Choroidal thinning can be assessed through facial video analysis
Introduction
Situated between the retina and the sclera, the choroidal perfusion provides essential nutrients to the retinal pigment epithelium and the outer retina (1) and allows for the dissipation of heat from light impinging on the fundus (2). Alterations in choroidal circulation have been associated with ocular health and visual functions (3,4). Studies investigating the relationship between choroidal volume and choroidal circulation suggest that choroidal thinning, defined as a reduction in choroidal thickness (CT) [CT <200 µm, the lowest value observed in normal populations (5-7)] may be a marker of a compromised choroidal circulation (8). Consequently, the evaluation of choroidal thinning has emerged as a critical biomarker (9-11), and the assessment of choroidal thinning has become an area of interest in the diagnosis and management of conditions like age-related macular degeneration (AMD) (12), myopia (13), and diabetic retinopathy (14). In such cases, changes in CT have been shown correlation with the progression of the disease and are thought to be prognostic indicators (12-15).
Conventional assessments of CT have relied heavily on ophthalmic imaging techniques, with optical coherence tomography (OCT) being the most valuable (7,16). While these techniques have proven invaluable within clinical context, their implementation requires specialized equipment, trained personnel, and time-intensive procedures. Moreover, their utility remains largely confined to controlled clinical environments, impeding their broader adoption for routine screening. Given the constraints imposed by existing assessment techniques, there is a need to create innovative tools that facilitate accessible, efficient, and widespread screening for choroidal thinning.
According to the angiosomes theory, specific anatomical regions within human tissues are supplied with blood through dedicated source arteries and accompanying veins (17,18). The skin, being the body’s largest organ, comprises a multitude of these angiosomes, intricately linked with the vascular network of the circulatory system (19,20). This forms the basis for evaluating vascular conditions by examining hemodynamics within the skin. Previous research has hinted at the potential of harnessing camera data streams from skin to predict vascular conditions (21,22). Integrating these advancements with the investigation of choroidal thinning may open the door to a transformative paradigm in the field.
This study uses facial video analysis to craft a tool for assessing choroidal thinning. Based on facial videos, we introduce the trans-angiosomes imaging photoplethysmography (TaiPPG) strategy; a method that computes regional facial blood pulsation to create regional markers capable of predicting CT (Figure 1). The findings provide compelling evidence that relative blood pulsation amplitude (BPA), derived from facial skin angiosomes and reflecting blood volume variations induced by the heart’s rhythmic pumping, may be used to predict the CT with notable accuracy. This validation attests to the efficacy of our analysis strategy and underscores its potential to enhance our ability to conveniently assess choroidal thinning. This study describes a method that could equip healthcare professionals with advanced tools that bypass traditional imaging methods to screen for patients at risk for disease progression. We present this article in accordance with the STROBE reporting checklist (available at https://qims.amegroups.com/article/view/10.21037/qims-24-2146/rc).
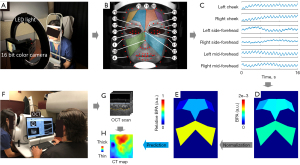
Methods
Study design and participants
The TaiPPG imaging method underwent clinical validation in a study that aimed to predict CT and detect choroidal thinning in patients with AMD. The study was conducted in accordance with the Declaration of Helsinki (as revised in 2013). The study was approved by Institutional Review Board of the University of Miami (No. 20201475) and informed consent was obtained from all individual participants. Facial videos were collected from the Bascom Palmer Eye Institute, University of Miami Miller School of Medicine, between 30 April 2021 and 10 August 2023. These videos were subsequently anonymized and de-identified to protect the privacy of the participants. The study included dry AMD, wet AMD, and normal cases, with exclusion criteria being uncorrectable global and local motion in the face, incomplete facial skin video coverage due to masks, hair, etc., overexposure of facial skin, and poor quality of OCT scanning. Throughout the data collection process, clinicians were masked to the technology used in video processing. The OCT and TaiPPG data were processed separately by different analysts, who were also masked to the data outside of their area of analysis.
