||||
---|---|---|---|---|---|---|---|---|---|---|---|
<50 mL (n=3) | ≥50 mL (n=7) | P value | <100 mL (n=4) | ≥100 mL (n=6) | P value | <400 mL (n=7) | ≥400 mL (n=3) | P value | |||
Age (years) | 60 | 52 (51–64.5) | 0.36 | 58.50 (54.00–63.00) | 54.50 (51.00–67.00) | 0.831 | 57 (51–64.5) | 57 | 0.65 | ||
Female | 2 (66.70) | 6 (85.71) | 0.53 | 3 (75.00) | 5 (83.33) | >0.99 | 5 (71.43) | 3 (100.00) | >0.99 | ||
Left | 3 (100.00) | 3 (42.86) | 0.20 | 4 (100.00) | 2 (33.33) | 0.076 | 5 (71.43) | 1 (33.33) | 0.500 | ||
Right | 0 (0.00) | 4 (57.14) | 0.20 | 0 (0.00) | 4 (66.67) | 0.076 | 2 (28.57) | 2 (66.67) | 0.500 | ||
Blood loss (mL) | 20 | 150.00 (100.00–550.00) |
0.017 | 20.00 (15.00–35.00) |
275.00 (100.00–600.00) |
0.011 | 50.00 (20.00–100.00) |
600 | 0.017 | ||
Shamblin type I | 3 (100.00) | 0 (0.00) | 0.008 | 3 (75.00) | 0 (0.00) | 0.033 | 3 (42.86) | 0 (0.00) | 0.475 | ||
Shamblin type II | 0 (0.00) | 4 (57.14) | 0.20 | 1 (25.00) | 3 (50.00) | 0.571 | 3 (42.86) | 1 (33.33) | >0.99 | ||
Shamblin type III | 0 (0.00) | 3 (42.86) | 0.475 | 0 (0.00) | 3 (50.00) | 0.200 | 1 (14.28) | 2 (66.67) | 0.523 | ||
Dmax (mm) | 11.25 (10.40–16.95) |
39.35 (36.50–41.20) |
0.019 | 10.5 | 38.70 (29.60–40.90) |
0.017 | 23.60 (10.88–34.20) |
41.2 | 0.033 | ||
CEUS enhancement pattern | |||||||||||
1 | 2 (66.67) | 1 (16.67) | 0.183 | 3 (0.75) | 0 (0.00) | 0.024 | 3 (42.86) | 0 (0.00) | 0.475 | ||
2 | 1 (33.33) | 1 (14.29) | 0.533 | 1 (0.25) | 1 (16.67) | >0.99 | 2 (28.57) | 0 (0.00) | >0.99 | ||
3 | 0 (0.00) | 5 (71.43) | 0.167 | 0 (0.00) | 5 (83.33) | 0.048 | 2 (28.57) | 3 (100.00) | 0.167 | ||
Peak intensityCBP (%) | 26.86 | 29.63 (28.12–40.31) |
0.732 | 28.25 (25.55–41.57) |
34.07 (28.03–44.50) |
0.831 | 29.63 (24.89–44.50) |
28.4 | 0.909 | ||
TPCBP (s) | 35.5 | 41.34 (34.31–46.37) |
0.833 | 41.20 (29.48–65.38) |
41.06 (32.16–44.76) |
0.914 | 41.34 (32.99–46.37) |
40.77 | >0.99 | ||
AUCCBP (%s) | 2,553.03 | 3,173.40 (1,869.17–5,283.36) |
0.517 | 2,648.65 (1,928.77–3,443.90) |
2,640.30 (1,789.83–5,663.30) |
0.914 | 2,744.27 (1,616.63–5,283.36) |
2107.2 | 0.667 | ||
MTTCBP (s) | 72.78 | 87.24 (47.73–94.71) |
>0.99 | 86.88 (50.11–103.12) |
73.46 (43.75–94.12) |
0.610 | 94.12 (48.14–99.47) |
59.67 | 0.517 | ||
Peak intensityCCA (%) | 49.05 | 38.55 (34.86–46.93) | 0.183 | 45.65 (37.18–61.75) | 40.45 (37.15–48.45) | 0.762 | 48.45 (36.14–53.63) | 38.55 | 0.517 | ||
TPCCA (s) | 29.89 | 34.25 (29.89–40.50) |
0.833 | 35.63 (26.36–46.83) |
32.29 (29.74–40.14) |
0.762 | 40.14 (27.81–41.17) |
30.33 | 0.667 | ||
AUCCCA (%s) | 3,201.7 | 2,728.40 (1,872.10–5,406.84) |
>0.99 | 3,316.43 (2,644.18–4,290.10) |
2,464.43 (1,762.65–6,065.40) |
0.762 | 3,431.15 (2,365.41–5,836.31) |
2,200.45 | 0.267 | ||
MTTCCA (s) | 50.42 | 55.29 (41.65–88.60) |
0.833 | 65.97 (39.26–87.29) |
49.43 (41.01–90.96) |
>0.99 | 81.52 (40.79–92.54) |
43.57 | 0.517 | ||
Ratio of CBP PI to CCA PI | 0.57 | 0.81 (0.74–0.92) | 0.117 | 0.65 (0.56–0.82) | 0.78 (0.74–0.92) | 0.476 | 0.72 (0.55–0.89) | 0.75 | 0.383 | ||
Ratio of CBP TP to CCA TP | 1.19 | 1.13 (1.06–1.19) | 0.833 | 1.16 (1.08–1.40) | 1.15 (1.05–1.19) | 0.914 | 1.13 (1.05–1.19) | 1.19 | 0.383 | ||
Ratio of CBP AUC to CCA AUC | 0.63 | 0.81 (0.71–1.14) | 0.383 | 0.74 (0.56–1.03) | 0.79 (0.69–0.93) | 0.91 | 0.69 (0.53–0.91) | 0.81 | 0.383 | ||
Ratio of CBP MTT to CCA MTT | 1.13 | 1.06 (1.01–1.20) | 0.833 | 1.18 (1.05–1.34) | 1.05 (1.00–1.08) | 0.476 | 1.06 (0.99–1.21) | 1.08 | 0.667 |
