Comparison of bone ingrowth and clinical outcome of a collagen-hydroxyapatite bone graft substitute versus autologous bone graft in posterior lumbar interbody fusion
Introduction
Low back pain is the leading cause of years lived with disability (1) and a huge economic burden (2). When conservative measures to treat low back and/or leg pain have failed and a radiological finding that may cause the pain has been identified, surgical intervention can be considered.
Posterior lumbar interbody fusion (PLIF) is a surgical procedure to decompress entrapped nerve roots and stabilize spinal segments using pedicle screw instrumentation and bone graft filled interbody cages. Loads are distributed between the instrumentation, the vertebrae and the cages, to allow bone healing and remodeling. The bony bridges that are subsequently formed will overtake the load in the course of time. The current gold standard of bone graft is autologous bone from the decompression or from the iliac crest. When the amount of available autologous bone is not sufficient as in revision surgery or in case the autologous bone is of poor quality, an artificial bone graft can be considered. Synthetic bone graft substitutes to fill bone defects have been available since the 1980s, serving as alternative osteoconductive materials. Hydroxyapatite (HA) is a ceramic-based material with a structural chemical composition closely mimicking the native bone mineral (3). HA can be used to replace autologous bone in spinal applications resulting in similar fusion rates as reported for autologous bone, without donor site complications (4). Several synthetic materials have been developed to enhance the biochemical and biophysical mimicry of human bone.
RegenOss (Fin-Ceramica Faenza S.p.A., Faenza, Italy) is a porous, 3-dimensional composite bone substitute consisting of type I collagen fibers incorporated with nano-sized crystals of biomimetic magnesium-doped HA (Mg-HA). The type I collagen fibers (from equine source) and doping crystals allow the formation of a composite material with stiffness and elasticity characteristics resembling bone. Osteoblast adhesion is promoted by the information stored in the type I collagen fibers that drives the mineralization process (5,6). Previous clinical studies have demonstrated that RegenOss promotes bone regeneration, assists new bone formation (3,7,8) and is safe to use (9). So far, RegenOss or RegenOss combined with autologous bone has been used to perform posterolateral fusion in scoliosis correction surgery (3,7,8). Giorgi et al. followed 41 patients for 3 years after surgery and reported a fusion rate of 95% (56% bilateral, 39% unilateral fusion) after one year and 95% (61% bilateral, 34% unilateral fusion) after 3 years evaluated on plain radiographs, together with a significant improvement in clinical outcomes (3). Gioia et al. and Grigolo et al. both described a case report in which a histology of a fusion mass biopsy obtained during revision surgery at 1 and 1.5 years postoperatively, showed mature bone of good quality with a well-organized trabecular structure, without remnants of RegenOss bone substitute (7,8). So far, the RegenOss bone substitute was not applied in interbody spinal fusion and the resulting bone mass has only been evaluated by means of plain radiographs and quantified using histology (invasive technique). Positron emission tomography/computed tomography (PET/CT) with the bone seeking agent 18F-fluoride allows for localization and quantification of bone metabolism through imaging (10), already during early phases of the bone fusion process (11,12).
The aim of this study was to evaluate RegenOss as bone graft material in patients undergoing PLIF, as compared to a historical cohort of PLIF patients treated with autologous bone graft. Outcome measures include: bone metabolism activity on 18F-fluoride PET/CT at six weeks and one year postoperatively; bony bridging one year after surgery on CT; and clinical outcome using patient reported outcome measures (PROMs). We present this article in accordance with the STROBE reporting checklist (available at https://qims.amegroups.com/article/view/10.21037/qims-24-755/rc).
