Value of two-dimensional speckle-tracking echocardiography in the assessment of left atrial function in patients with chronic kidney disease
Introduction
The rising prevalence of chronic kidney disease (CKD) has emerged as a global public health concern, posing a significant threat to human health (1). In 2017, a systematic analysis of the global all-age Global Burden of Disease (GBD) study project found that there were 697.5 million cases of CKD at all stages worldwide, representing a global prevalence of 9.1%, with CKD and its impact on cardiovascular disease (CVD) causing 2.6 million deaths (2). As renal dysfunction progresses, the prevalence of CVD in patients with CKD is directly related to the severity of CKD (3). Many patients with CKD succumb to severe complications related to renal dysfunction before reaching the end stage of the disease, with CVD being the most prevalent complication and leading cause of death among this population (1).
During the cardiac cycle, the left atrium (LA) is closely linked to the left ventricle, and LA function is essential for maintaining efficient cardiac function, especially during exercise (4,5). It helps increase stroke volume and cardiac output by enhancing left ventricular (LV) filling. Structural and functional changes in the LA, such as dilation and reduced compliance, are associated with adverse cardiovascular outcomes, including atrial fibrillation, heart failure, and stroke (4,6,7).
Changes in LA volume and diameters are independent risk factors for CVD, death, or atrial fibrillation in patients with CKD (8-10). Moreover, research has indicated (11-13) that alterations in LA function often occur before structural changes, and that two-dimensional speckle-tracking echocardiography (2D-STE) is more effective in detecting these functional changes earlier than is conventional echocardiography.
2D-STE tracks the spatial movement of echo specks within the myocardium to reflect the real-time movement and deformation of the myocardial tissue. It is a critical tool for evaluating LA function, as it can evaluate LA mechanics by measuring strain and strain rate, which reflect the deformation and deformation rate of the atrial myocardium, respectively. 2D-STE is superior to conventional echocardiography in detecting subclinical myocardial dysfunction due to its higher sensitivity, angle independence, comprehensive assessment of myocardial mechanics, and superior prognostic ability (14,15).
LA strain and LA volume index (LAVI) are more sensitive parameters than are traditional echocardiographic parameters and LV strain in patients with early CKD (16). Saito et al. (17). demonstrated that myocardial fibrosis can be detected by 2D strain. Given that the atrium has thinner walls than the ventricle has, it is possible that alterations in myocardial deformation may be detected earlier in the atrium through use of strain analysis. With the deterioration of renal function, the risk of CVDs in patients with CKD significantly increases, which emphasizes the importance of early identification and intervention.
Although numerous studies have employed 2D-STE to evaluate changes in left ventricle geometry (such as increased wall thickness and/or dilation) and function in patients with CKD (18-20), few studies have focused on changes in LA function. Therefore, we conducted this study to assess the changes in LA function in patients with CKD with LV hypertrophy (LVH) using 2D-STE, and to explore the independent correlations between baseline parameters and LA strain and strain rate. We present this article in accordance with the STROBE reporting checklist (available at https://qims.amegroups.com/article/view/10.21037/qims-24-1537/rc).
Methods
Study population
The study was conducted in accordance with the Declaration of Helsinki (as revised in 2013). The study was approved by the Provincial Ethics Committee of Shenzhen People’s Hospital (No. LL-KY-2021282). All individuals in this prospective study provided written informed consent prior to their participation.
The study included 166 patients with CKD who visited Shenzhen People’s Hospital between November 2020 and September 2021. The diagnostic criteria for CKD (21) were a glomerular filtration rate (GFR) <60 mL/min/1.73 m2 for ≥3 months with or without renal injury, including structural and functional renal abnormalities for ≥3 months with or without a GFR decline. Patients were excluded if they had a low ejection fraction (EF <50%), coronary artery disease, severe valvular disease, congenital heart disease, or severe arrhythmia (including atrial fibrillation) or had undergone renal transplantation. All patients were clinically and hemodynamically stable. The study flowchart of the inclusion and exclusion of patients with CKD is shown in Figure 1. Additionally, 70 healthy participants were collected as a healthy control group, and people with underlying diseases such as heart disease, diabetes, liver and kidney diseases, etc. were excluded.