Extraction of facial indicators
In the TaiPPG system, a 16-bit camera (Chronos 1.4; Kronos, Canada) was utilized to capture video images of reflections on the facial skin, which were produced by the illumination of a white ring light-emitting diode (LED) light source. A zoom lens (Computar 12.5-75 mm/f 1.2; CBC Group, Japan) was used to relay the facial image onto the camera sensor. To reduce specular reflections on the skin surface, we placed a pair of polarized films in front of the camera lens and light source at orthogonal orientations. The illumination uniformity is calculated as the ratio of U1 to U2, where U1 is the ratio of Minimum to Average lux, and U2 is the ratio of Minimum to Maximum lux. A uniformity value of 1 represents ideal lighting uniformity, with identical lux levels across all sampling points (23). In this study, they were measured at 0.99 and 0.95 respectively, indicating that the illumination was uniform across the facial skin. To prevent large motions, a chin rest was installed in front of the camera and light source to stabilize the patient’s head.
Forty-eight patients diagnosed with AMD (Dry: 33; Wet: 15) and 12 normal cases were included in this study, and TaiPPG and OCT angiographic (OCTA) imaging were used to follow them. Before recording the facial skin video in TaiPPG imaging, the subjects were asked to sit calmly in a chair for 30 seconds for heart rate stabilization. The room temperature and humidity were kept constant during the experiment. The video recording lasted for 16 seconds at 100 frames per second, with each frame having a resolution of 640 by 512 pixels in the R, G, and B channels. The acquired video was saved as 16-bit modes for further processing. To minimize motion artifacts, we applied a sub-pixel registration algorithm to co-register frames before extracting the pulsation signal (24,25). The green channel was selected for analysis since it had the highest signal-to-noise ratio (SNR) among the three channels.
We employed a well-known modified 81-point landmark detection algorithm to automatically identify facial landmarks (26). This is a custom shape predictor model trained to find 81 facial feature landmarks that have been widely used in facial recognition applications (27-29). Utilizing these landmarks, we delineated the cheek, side forehead, and central forehead regions, followed by the extraction of the pulse waveform. Each pulse waveform underwent a lock-in amplification algorithm to derive the BPA (30). Briefly, a global pulse wave was extracted from the video cube, followed by heart rate filtering to form a reference function. This generated reference function was then applied to other pulse waveforms to amplify the signal at the heart rate frequency and calculate their amplitudes. The resultant BPA map encapsulated the averaged magnitudes within the six outlined regions. To improve data presentation, we merged information from the left and right facial sides and normalized each region using overall face BPA values derived from the cheek and forehead regions. This process yielded three distinct groups of facial indicators: relative cheek BPA, relative side forehead BPA, and relative central forehead BPA.
CT measurements
The OCT imaging was performed using a SS-OCT system (PLEX Elite 9000; Carl Zeiss Meditec, CA, USA) with a laser source centered at 1,050 nm and a bandwidth of 100 nm. The system has an axial resolution of ~5 micrometers and a lateral resolution of approximately 20 µm in tissue. A 12 mm by 12 mm SS-OCT angiography (SS-OCTA) scan pattern centered on the fovea, consisting of 500 A-scans per B-scan and 500 B-scan positions with each B-scan position repeated twice, was used to image all subjects. From the three-dimensional scan volume, choroidal slabs were semi-automatically generated by segmenting the choroidal boundaries, including the Bruch’s membrane and choroid-sclera interface, using a previously reported algorithm (31,32). The en face CT map was then generated by measuring the distance between choroidal boundaries. The mean CT (MCT) value was obtained by averaging the CT map of both eyes (OD: right eye; OS: left eye).
Statistical analysis
Summary statistics are provided in the form of counts (percentages) and median (interquartile range) for categorical and continuous variables. To assess the relationships between CT and facial indicators (cheek, side forehead, and central forehead relative BPA), we utilized Pearson correlations. Subsequently, based on scatter plots, we constructed three linear regression models for predicting CT from facial indicators. Prior studies on normative databases indicate that the lowest value of the average CT thickness is around 200 µm among normal populations (5-7). Therefore by applying a threshold of 200 µm, we categorized 60 cases into choroidal thinning and non-choroidal thinning groups, enabling the calculation of prediction accuracy for the three models. Furthermore, we employed the area under the receiver operating characteristic curve (AUROC) to gauge model discrimination, presenting the AUROC along with its 95% confidence interval. All analyses were executed using Origin 2021b, and the significance level was set at an alpha of 0.05.