Methods
Patients
A consecutive cohort of 15 patients suffering from low-grade lumbar spondylolisthesis who gave written informed consent for this study, was enrolled prospectively at the Maastricht University Medical Center between November 2016 and May 2019 in the RegenOss cohort. See Figure 1A for a flow chart of the patient inclusion. Patients met the following eligibility criteria: aged 18–65 years; body mass index (BMI) of equal to or less than 30 kg/m2; able to read, speak and write in Dutch; spondylolisthesis of L3–L4, L4–L5 or L5–S1, grade I or grade II as documented by plain radiographs, CT or magnetic resonance imaging (MRI). Patients who had undergone previous surgical interventions at the index level; had an active or recent systemic infection; were suffering from an inflammatory disease; had an active or recent malignancy; has had radiotherapy of the lumbar region; were pregnant; or had a spinal deformity were excluded. PROMs were filled out pre-operatively as well as six weeks and one year postoperatively. 18F-fluoride PET/CT scans were acquired at six weeks and one year postoperatively. No power calculation was performed since no previous study evaluated patients shortly after PLIF surgery by 18F-fluoride PET/CT (13). With the suggestion of a minimum of 10 patients needed for a pilot experiment (14), it was decided to include 15 patients for the current cohort study. Adverse events, whether or not related to the used biomaterial, were documented. This study was conducted in accordance with the Declaration of Helsinki (as revised in 2013). The study was approved by the medical ethics committee of the Maastricht University Medical Center (RegenOss cohort: No. METC14-1-056; Autologous cohort: No. METC10-1-025) and all subjects signed an informed consent form. In this cohort study, the RegenOss cohort was compared to an autologous graft cohort of patients suffering from spondylolisthesis that had been enrolled prospectively at the Maastricht University Medical Center between October 2011 and January 2014. Eligibility criteria and exclusion criteria for the autologous graft cohort were the same as for the RegenOss cohort. The autologous graft cohort underwent PLIF surgery with local autologous bone graft from the lamina, and was subjected to a similar study protocol including 18F-fluoride PET/CT six weeks and one year post-operatively, as well as PROMs pre-operatively, at six weeks and one year. The 15 patients from the autologous graft cohort that matched the 15 patients of the RegenOss cohort best in terms of gender, age and BMI were used as standard comparison in this cohort study. See Figure 1B for a flow chart of the patient inclusion.
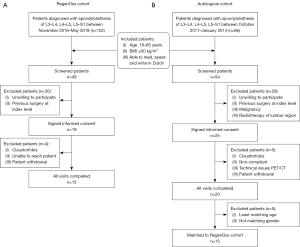
Surgical procedure
PLIF was performed according to routine clinical practice in Maastricht University Medical Center (15,16). With the patient under general anesthesia and in a prone position, the index vertebrae were identified and exposed under fluoroscopic control, using an open posterior lumbar approach. Laminectomy (and facetectomy if deemed necessary) was performed to decompress the nerve roots, followed by excision of the intervertebral disc of the index vertebrae. After thorough preparation of the endplates, two intervertebral cages (Capstone® PEEK, Medtronic, Memphis, U.S.A.) of 10–12 mm in height, were inserted into the disc space. The cages and the remaining disc space were filled with RegenOss® (Finceramica, Faenza, Italy) in the RegenOss cohort and with autologous bone from the removed lamina in the autologous graft cohort. No additional posterolateral fusion was performed. Next, the upper and lower vertebrae were fixed bilaterally using four multi-axial pedicle screws connected to 5.5 mm titanium rods (CD Legacy®, Medtronic, Memphis, U.S.A.) for primary stabilization.
PROMs
Clinical decision making is largely dependent on symptoms that patients experience and therefore it is important to take clinical outcome into account besides radiological findings only, when evaluating a biomaterial. Clinical outcome was assessed using PROMs in the form of validated questionnaires. Three validated questionnaires were used to quantify the pain and disability experienced by the patients. (I) Functional outcome was quantified by the Oswestry Disability Index (ODI) (17,18) ranging from 0 being the lowest functional outcome to 100 being the highest possible functional outcome. (II) The amount of back pain and/or leg pain was quantified by Visual Analogue Scale (VAS) (19), ranging from 0 presenting no pain to 100 presenting the worst imaginable pain. (III) Health-related quality of life was measured using the EuroQol-5D (EQ-5D) (20), calculated based on a Dutch value set (21) and linearly re-scaled from 0 being the worst possible score to 100 being the most optimal score.