Data are expressed as median (interquartile range), n (%) or median. AUC, area under the curve; CBP, carotid body paraganglioma; CCA, common carotid artery; CEUS, contrast-enhanced ultrasound; Dmax, the maximum diameter of carotid body paraganglioma; IBL, intraoperative blood loss; MTT, mean transit time; PI, peak intensity; TP, time to peak.
Multiple linear regression analysis
As shown in Table 2, neither the basic characteristics nor CEUS enhancement pattern and quantitative perfusion parameters showed statistically significant correlations with IBL in the multiple regression analysis (P>0.05). However, Dmax and CEUS enhancement pattern both exhibited statistically significant correlations with IBL (P<0.05) in the stepwise multiple regression analysis. The regression equation of Model 1 was y = 14.735 × –211.314 (R=0.818; R2=0.669; R2-adjusted =0.629; F=16.232; P=0.004), where x and y are the Dmax (mm) and IBL (mL), respectively. The regression equation of Model 2 was y = 200 × –215 (R=0.659; R2=0.434; R2-adjusted =0.364; F=6.147; P=0.038), where x and y are the enhancement pattern of CBP (homogeneous, heterogeneous without perfusion, or heterogeneous with perfusion; i.e., grade 1, 2, or 3, respectively) and IBL (mL), respectively.
Table 2
Independent variable | Enter method | Stepwise method | |||||||||||
---|---|---|---|---|---|---|---|---|---|---|---|---|---|
Unstandardized coefficients | Standardized coefficients | t | Sig | Unstandardized coefficients | Standardized coefficients | t | Sig | ||||||
B | Std. error | Beta | B | Std. error | Beta | ||||||||
Model 1 | |||||||||||||
(Constant) | −1,127.153 | 1,335.403 | −0.844 | 0.416 | −211.314 | 120.894 | −1.748 | 0.119 | |||||
Age | 112.624 | 442.106 | 0.170 | 0.255 | 0.815 | ||||||||
Gender | 11.639 | 8.706 | 0.456 | 1.337 | 0.274 | ||||||||
Location | 118.965 | 280.052 | 0.220 | 0.425 | 0.700 | ||||||||
Dmax | 14.751 | 13.697 | 0.891 | 1.077 | 0.360 | 14.735 | 3.657 | 0.818 | 4.029 | 0.004 | |||
Adler grade | −14.151 | 455.609 | −0.035 | −0.031 | 0.977 | ||||||||
Shamblin type | 101.828 | 217.691 | 0.298 | 0.468 | 0.672 | ||||||||
Model 2 | |||||||||||||
(Constant) | −12,110.276 | 0.000 | −215.000 | 190.896 | −1.126 | 0.293 | |||||||
CEUS enhancement pattern | 658.596 | 0.000 | 2.171 | 200.000 | 80.668 | 0.659 | 2.479 | 0.038 | |||||
Peak intensityCBP | |||||||||||||
TPCBP | −132.884 | 0.000 | −7.785 | ||||||||||
AUCCBP | 0.137 | 0.000 | 0.860 | ||||||||||
MTTCBP | |||||||||||||
Peak intensityCCA | 57.841 | 0.000 | 2.588 | ||||||||||
TPCCA | 339.065 | 0.000 | 10.602 | ||||||||||
AUCCCA | −0.585 | 0.000 | −4.707 | ||||||||||
MTTCCA | |||||||||||||
Ratio of CBP PI to CCA PI | 1,500.126 | 0.000 | 0.867 | ||||||||||
Ratio of CBP TP to CCA TP | −3,030.020 | 0.000 | −4.260 | ||||||||||
Ratio of CBP AUC to CCA AUC | |||||||||||||
Ratio of CBP MTT to CCA MTT | 4,495.13 | 0.000 | 5.872 |
Model 1 was y = 14.735 x –211.314, where x and y are the Dmax (mm) and IBL (mL), respectively. Model 2 was y = 200 x –215, where x and y are the enhancement pattern of CBP (homogeneous, heterogeneous without perfusion, or heterogeneous with perfusion; i.e., grade 1, 2, or 3, respectively) and IBL (mL), respectively. AUC, area under the curve; CBP, carotid body paraganglioma; CCA, common carotid artery; CEUS, contrast-enhanced ultrasound; Dmax, maximum diameter of carotid body paraganglioma; IBL, intraoperative blood loss; MTT, mean transit time; PI, peak intensity; Sig, significance; Std., standard; TP, time to peak.