Results
Participants
A total of 60 participants were enrolled, comprising 36 (60%) women. The recruitment of patients was primarily focused on populations with AMD, owing to the proposed importance of reduced choroidal circulation in disease progression (3,33). Consequently, the study encompassed 33 subjects with dry AMD, 15 with wet AMD, and 12 exhibiting normal eyes (as presented in Tables 1,2). Each participant underwent both facial video recording and SS-OCT imaging. Multiple measurements were gathered from each AMD participant during successive follow-up visits. Normal cases underwent one measurement.
Table 1
Characteristics | Value (N=60) |
---|---|
Gender | |
Female | 36 (60%) |
Male | 24 (40%) |
Age range (years) | |
<60 | 22 (73%) |
60–79 | 24 (40%) |
80–89 | 14 (23%) |
Table 2
Characteristics | Dry AMD (n=33) | Wet AMD (n=15) | Normal (n=12) |
---|---|---|---|
Gender | |||
Female | 23 | 9 | 6 |
Male | 10 | 6 | 6 |
Age (years) | 76±10 | 77±6 | 67±10 |
SBP (mmHg) | 133±14 | 132±16 | 135±18 |
DBP (mmHg) | 72±7 | 70±8 | 75±8 |
Data are presented as number or mean ± standard deviation. AMD, age-related macular degeneration; SBP, systolic blood pressure; DBP, diastolic blood pressure.
Facial video indicators
To provide a visual depiction of the correlation between facial BPA and CT, we have chosen six illustrative cases and presented their outcomes in the following section. The visual representation in Figure 2A highlights facial BPA maps processed from the pixelated pulse waveform and distinct relative BPA values which is normalized by whole face across the cheek, side-forehead, and mid-forehead regions for these six individuals. A noticeable trend emerged from the leftmost to the rightmost cases, indicating a gradual decrease in BPA values in the forehead region. Figure 2B provides an insightful view of CT, revealing a discernible escalation in thickness as we progress from the left to the right across the subjects. This visual presentation underscores the potential relationship between facial BPA and CT, emphasizing the importance of considering regional variations in BPA values and their corresponding impact on CT. The selected cases serve as representative instances, shedding light on the nuanced patterns within facial BPA and CT, contributing to a deeper understanding of their interconnected dynamics.
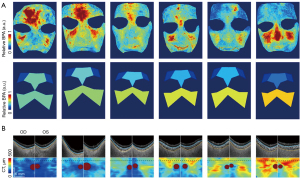
Relationship between video indicators and clinical measurements
We found significant correlations between the mean CT measured from SS-OCT scanning and automatic video-based facial indicators describing cheek BPA (r=0.48, P<0.001), side forehead BPA (r=−0.56, P<0.001), and central forehead BPA (r=−0.40, P<0.01) (Figure 3A-3C). It is worth noting that the outliers were detected and masked in the correlation analysis. This method uses regression analysis to identify outliers based on standardized residuals. After importing the data and creating a scatter plot, a linear fit is applied. The standardized residual is calculated and checked to spot any outliers (values beyond ±2). Identified outliers are masked using a tool, which automatically updates the regression analysis, excluding these points for more accurate results. It is worth noting that the interrelation among the three facial indicators is attributable to the way the BPA normalization denominator is calculated, which involves summing the BPA values from the cheek, side forehead, and central forehead areas. Consequently, the observed correlations between CT and the facial indicators could be the result of interactions between the choroid and one or two specific facial regions, rather than all three.
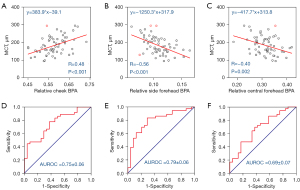
Based on the scatter plots, three linear regression models were developed to predict the CT. Although there is no established standard to define choroidal thinning in clinics, we used an artificial threshold to assess the performance of our models in classification. We separated the 60 cases into choroidal thinning and non-choroidal thinning groups using a cutoff at 200 µm as the reference. We then used the relative BPA values at this cutoff as the prediction threshold to classify these cases again. The resulting classification accuracy for the three models is 69.5% (relative cheek BPA, sensitivity: 88.9%, specificity: 39.1%), and 69.5% (relative side forehead BPA, sensitivity: 86.1%, specificity: 45.4%), and 66.9% (relative side forehead BPA, sensitivity: 91.7%, specificity: 26.1%), respectively. Receiver operating characteristic (ROC) curves for these three models are presented in Figure 3D-3F. The AUROC values were calculated to be 0.75±0.06, 0.79±0.06 and 0.69±0.07. These results suggest that video-based TaiPPG is capable of generating effective classification models for choroidal thinning.