18F-fluoride PET/CT scans
18F-fluoride is taken up by sites of new bone formation and serves as a marker of regional bone blood flow and osteoblastic activity (10). 18F-fluoride PET/CT can therefore be used to evaluate earlier phases of the bone fusion process (11,12). An integrated PET/CT scanner was used for acquisition of PET and CT images (Gemini TF 64 PET-CT, Philips, The Netherlands or a Discovery MI 5 ring PET/CT system, GE Healthcare, Milwaukee, WI, USA, both systems complied to the EARL accreditation program). Sixty minutes after intravenous injection of sodium fluoride (18F-NaF) [at six weeks: mean 189.8 MBq (range, 117.7–219.6 MBq) for the RegenOss cohort and mean 194.9 MBq (range, 100–221.6 MBq) for the autologous cohort; at one year: mean 183.1 MBq (range, 88.2–216.9 MBq) for the RegenOss cohort and mean 199.9 MBq (range, 146–219.8 MBq) for the autologous graft cohort], a low-dose CT scan (120 kV, 30 mAs, slice thickness 4 mm) was acquired covering the lumbosacral spine. Subsequently, a static PET scan of two bed positions (5 minutes each) was acquired. Immediately thereafter, a diagnostic CT scan (64-slice helical, 250 mAs, 120 kV, slice thickness 1 mm, increment 0.8 mm) was acquired. Clinical software (EBW, Philips, The Netherlands) and research software for molecular imaging (PMOD 3.7, PMOD Technologies LLC, Zürich) was used for further analysis.
Interbody fusion scoring
In spinal fusion surgery, CT can be used reliably to evaluate the mineralized bony bridges formed between the involved vertebrae from approximately one year after surgery onwards. Interbody fusion was assessed according to Glassman et al. (22) on the diagnostic CT scan at one year after surgery. Grade 1 represents no fusion, grade 2 represents partial or limited unilateral fusion, grade 3 represents partial or limited bilateral fusion, grade 4 represents solid unilateral fusion and grade 5 represents solid bilateral fusion (22). Solid fusion was defined as bone mass progressing between endplates without interruptions. Partial or limited fusion was defined as bone mass progressing between the endplates with interruptions. No fusion was defined as no bone mass present between the endplates. Fusion was assessed by three independent blinded observers (dedicated musculoskeletal radiologist D.L. with over 10 years of experience in an academic setting, orthopedic surgeon P.C.W. specialized in spine surgery with over 25 years of experience with spinal fusion patients, professor of Translational Biomaterials Research J.A.A., specialized in biomaterials, fusion and ingrowth). After consensus was reached, patients were considered as successfully fused when a Glassman grade of 4 or 5 was assigned. The fusion was considered as not completed for patients with a Glassman grade of 1, 2 or 3.
18F-fluoride PET/CT analysis
PET parameters were calculated based on a region of interest (ROI) approach (16). Three ROIs were manually drawn in each low-dose CT in the upper endplate (endUP), intervertebral disc (inter) and lower endplate (endLOW) of the operated segment following the contours of the vertebrae. Three control ROIs were manually drawn in each low-dose CT in the upper endplate (endUP_C), intervertebral disc (inter_C) and lower endplate (endLOW_C) of a control segment two levels above the operated segment. The six ROIs were subsequently copied to the co-registered static PET image. The maximum standardized uptake value [SUVmax (g/mL)] within each ROI was obtained, by correction of the measured radioactivity concentration [A (kBq/mL)] with the injected dose of 18F-fluoride [ID (MBq)] and the body weight of the patient [m (kg)] according to Eq. [1] assuming a body density of 1 g/mL.
Statistical analysis
Statistical analysis was performed using IBM SPSS Statistics version 23.0 (Armonk, NY: IBM Corporation). Differences in patient demographics and PROMs between the RegenOss cohort and the autologous graft cohort were evaluated by an independent t-test when normally distributed and a Mann-Whitney U test otherwise. Differences in 18F-fluoride PET/CT outcome measures between follow-up moments (six weeks compared to one year) were assessed by a paired samples t-test when normally distributed and a Wilcoxon Signed-Rank test otherwise. Differences in 18F-fluoride PET/CT outcome measures between graft cohorts (RegenOss cohort and autologous graft cohort) were assessed by an independent t-test when normally distributed and a Mann-Whitney U test otherwise. All tests were two-tailed. Statistically significant differences were indicated by P values ≤0.05.