ROC curve analysis
As shown in Table 3, Model 1 was more powerful than Model 2 in predicting a IBL ≥50 and >400 mL, while Model 2 and Model 1 demonstrated comparable strength in predicting IBL ≥100 mL.
Table 3
Variable | AUC (95% CI) | z statistic | P value | Cutoff | Sensitivity (%) | Specificity (%) | Youden index |
---|---|---|---|---|---|---|---|
Model 1 | |||||||
≥50 mL | 1.000 (0.692–1.000) | – | <0.0001 | 10.52 | 100.00 | 100.00 | 1.000 |
≥100 mL | 0.958 (0.630–1.000) | 7.778 | <0.0001 | 163.45 | 83.33 | 100.00 | 0.833 |
≥400 mL | 0.952 (0.622–1.000) | 6.718 | <0.0001 | 205.69 | 100.00 | 85.71 | 0.857 |
Model 2 | |||||||
≥50 mL | 0.881 (0.532–0.995) | 3.608 | 0.0003 | 185 | 71.43 | 100.00 | 0.714 |
≥100 mL | 0.979 (0.660–1.000) | 16.263 | <0.0001 | 185 | 83.33 | 100.00 | 0.833 |
≥400 mL | 0.857 (0.505–0.991) | 3.873 | 0.0001 | 185 | 100.00 | 71.43 | 0.714 |
Model 1 was y = 14.735 x –211.314, where x and y are the Dmax (mm) and IBL (mL), respectively. Model 2 was y = 200 x –215, where x and y are the enhancement pattern of CBP (homogeneous, heterogeneous without perfusion, or heterogeneous with perfusion; i.e., grade 1, 2, or 3, respectively) and IBL (mL), respectively. AUC, area under the curve; CI, confidence interval; IBL, intraoperative blood loss.
Discussion
To our knowledge, this is the first study to evaluate the performance of CEUS in predicting IBL in CBP resection. The Dmax and CEUS enhancement pattern can be used as imaging biomarkers for predicting IBL in CBP resection.
The results of our study were in agreement with those of previous studies in which IBL was correlated with tumor size (volume), Shamblin classification, and distance to base of skull (DTBOS) (3-7). However, there are certainly other factors that affect IBL, such as coagulopathy, antiplatelet/anticoagulant usage, and the technical and coordination proficiency of surgeons. In general, the larger the tumor is, the greater the degree of encirclement of the carotid artery (and the higher the Shamblin classification). The dissection of the tumor from the closely adhered carotid artery will inevitably lead to an increase in intraoperative bleeding. In addition, the larger the tumor is, the closer the tumor is to the skull base (and the smaller the DTBOS), which will increase the difficulty of the operation and increase the amount of intraoperative bleeding.
The grade 1 CEUS enhancement pattern was more common in the <100 mL group, while the grade 3 CEUS enhancement pattern was more common in the ≥100 mL group (P<0.05), and therefore the CEUS enhancement pattern can be used to predict IBL ≥100 mL; however, we found that the CEUS enhancement pattern and quantitative perfusion parameters of CBPs in the ≥50 mL group and ≥400 mL group did not differ from those of the <50 mL group and <400 mL group, respectively, nor were they identified as independent predictors in multivariate regression analyses. This might be explained by the fact that the CEUS enhancement pattern and quantitative perfusion parameters mainly reflect the blood supply inside the tumor. The primary aim of CBP resection is to remove the tumor completely from the carotid artery along the edge of the tumor, with the site of bleeding mainly being the edge or where it adheres to the carotid artery. Perhaps examining the blood supply at the edge of CBP may help to predict the amount of IBL.