Discussion
In this study, we introduced a method that utilized data streams acquired by a camera for the automated and objective evaluation of CT. Our approach involves the application of TaiPPG technology to detect relative BPA in specific facial regions, namely the cheek, side-forehead, and central forehead. Notably, this methodology achieved a commendable 65–70% accuracy in distinguishing choroidal thinning, positioning it as a promising screening strategy for recommending additional imaging technologies like OCT. What sets this approach apart is its potential application not only in the context of the assessment of choroidal thinning but also in the context of various ocular diseases. The utilization of video-based assessment not only enhances diagnostic capabilities but also offers the advantage of reducing the economic and physical burden on patients and healthcare systems during health monitoring. One of the key strengths lies in the ease of obtaining video recordings, a process that can be seamlessly executed by an operator without imaging expertise. This characteristic opens up the possibility of scaling up the method to cater to a larger population. The practicality of video-based assessment makes it particularly well-suited for integration into mobile and digital health applications, positioning it as a convenient and feasible diagnostic strategy for widespread use.
While the vascular explanation for this hemodynamic connection between the choroid and facial skin is not yet fully elucidated, we hypothesize that collateral circulation from the external carotid arteries (ECAs) might underlie this connection, similar to observations in cerebral studies where internal carotid stenosis induces increased blood supply in ECAs to compensate for cerebral tissue ischemia (34,35). As shown in Figure 4, within the context of our study, we suggest that there may be an abnormal chronic reduction of blood supply in the arteries downstream along the ophthalmic artery (OA), perhaps due to stenoses in the OA or carotid arteries, triggering a supplementary response that supports ocular tissue through the collateral circulation from the ECAs (32,36,37). This is specifically mediated by the anastomoses between the downstream OA branches and their ECA branches, such as the dorsal nasal arteries (DNA) connecting to the angular termination of the facial artery (FA) and infraorbital arteries (38). Consequently, as a result of decreased terminal OA perfusion to the forehead or cheek, there may be increased blood flow in the FA leading to heightened cheek blood pulsation, which would establish retrograde blood flow into the OA and a positive relationship between CT and relative cheek BPA. Conversely, if retrograde blood flow couldn’t be established in the OA, then the BPA of the side and central forehead exhibit a negative correlation with CT suggesting that the decreased blood flow from the OA to the forehead results in compensatory blood flow from the ECA. Specifically, a pronounced negative correlation between the side-forehead BPA and CT may be due to a relative increase to the forehead from the superficial temporal artery (STA) branches to compensate for the reduced perfusion from the OA to this same BPA, though this requires further investigation and substantiation.
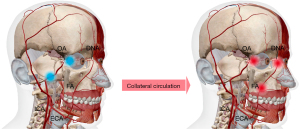
Our study introduces TaiPPG as a potential supplementary modality to conventional vascular imaging techniques. PPG operates indirectly, without producing angiography, making it challenging to predict the specific location and scale of abnormalities. Consequently, it should not be solely relied upon for clinical diagnosis. However, like PPG, TaiPPG excels in monitoring early disease stages before symptoms are manifested, a realm often inaccessible to conventional vascular imaging due to resource and expertise requirements (39-41). TaiPPG offers ubiquitous and frequent monitoring (42), facilitating early screening and risk detection (41,43,44). Moreover, the TaiPPG-based prediction mechanism developed here expands the capabilities of existing PPG and mobile health techniques. While these methods primarily focus on global hemodynamic indicators, angiosome-resolved monitoring introduces additional data dimensions, enabling the extraction of parameters related to symmetry, evenness, phase differences, and relative intensities for the prediction of specific vascular conditions (45,46).