Results
Patients
The characteristics of the 15 patients included in the RegenOss cohort are presented next to the characteristics of the 15 patients in the autologous graft cohort in Table 1. Mean age of the RegenOss patients at surgery was 54.8 years, ranging from 39 to 69 years. Mean age of patients in the autologous graft cohort was 54.9 years, ranging from 41 years to 64 years. Age at surgery and BMI of the patients in the RegenOss cohort were similar to the patients in the autologous graft cohort (respectively P=0.965 and P=0.717). Within the RegenOss cohort, seven patients were operated at lumbar level L4–L5, seven patients were operated at L5–S1, one patient was operated at L3–L4. Within the autologous cohort, seven patients were operated at lumbar level L4–L5 and eight patients were operated at L5–S1. Follow-up intervals were similar for the RegenOss cohort and the autologous cohort at 6 weeks (6.0±0.60 and 6.4±0.61 respectively, P=0.131) and at 1 year (53.5±4.7 and 52.8±2.9 respectively, P=0.617). Serious adverse events in the RegenOss patients included wound leakage (n=1), screw malpositioning (n=2) without clinical consequences, coronavirus disease 2019 (COVID-19) infection (n=1). Serious adverse events related to the surgical intervention in the autologous graft cohort included wound leakage (n=4) and screw malpositioning (n=1). Wound leakage was defined as persistent leakage for more than 5 days, without signs of infection. For the cases of wound leakage, one patient was readmitted for observation, one patient was treated with antibiotics and one patient suffered from persistent wound leakage of 2 weeks. In none of these cases, surgical debridement was indicated. There were no signs of cerebrospinal fluid leakage. The case of screw malpositioning required operative screw repositioning.
Table 1
Demographics | RegenOss cohort (n=15) | Autologous graft cohort (n=15) | P value |
---|---|---|---|
Age at surgery (years) | 54.8±8.6 | 54.9±7.9 | 0.965 |
Gender | n.a. | ||
Male | 9 | 9 | |
Female | 6 | 6 | |
BMI (kg/m2) | 28.1±4.3 | 27.6±2.5 | 0.717 |
Type of spondylolisthesis | n.a. | ||
Degenerative | 9 | 5 | |
Isthmic | 6 | 10 | |
Degree of spondylolisthesis | n.a. | ||
1 | 10 | 7 | |
2 | 5 | 8 | |
Level | n.a. | ||
L3–L4 | 1 | 0 | |
L4–L5 | 7 | 7 | |
L5–S1 | 7 | 8 |
Data are presented as mean ± standard deviation or as the number of patients (n) with a certain characteristic. BMI, body mass index; n.a., not applicable; kg, kilograms; m, meter.
PROMs
PROMs pre-operatively, at six weeks and at one year postoperatively for both the RegenOss cohort and the autologous graft cohort are presented in Figure 2. At baseline, the ODI score and EQ-5D score were significantly lower in the RegenOss cohort as compared to the autologous graft cohort (P=0.02 for ODI, P=0.03 for EQ-5D). However, at one year after surgery, no differences in PROMs were detected between the cohorts (P=0.12 for ODI, P=0.15 for VAS, P=0.22 for EQ-5D).
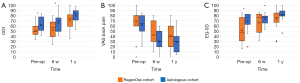
Interbody fusion scoring
The fusion scores according to the Glassman scoring system for both the RegenOss cohort and the autologous graft cohort are presented in Table 2. Seven of the 15 RegenOss patients (47%) showed at least one solid bony bridge (Glassman score 4 or 5) and therefore fusion was attained. The remaining 8 patients (53%) had one or two partial bony bridges and were considered as not fused. For the autologous graft cohort, fusion was attained in 8 of the 15 patients (53%) (Glassman score 4 or 5), and 7 of the 15 patients (47%) had one or two partial bony bridges and were considered as not fused. Interbody fusion scoring can be considered in the same order of magnitude for both cohorts. None of the patients, either fused or not fused, had clinical symptoms at 1 year follow-up that required revision surgery.