In this study, we used the CCA as the control due to its measurements being readily obtainable. In contrast to the CCA, CBPs have a slow wash-in and fast wash-out pattern with a low AUC in the TIC analysis. Theoretically, the ratio of CBP PI to CCA PI, the ratio of CBP TP to CCA TP, the ratio of CBP AUC to CCA AUC, and the ratio of CBP MTT to CCA MTT might constitute novel parameters for predicting IBL. However, our study failed to draw such a conclusion, possibly because these ratios still reflected the internal perfusion of CBP and could not differentiate between the <50 mL group and ≥50 mL group, <100 mL group and ≥100 mL group, or between the <400 mL group and ≥400 mL group.
Our study was inevitably affected by two factors. First, the sample size was small, and thus deviation in the statistical results could not be avoided. Studies based on larger populations would be more persuasive. Second, there was variability in the IBL measurement, and it was necessary to combine multiple calculation methods, such as the volumetric method, weighing method, and hemoglobin method.
Conclusions
This study assessed the value of CEUS in predicting IBL in CBP resection. Our results indicate that CEUS enhancement pattern can be used to predict IBL ≥100 mL and that the Dmax can be used to predict IBL ≥50, 100, or 400 mL. Despite its limitations, this study produced findings that may provide considerable clinical value for the evaluation of IBL and the formulation of treatment plans in patients with CBP.
Acknowledgments
None.
Footnote
Reporting Checklist: The authors have completed the STROBE reporting checklist. Available at https://qims.amegroups.com/article/view/10.21037/qims-24-832/rc
Funding: None.
Conflicts of Interest: All authors have completed the ICMJE uniform disclosure form (available at https://qims.amegroups.com/article/view/10.21037/qims-24-832/coif). The authors have no conflicts of interest to declare.
Ethical Statement: The authors are accountable for all aspects of the work in ensuring that questions related to the accuracy or integrity of any part of the work are appropriately investigated and resolved. This study was conducted in accordance with the Declaration of Helsinki (as revised in 2013) and was approved by Ethics Committee of Qinghai University Affiliated Hospital (No. P-SL-20190057). Informed consent was obtained from all participants.
Open Access Statement: This is an Open Access article distributed in accordance with the Creative Commons Attribution-NonCommercial-NoDerivs 4.0 International License (CC BY-NC-ND 4.0), which permits the non-commercial replication and distribution of the article with the strict proviso that no changes or edits are made and the original work is properly cited (including links to both the formal publication through the relevant DOI and the license). See: https://creativecommons.org/licenses/by-nc-nd/4.0/.
References
- Shamblin WR. ReMine WH, Sheps SG, Harrison EG Jr. Carotid body tumor (chemodectoma). Clinicopathologic analysis of ninety cases. Am J Surg 1971;122:732-9. [PubMed]
- Gu G, Zhang X, Shen J, Gulidanna S, Gao Q, Shao J, Liu B, Zhang B, Zheng Y. Comparison of Contrast-Enhanced Ultrasonography to Color Doppler Ultrasound in Evaluation of Carotid Body Tumors. Front Oncol 2022;12:872890. [Crossref] [PubMed]
- Dardik A, Eisele DW, Williams GM, Perler BA. A contemporary assessment of carotid body tumor surgery. Vasc Endovascular Surg 2002;36:277-83. [PubMed]
- Kim GY, Lawrence PF, Moridzadeh RS, Zimmerman K, Munoz A, Luna-Ortiz K, et al. New predictors of complications in carotid body tumor resection. J Vasc Surg 2017;65:1673-9. [PubMed]
- Li ZZ, Liang Y, Zhang Y, Du J, Liu H, Ruan C, Wang Y, Gu G, Sun H, Chen Y, Jin Z. Value of head and neck CT angiography in the clinical evaluation of intraoperative bleeding volume of carotid body tumours. Acta Acad Med Sin 2020;42:491-6. [PubMed]
- Ghoddusi Johari H, Afshari A, Abolhasani Foroughi A, Khademi B, Shahriarirad S, Erfani A, Zeinali-Rafsanjani B, Saeedi-Moghadam M, Shahriarirad R. Association of Complications During and After Carotid Body Tumor Resection with Tumor Size and Distance to the Base of Skull. Ann Vasc Surg 2023;94:223-8. [Crossref] [PubMed]
- Cao Y, Bao H, Zhang G, Zhang J, Yan X, Wen S, Zhao G, Zhou J. Correlation between preoperative imaging features and operative complications of carotid body tumors. J Clin Radiology 2021;40:220-4.