Nonetheless, our study does present certain limitations, which offer opportunities for future research endeavors. Firstly, the underlying mechanism of our observations remains speculative. While we have proposed a hypothesis in our discussion, additional validation with robust evidence is imperative. One promising avenue is the ultrasound imaging, which can assess blood flow in the FA and even detect reverse flow in OA branches, shedding further the light on our assumptions (47,48). Moreover, studying the relationship between retinal characteristics and facial skin could provide valuable insights since OA perfusion may also affect retinal perfusion (49). Thus, a future study focusing on the retina or the entire fundus would be highly valuable. Additionally, TaiPPG technology faces challenges when applied to darker skin tones due to increased melanin absorption, which may reduce the signal-to-noise ratio and affect measurement accuracy. These limitations, however, may be mitigated by improving imaging sensor sensitivity and using multi-wavelength approaches to account for differences in skin tone. Furthermore, the TaiPPG system used in our study involved an advanced camera with a high bit depth, resulting in relatively higher costs and computational demands compared to the current internet of medical things systems. To ensure wider accessibility, it will be crucial to optimize processing steps for deployment in low-resource settings. Another limitation is the lack of a universally established threshold for defining choroidal thinning. In this study, we adopt a CT of less than 200 µm as the diagnostic threshold, based on findings from our previous research and related studies. Notably, testing alternative thresholds did not impact the overall conclusions of this work. Finally, the relatively small number of normal controls enrolled in this study limits the generalizability of our findings. Future work will focus on recruiting a larger cohort of controls to further validate our findings and strengthen the statistical robustness of the study.
Conclusions
In conclusion, we demonstrate that a facial imaging approach can quantitatively assess CT, offering significant clinical and public health implications for ocular health. These findings lay the scientific groundwork for mobile and digital health initiatives, allowing for the tracking of these traits in the general population without the need for extensive medical imaging.
Acknowledgments
None.
Footnote
Reporting Checklist: The authors have completed the STROBE reporting checklist. Available at https://qims.amegroups.com/article/view/10.21037/qims-24-2146/rc
Funding: This research was supported in part by an unrestricted grant from
Conflicts of Interest: All authors have completed the ICMJE uniform disclosure form (available at https://qims.amegroups.com/article/view/10.21037/qims-24-2146/coif). R.K.W. serves as a Deputy Editor of Quantitative Imaging in Medicine and Surgery. R.K.W. discloses intellectual property owned by the Oregon Health and Science University and the University of Washington. R.K.W. also receives research support from Research to Prevent Blindness, Inc., Carl Zeiss Meditec Inc., Colgate Palmolive Company, and Estee Lauder Inc. He is a consultant to Carl Zeiss Meditec and Cyberdontics. G.G. receives research support from Carl Zeiss Meditec, Inc. He and the University of Miami co-owned a patent that is licensed to Carl Zeiss Meditec, Inc. P.J.R. received research support from Research to Prevent Blindness, Inc., Carl Zeiss Meditec, Inc., and Gyroscope Therapeutics. P.J.R. and the University of Miami co-own a patent that is licensed to Carl Zeiss Meditec, Inc. P.J.R. is also a consultant for Abbvie, Annexon, Apellis, Boehringer-Ingelheim, Carl Zeiss Meditec, Chengdu Kanghong Biotech, Genentech/Roche, InflammX Therapeutics, Ocudyne, Regeneron Pharmaceuticals, and Unity Biotechnology. He also has equity interest in Apellis, InflammX, Ocudyne, and Valitor. The other authors have no conflicts of interest to declare.
Ethical Statement: The authors are accountable for all aspects of the work in ensuring that questions related to the accuracy or integrity of any part of the work are appropriately investigated and resolved. The study was conducted in accordance with the Declaration of Helsinki (as revised in 2013). The study was approved by Institutional Review Board of the University of Miami (No. 20201475) and informed consent was obtained from all individual participants.
Open Access Statement: This is an Open Access article distributed in accordance with the Creative Commons Attribution-NonCommercial-NoDerivs 4.0 International License (CC BY-NC-ND 4.0), which permits the non-commercial replication and distribution of the article with the strict proviso that no changes or edits are made and the original work is properly cited (including links to both the formal publication through the relevant DOI and the license). See: https://creativecommons.org/licenses/by-nc-nd/4.0/.