Table 2
Fusion status | Glassman grades | RegenOss cohort (number of patients) | Autologous graft cohort (number of patients) |
---|---|---|---|
Incomplete fusion | Grade 1—no fusion | 0 | 0 |
Grade 2—partial or limited unilateral fusion | 1 | 1 | |
Grade 3—partial or limited bilateral fusion | 7 | 6 | |
Complete fusion | Grade 4—solid unilateral fusion | 3 | 4 |
Grade 5—solid bilateral fusion | 4 | 4 |
18F-fluoride PET/CT scan analysis
An overview of the SUVmax values of the RegenOss cohort at both time points for each of the six ROIs is presented in Figure 3A,3B, next to the same graphs for the autologous graft cohort (Figure 3C,3D). For both the RegenOss cohort and the autologous graft cohort, no significant differences were observed between the three control regions at 6 weeks and 1 year after surgery (endUP_C, inter_C, endLOW_C). For the RegenOss cohort, the SUVmax values in the endplates of the operated level at one year postoperatively were significantly lower compared to at six weeks postoperatively, indicating a lower bone metabolism at one year follow-up [P value of 0.003 for the upper operated endplate (endUP), P value of 0.005 for the lower operated endplate (endLOW)]. The bone metabolism in the intervertebral disc space of the operated segment was not significantly different between the six weeks and one year follow-up time point [P value of 0.074 for the intervertebral disc space (inter)]. Similar findings were observed for the autologous graft cohort in the operated upper and lower operated endplate; significantly lower SUVmax values were found at one year as compared to at 6 weeks postoperatively (P=0.001 for endUP, P=0.001 for endLOW). In the autologous graft cohort a significant decrease in SUVmax values within the operated intervertebral disc space was also observed (P=0.05). No significant differences were found between the SUVmax values of the RegenOss cohort and the autologous graft cohort at any of the time points.
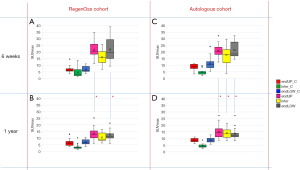
Discussion
This study presents the clinical (by means of PROMs) and radiological (by means of 18F-fluoride PET/CT) follow-up of PLIF patients fused by means of RegenOss, a porous, 3-dimensional composite bone substitute made of collagen fibers including HA, in comparison to a historical patient cohort treated with autologous bone graft.
RegenOss showed similar bone metabolism activity at six weeks on PET/CT as compared to autologous graft, as well as similar fusion rates at one year on CT. In that sense, the biomaterial RegenOss could be used as grafting material in case autologous bone is not available or expected to be of insufficient quality, for instance in case of a previous decompression. How the RegenOss bone substitute compares to other bone graft substitutes such as demineralized bone matrix (DBM) is left to be evaluated. A meta-analysis reported similar fusion rates for patients treated with DBM grafts compared to patients treated with autologous grafts in posterolateral spinal fusion as well as in interbody spinal fusion (23), basically similar to our findings for the RegenOss cohort compared to the autologous cohort. The rate of adverse events possibly related to the graft material was similar for RegenOss and the autologous graft cohort, as previously confirmed by clinical safety studies (3,7,8) and a post-marketing analysis (9) carried out with the device. Clinical questionnaires at multiple timepoints showed significant improvement of the clinical outcome of the patients comparing the situation before and after surgery in both cohorts.
A decline in the bone metabolism level was seen when comparing the six weeks time point to one year after surgery in operated segments, but was still higher compared to the bone metabolism level in non-operated segments. This finding is coherent with earlier studies who reported elevated bone metabolism values over the course of several years postoperatively in operated bone regions (24,25). Brenner et al. reported sustained elevated bone metabolism levels over time in unsuccessful cases (24). Therefore, elevated levels of bone metabolism at one year in the current study might indicate ongoing bony bridging. However, other processes such as subsidence can also elevate bone metabolism values (26). We did not differentiate between elevated bone metabolism as a result of non-fusion and elevated bone metabolism as a result of subsidence, in this study. Both for the RegenOss cohort and for the autologous graft cohort, the bone metabolism values decreased over time, suggesting successful (stable) union of vertebrae. The bone metabolism values over time for the RegenOss cohort and the autologous graft cohort were similar.