References
- Nickla DL, Wallman J. The multifunctional choroid. Prog Retin Eye Res 2010;29:144-68. [Crossref] [PubMed]
- Parver LM, Auker C, Carpenter DO. Choroidal blood flow as a heat dissipating mechanism in the macula. Am J Ophthalmol 1980;89:641-6. [Crossref] [PubMed]
- Grunwald JE, Metelitsina TI, Dupont JC, Ying GS, Maguire MG. Reduced foveolar choroidal blood flow in eyes with increasing AMD severity. Invest Ophthalmol Vis Sci 2005;46:1033-8. [Crossref] [PubMed]
- Langham ME, Grebe R, Hopkins S, Marcus S, Sebag M. Choroidal blood flow in diabetic retinopathy. Exp Eye Res 1991;52:167-73. [Crossref] [PubMed]
- Margolis R, Spaide RF. A pilot study of enhanced depth imaging optical coherence tomography of the choroid in normal eyes. Am J Ophthalmol 2009;147:811-5. [Crossref] [PubMed]
- Manjunath V, Taha M, Fujimoto JG, Duker JS. Choroidal thickness in normal eyes measured using Cirrus HD optical coherence tomography. Am J Ophthalmol 2010;150:325-329.e1. [Crossref] [PubMed]
- Zhou H, Dai Y, Shi Y, Russell JF, Lyu C, Noorikolouri J, Feuer WJ, Chu Z, Zhang Q, de Sisternes L, Durbin MK, Gregori G, Rosenfeld PJ, Wang RK. Age-Related Changes in Choroidal Thickness and the Volume of Vessels and Stroma Using Swept-Source OCT and Fully Automated Algorithms. Ophthalmol Retina 2020;4:204-15. [Crossref] [PubMed]
- Ahn SM, Lee SY, Hwang SY, Kim SW, Oh J, Yun C. Retinal vascular flow and choroidal thickness in eyes with early age-related macular degeneration with reticular pseudodrusen. BMC Ophthalmol 2018;18:184. [Crossref] [PubMed]
- Ahn SJ, Ryu SJ, Joung JY, Lee BR. Choroidal Thinning Associated With Hydroxychloroquine Retinopathy. Am J Ophthalmol 2017;183:56-64. [Crossref] [PubMed]
- Pang CE, Sarraf D, Freund KB. Extreme choroidal thinning in high myopia. Retina 2015;35:407-15. [Crossref] [PubMed]
- Garg A, Oll M, Yzer S, Chang S, Barile GR, Merriam JC, Tsang SH, Bearelly S. Reticular pseudodrusen in early age-related macular degeneration are associated with choroidal thinning. Invest Ophthalmol Vis Sci 2013;54:7075-81. [Crossref] [PubMed]
- Mullins RF, Schoo DP, Sohn EH, Flamme-Wiese MJ, Workamelahu G, Johnston RM, Wang K, Tucker BA, Stone EM. The membrane attack complex in aging human choriocapillaris: relationship to macular degeneration and choroidal thinning. Am J Pathol 2014;184:3142-53. [Crossref] [PubMed]
- Read SA, Fuss JA, Vincent SJ, Collins MJ, Alonso-Caneiro D. Choroidal changes in human myopia: insights from optical coherence tomography imaging. Clin Exp Optom 2019;102:270-85. [Crossref] [PubMed]
- Kim JT, Lee DH, Joe SG, Kim JG, Yoon YH. Changes in choroidal thickness in relation to the severity of retinopathy and macular edema in type 2 diabetic patients. Invest Ophthalmol Vis Sci 2013;54:3378-84. [Crossref] [PubMed]
- Manjunath V, Goren J, Fujimoto JG, Duker JS. Analysis of choroidal thickness in age-related macular degeneration using spectral-domain optical coherence tomography. Am J Ophthalmol 2011;152:663-8. [Crossref] [PubMed]
- Laviers H, Zambarakji H. Enhanced depth imaging-OCT of the choroid: a review of the current literature. Graefes Arch Clin Exp Ophthalmol 2014;252:1871-83. [Crossref] [PubMed]
- Taylor GI, Palmer JH. The vascular territories (angiosomes) of the body: experimental study and clinical applications. Br J Plast Surg 1987;40:113-41. [Crossref] [PubMed]
- Taylor GI, Corlett RJ, Ashton MW. The Functional Angiosome: Clinical Implications of the Anatomical Concept. Plast Reconstr Surg 2017;140:721-33. [Crossref] [PubMed]
- Houseman ND, Taylor GI, Pan WR. The angiosomes of the head and neck: anatomic study and clinical applications. Plast Reconstr Surg 2000;105:2287-313. [Crossref] [PubMed]
- Taylor GI. The angiosomes of the body and their supply to perforator flaps. Clin Plast Surg 2003;30:331-42. v. [Crossref] [PubMed]
- Kikuchi S, Miyake K, Tada Y, Uchida D, Koya A, Saito Y, Ohura T, Azuma N. Laser speckle flowgraphy can also be used to show dynamic changes in the blood flow of the skin of the foot after surgical revascularization. Vascular 2019;27:242-51. [Crossref] [PubMed]
- Kawarada O, Yasuda S, Nishimura K, Sakamoto S, Noguchi M, Takahi Y, Harada K, Ishihara M, Ogawa H. Effect of single tibial artery revascularization on microcirculation in the setting of critical limb ischemia. Circ Cardiovasc Interv 2014;7:684-91. [Crossref] [PubMed]
- He Q, Geng W, Li W, Wang RK. Non-contact measurement of neck pulses achieved by imaging micro-motions in the neck skin. Biomed Opt Express 2023;14:4507-19. [Crossref] [PubMed]
- An L, Subhush HM, Wilson DJ, Wang RK. High-resolution wide-field imaging of retinal and choroidal blood perfusion with optical microangiography. J Biomed Opt 2010;15:026011. [Crossref] [PubMed]
- Cheng Y, Chu Z, Wang RK. Robust three-dimensional registration on optical coherence tomography angiography for speckle reduction and visualization. Quant Imaging Med Surg 2021;11:879-94. [Crossref] [PubMed]
- Patel J, Shetty A, Vishwakarma A, Shelke V, editors. Automated Face Detection And Swapping In Video. Proceedings of the First International Conference on Advanced Scientific Innovation in Science, Engineering and Technology, ICASISET 2020, 16-17 May 2020, Chennai, India.
- Zhao J, Deng F, Jia J, Wu C, Li H, Shi Y, Zhang S. A new face feature point matrix based on geometric features and illumination models for facial attraction analysis. Discrete and Continuous Dynamical Systems-S 2019;12:1065-72. [Crossref]
- Chang TR, Tsai MY. Classifying conditions of speckle and wrinkle on the human face: A deep learning approach. Electronics 2022;11:3623. [Crossref]
- Shiohara K, Yamasaki T, editors. Detecting deepfakes with self-blended images. Proceedings of the IEEE/CVF Conference on Computer Vision and Pattern Recognition (CVPR), 2022, pp. 18720-18729.
- He Q, Sun Z, Li Y, Wang W, Wang RK. Spatiotemporal monitoring of changes in oxy/deoxy-hemoglobin concentration and blood pulsation on human skin using smartphone-enabled remote multispectral photoplethysmography. Biomed Opt Express 2021;12:2919-37. [Crossref] [PubMed]
- Zhou H, Chu Z, Zhang Q, Dai Y, Gregori G, Rosenfeld PJ, Wang RK. Attenuation correction assisted automatic segmentation for assessing choroidal thickness and vasculature with swept-source OCT. Biomed Opt Express 2018;9:6067-80. [Crossref] [PubMed]
- Zhang Y, Zhou SW, Noam N, Rabinovitch D, Bar D, Yousif BS, O'Brien R, Gregori G, Wang RK, Rosenfeld PJ, Trivizki O. Influence of Carotid Endarterectomy on Choroidal Perfusion: The INFLATE Study. Ophthalmol Retina 2024;8:62-71. [Crossref] [PubMed]
- Metelitsina TI, Grunwald JE, DuPont JC, Ying GS, Brucker AJ, Dunaief JL. Foveolar choroidal circulation and choroidal neovascularization in age-related macular degeneration. Invest Ophthalmol Vis Sci 2008;49:358-63. [Crossref] [PubMed]