Limitations of the study include the relatively small number of patients included in the study. Moreover, the comparison of the RegenOss patients against the autologous patients would preferably have been randomized as opposed to the current comparison with a historical autologous graft cohort. Conclusions thus should be drawn cautiously, as possible changes in overall patient treatment in the hospital at the time of both cohorts may have imposed bias. Another limitation was that the method of final ingrowth assessment, in other words assessing whether segments were fused or not, was based on CT one year postoperatively. The assessment of either fusion or non-fusion on CT is not perfect (27). However, studies showed that CT imaging is the most reliable noninvasive method to assess fusion after PLIF (27,28). The strict definition of fusion combined with the fact that bony fusion may very well occur later than one year after surgery, may explain the relatively high amount of non-fusion segments observed in both cohorts in this study. A grade of impartial fusion at one year after surgery does not mean that the patient will not attain fusion at a later time point. In that sense, a follow-up period of only one year after surgery was a limitation in this study. Nevertheless, these limitations apply for both the RegenOss and autologous graft cohort. In the current study, specific inclusion criteria were set to minimize the factors that can affect bone metabolism activity, apart from the difference in the type of graft used during PLIF. However, we have no reason to think that the findings of this study would not apply to patients that are operated at different spinal levels or when PLIF is performed over multiple segments. The findings cannot be directly generalized for all types of graft material that may be similar to the RegenOss material, and for which a separate study cohort should be followed-up by means of PET/CT.
Conclusions
In conclusion, bony bridging scored on CT at one year after PLIF surgery was similar for the RegenOss bone graft cohort compared to the autologous bone graft cohort. Furthermore, we found that PROM scores and PET/CT values after PLIF surgery, were also similar for both graft cohorts. RegenOss appeared to be safe to use and as a bone graft substitute clinically and radiographically comparable to the gold standard of autologous bone graft.
Acknowledgments
None.
Footnote
Reporting Checklist: The authors have completed the STROBE reporting checklist. Available at https://qims.amegroups.com/article/view/10.21037/qims-24-755/rc
Funding: This work was supported by Fin-Ceramica (Faenza S.p.A., Faenza, Italy) for funding the 18F-fluoride PET/CT scans at six weeks and one year postoperatively in the RegenOss cohort, and an External Research Program grant from Medtronic partially covering 18F-fluoride PET/CT scanning costs in the autologous graft cohort. Fin-Ceramica and Medtronic were not involved in the study execution nor data analysis.
Conflicts of Interest: All authors have completed the ICMJE uniform disclosure form (available at https://qims.amegroups.com/article/view/10.21037/qims-24-755/coif). The authors have no conflicts of interest to declare.
Ethical Statement: The authors are accountable for all aspects of the work in ensuring that questions related to the accuracy or integrity of any part of the work are appropriately investigated and resolved. The study was conducted in accordance with the Declaration of Helsinki (as revised in 2013). The study was approved by the medical ethics committee of the Maastricht University Medical Center (RegenOss cohort: No. METC14-1-056; Autologous cohort: No. METC10-1-025) and informed consent was taken from all individual participants.
Open Access Statement: This is an Open Access article distributed in accordance with the Creative Commons Attribution-NonCommercial-NoDerivs 4.0 International License (CC BY-NC-ND 4.0), which permits the non-commercial replication and distribution of the article with the strict proviso that no changes or edits are made and the original work is properly cited (including links to both the formal publication through the relevant DOI and the license). See: https://creativecommons.org/licenses/by-nc-nd/4.0/.
References
- GBD 2017 Disease and Injury Incidence and Prevalence Collaborators. Global, regional, and national incidence, prevalence, and years lived with disability for 354 diseases and injuries for 195 countries and territories, 1990-2017: a systematic analysis for the Global Burden of Disease Study 2017. Lancet 2018;392:1789-858. [Crossref] [PubMed]
- Hartvigsen J, Hancock MJ, Kongsted A, Louw Q, Ferreira ML, Genevay S, Hoy D, Karppinen J, Pransky G, Sieper J, Smeets RJ, Underwood MLancet Low Back Pain Series Working Group. What low back pain is and why we need to pay attention. Lancet 2018;391:2356-67. [Crossref] [PubMed]
- Giorgi P, Capitani D, Sprio S, Sandri M, Tampieri A, Canella V, Nataloni A, Schirò GR. A new bioinspired collagen-hydroxyapatite bone graft substitute in adult scoliosis surgery: results at 3-year follow-up. J Appl Biomater Funct Mater 2017;15:e262-70. [Crossref] [PubMed]
- Alsaleh KA, Tougas CA, Roffey DM, Wai EK. Osteoconductive bone graft extenders in posterolateral thoracolumbar spinal fusion: a systematic review. Spine (Phila Pa 1976) 2012;37:E993-1000. [Crossref] [PubMed]
- Tampieri A, Sprio S, Sandri M, Valentini F. Mimicking natural bio-mineralization processes: a new tool for osteochondral scaffold development. Trends Biotechnol 2011;29:526-35. [Crossref] [PubMed]
- Landi E, Tampieri A, Celotti G, Langenati R, Sandri M, Sprio S. Nucleation of biomimetic apatite in synthetic body fluids: dense and porous scaffold development. Biomaterials 2005;26:2835-45. [Crossref] [PubMed]
- Grigolo B, Dolzani P, Giannetti C, Tenucci M, Calvosa G. Use of a fully-resorbable, biomimetic composite hydroxyapatite as bone graft substitute for posterolateral spine fusion: A case report. Int J Clin Exp Med 2016;9:22458-62.
- Gioia G, Agnoletto M, Di Giancamillo A, Domenicucci M, Mangiavini L, Lombardo MDM, Brambilla L, Peretti GM. Histological assessment of new bone formation with biomimetic scaffold in posterolateral lumbar spine fusion. J Biol Regul Homeost Agents 2020;34:99-103. Congress of the Italian Orthopaedic Research Society.
- Barbanti Brodano G, Griffoni C, Zanotti B, Gasbarrini A, Bandiera S, Ghermandi R, Boriani S. A post-market surveillance analysis of the safety of hydroxyapatite-derived products as bone graft extenders or substitutes for spine fusion. Eur Rev Med Pharmacol Sci 2015;19:3548-55.
- Beheshti M, Mottaghy FM, Paycha F, Behrendt FFF, Van den Wyngaert T, Fogelman I, Strobel K, Celli M, Fanti S, Giammarile F, Krause B, Langsteger W. (18)F-NaF PET/CT: EANM procedure guidelines for bone imaging. Eur J Nucl Med Mol Imaging 2015;42:1767-77. [Crossref] [PubMed]
- Cofano F, Armocida D, Ruffini L, Scarlattei M, Baldari G, Di Perna G, Pilloni G, Zenga F, Ballante E, Garbossa D, Tartara F. The Efficacy of Trabecular Titanium Cages to Induce Reparative Bone Activity after Lumbar Arthrodesis Studied through the 18f-Naf PET/CT Scan: Observational Clinical In-Vivo Study. Diagnostics (Basel) 2022.
- Loenen ACY, Peters MJM, Wierts R, Bevers RTJ, van Rhijn LW, Arts JJ, Willems PC. Local bone metabolism during the consolidation process of spinal interbody fusion. J Bone Miner Metab 2022;40:220-8. [Crossref] [PubMed]
- Awosika T, Davidar AD, Hersh AM, Menta A, Weber-Levine C, Alomari S, Khan MA, Theodore N. SPECT/CT and PET/CT for the Evaluation of Persistent or Recurrent Pain After Spine Surgery: A Systematic Review and Case Series. World Neurosurg 2024;182:e344-59. [Crossref] [PubMed]
- Nieswiadomy RM. Foundations of nursing research. 4th ed. Upper Saddle River, NJ: Pearson Education; 2002.