- van Laar PJ, van der Grond J, Bremmer JP, Klijn CJ, Hendrikse J. Assessment of the contribution of the external carotid artery to brain perfusion in patients with internal carotid artery occlusion. Stroke 2008;39:3003-8. [Crossref] [PubMed]
- Fearn SJ, Picton AJ, Mortimer AJ, Parry AD, McCollum CN. The contribution of the external carotid artery to cerebral perfusion in carotid disease. J Vasc Surg 2000;31:989-93. [Crossref] [PubMed]
- Altinbas NK, Ustuner E, Ozcan H, Bilgic S, Sancak T, Dusunceli E. Effect of carotid artery stenting on ophthalmic artery flow patterns. J Ultrasound Med 2014;33:629-38. [Crossref] [PubMed]
- Zhou SW, Zhang Y, Noam N, Rabinovitch D, Bar D, Yousif BS, O'Brien R, Hiya FE, Lin Y, Berni A, Gregori G, Wang RK, Rosenfeld PJ, Trivizki O. The Impact of Carotid Endarterectomy on Choriocapillaris Perfusion. Invest Ophthalmol Vis Sci 2023;64:42. [Crossref] [PubMed]
- Geibprasert S, Pongpech S, Armstrong D, Krings T. Dangerous extracranial-intracranial anastomoses and supply to the cranial nerves: vessels the neurointerventionalist needs to know. AJNR Am J Neuroradiol 2009;30:1459-68. [Crossref] [PubMed]
- Zahedi E, Jaafar R, Ali MA, Mohamed AL, Maskon O. Finger photoplethysmogram pulse amplitude changes induced by flow-mediated dilation. Physiol Meas 2008;29:625-37. [Crossref] [PubMed]
- Selvaraj N, Jaryal AK, Santhosh J, Anand S, Deepak KK. Monitoring of reactive hyperemia using photoplethysmographic pulse amplitude and transit time. J Clin Monit Comput 2009;23:315-22. [Crossref] [PubMed]
- Gandhi PG, Rao GH. The spectral analysis of photoplethysmography to evaluate an independent cardiovascular risk factor. Int J Gen Med 2014;7:539-47. [PubMed]
- Lao CK, Che UK, Chen W, Pun SH, Mak PU, Wan F, Vai MI. Portable heart rate detector based on photoplethysmography with android programmable devices for ubiquitous health monitoring system. International Journal of Advances in Telecommunications, Electrotechnics Signals and Systems 2012;2:18-26.
- Spigulis J, Kukulis I, Fridenberga E, Venckus G, editors. Potential of advanced photoplethysmography sensing for noninvasive vascular diagnostics and early screening. International Symposium on Biomedical Optics, 2002, San Jose, CA, United States.
- Liang Y, Chen Z, Ward R, Elgendi M. Hypertension Assessment Using Photoplethysmography: A Risk Stratification Approach. J Clin Med 2018;8:12. [Crossref] [PubMed]
- Kamshilin AA, Miridonov S, Teplov V, Saarenheimo R, Nippolainen E. Photoplethysmographic imaging of high spatial resolution. Biomed Opt Express 2011;2:996-1006. [Crossref] [PubMed]
- Zaunseder S, Trumpp A, Ernst H, Förster M, Malberg H, editors. Spatio-temporal analysis of blood perfusion by imaging photoplethysmography. SPIE BiOS, 2018, San Francisco, California, United States.
- Zhao Z, Li S, Xu J, Li Y, Huang W, Yang M, Mu L, Liu Y, Zhai H, Jin J, Li J, Li J, Fu X. Color Doppler flow imaging of the facial artery and vein. Plast Reconstr Surg 2000;106:1249-53. [Crossref] [PubMed]
- Ward JB, Hedges TR 3rd, Heggerick PA. Reversible abnormalities in the ophthalmic arteries detected by color Doppler imaging. Ophthalmology 1995;102:1606-10. [Crossref] [PubMed]
- Hwang GJ, Woo SJ, Hwang JM, Jung C, Park KH, Kwon OK. Reversal of ischemic retinopathy following balloon angioplasty of a stenotic ophthalmic artery. J Neuroophthalmol 2010;30:228-30. [Crossref] [PubMed]