- Peters M, Willems P, Weijers R, et al. Pseudarthrosis after lumbar spinal fusion: the role of 18F-fluoride PET/CT. Eur J Nucl Med Mol Imaging 2015;42:1891-8. [Crossref] [PubMed]
- Peters MJ, Wierts R, Jutten EM, Halders SG, Willems PC, Brans B. Evaluation of a short dynamic 18F-fluoride PET/CT scanning method to assess bone metabolic activity in spinal orthopedics. Ann Nucl Med 2015;29:799-809. [Crossref] [PubMed]
- Fairbank JC, Couper J, Davies JB, O'Brien JP. The Oswestry low back pain disability questionnaire. Physiotherapy 1980;66:271-3.
- Ghogawala Z, Resnick DK, Watters WC 3rd, Mummaneni PV, Dailey AT, Choudhri TF, Eck JC, Sharan A, Groff MW, Wang JC, Dhall SS, Kaiser MG. Guideline update for the performance of fusion procedures for degenerative disease of the lumbar spine. Part 2: assessment of functional outcome following lumbar fusion. J Neurosurg Spine 2014;21:7-13. [Crossref] [PubMed]
- Zanoli G, Strömqvist B, Jönsson B. Visual analog scales for interpretation of back and leg pain intensity in patients operated for degenerative lumbar spine disorders. Spine (Phila Pa 1976) 2001;26:2375-80. [Crossref] [PubMed]
- Lamers LM, Stalmeier PF, McDonnell J, Krabbe PF, van Busschbach JJ. Measuring the quality of life in economic evaluations: the Dutch EQ-5D tariff. Ned Tijdschr Geneeskd 2005;149:1574-8.
- Lamers LM, McDonnell J, Stalmeier PF, Krabbe PF, Busschbach JJ. The Dutch tariff: results and arguments for an effective design for national EQ-5D valuation studies. Health Econ 2006;15:1121-32. [Crossref] [PubMed]
- Glassman SD, Dimar JR, Carreon LY, Campbell MJ, Puno RM, Johnson JR. Initial fusion rates with recombinant human bone morphogenetic protein-2/compression resistant matrix and a hydroxyapatite and tricalcium phosphate/collagen carrier in posterolateral spinal fusion. Spine (Phila Pa 1976) 2005;30:1694-8. [Crossref] [PubMed]
- Han S, Park B, Lim JW, Youm JY, Choi SW, Kim DH, Ahn DK. Comparison of Fusion Rate between Demineralized Bone Matrix versus Autograft in Lumbar Fusion: Meta-Analysis. J Korean Neurosurg Soc 2020;63:673-80. [Crossref] [PubMed]
- Brenner W, Vernon C, Conrad EU, Eary JF. Assessment of the metabolic activity of bone grafts with (18)F-fluoride PET. Eur J Nucl Med Mol Imaging 2004;31:1291-8. [Crossref] [PubMed]
- Piert M, Winter E, Becker GA, Bilger K, Machulla H, Müller-Schauenburg W, Bares R, Becker HD. Allogenic bone graft viability after hip revision arthroplasty assessed by dynamic 18Ffluoride ion positron emission tomography. Eur J Nucl Med 1999;26:615-24. [Crossref] [PubMed]
- Brans B, Weijers R, Halders S, Wierts R, Peters M, Punt I, Willems P. Assessment of bone graft incorporation by 18 F-fluoride positron-emission tomography/computed tomography in patients with persisting symptoms after posterior lumbar interbody fusion. EJNMMI Res 2012;2:42. [Crossref] [PubMed]
- Choudhri TF, Mummaneni PV, Dhall SS, Eck JC, Groff MW, Ghogawala Z, Watters WC 3rd, Dailey AT, Resnick DK, Sharan A, Wang JC, Kaiser MG. Guideline update for the performance of fusion procedures for degenerative disease of the lumbar spine. Part 4: radiographic assessment of fusion status. J Neurosurg Spine 2014;21:23-30. [Crossref] [PubMed]
- Raizman NM, O'Brien JR, Poehling-Monaghan KL, Yu WD. Pseudarthrosis of the spine. J Am Acad Orthop Surg 2009;17:494-503. [Crossref] [PubMed]