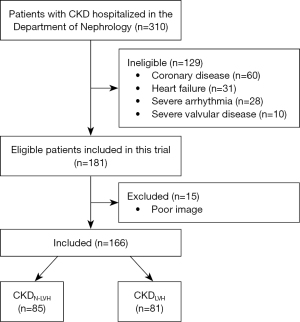
Clinical characteristics
Clinical data collected included gender, age, height, weight, blood pressure, heart rate, GFR, serum creatinine (SCr), blood urea nitrogen (BUN), echocardiographic parameters, the body mass index (BMI) [BMI = weight (kg)/height2 (m2)], and body surface area (BSA) [BSA = 0.010061 × height (cm) + 0.010124 × weight (kg) − 0.010099].
Echocardiographic analysis
A comprehensive transthoracic echocardiography (TTE) scan was conducted with the participants at rest with a Vivid E95 diagnostic ultrasound machine (GE HealthCare, Chicago, IL, USA) equipped with an M5S probe at 3.5 MHz. The participants were instructed to lie in the left decubitus position, and the electrocardiogram was connected and recorded simultaneously to ensure optimal image quality. LV ejection fraction (LVEF) was determined using the biplane Simpson method. M-mode echocardiographic measurements included LV end-diastolic dimension (LVEDD) and LV end-systolic dimension (LVESD), interventricular septal thickness at end-diastole (IVSd), posterior wall thickness at end-diastole (PWTd), and systolic anterior-posterior LA diameters in the end-systolic period (LAd) in long-axis views from the parasternal sternum. LV mass (LVM) and LV mass index (LVMI) were calculated using the Devereux correction formula as follows: LVM (g) = 0.8 × {1.04[(IVSd + PWTd + LVEDD)3 − LVEDD3] + 0.6}; LVMI (g/m2) = LVM/BSA. The criterion for LVH being LVMI was ≥115 g/m2 for men and was ≥95 g/m2 for women (22). LV end-diastolic volume (LVEDV) was calculated using the Teichholtz correction formula (23) as follows: LVEDV =7.0 × LVEDD3/(2.4 + LVEDD). The LV end-diastolic volume index (LVEDVI) was normalized to BSA (LVEDV/BSA). All recordings and measurements adhered to the guidelines of the American Society of Echocardiography (24). Pulsed Doppler was employed to measure early diastolic peak flow velocity (E peak), late diastolic peak flow velocity (A peak), the E/A ratio, and E peak deceleration time (DT) at the mitral orifice. Tissue Doppler imaging (TDI) velocity mode was used to measure the peak early diastolic velocity (e’) through placement of the sampling volume in the septal and lateral wall myocardium at the level of the mitral annulus. The average e’ from these two values was used to calculate the E/e’ ratio.
LA volume analysis
Apical four-chamber and two-chamber view cines (70–90 fps) of the LA apex were acquired. These cines were imported into EchoPAC version 203 software (GE HealthCare) in stored digital raw data format for analysis. The LA endocardium was manually outlined in each of the two apical dynamic images. The LA maximum volume (LAVmax) was measured at end-systole just before the mitral valve opened, the LA minimum volume (LAVmin) was measured at end-diastole at the apex of the R-wave, and the presystolic LA volume (LAVpre) was measured at the onset of the P-wave (25). The LA total emptying volume (LAVt; LAVt = LAVmax − LAVmin), LA total emptying fraction (LAVtEF; LAVtEF = LAVt/LAVmax), LA passive emptying volume (LAVp; LAVp = LAVmax − LAVpre), LA passive emptying fraction (LAVpEF; LAVpEF = LAVp/LAVmax), conduit volume (CV; CV=SV − LAVt), LA active emptying volume (LAVa; LAVa = LAVpre − LAVmin), and LA active emptying fraction (LAVaEF = LAVa/LAVpre) were calculated based on these three LA volume indicators (25). All calculated LA volume indicators were adjusted for BSA to obtain the LAVI. LAVt and LAVtEF reflect LA reservoir function; LAVp, LAVpEF, and CV reflect LA conduit function; and LAVa and LAVaEF LA booster pump function.
LA strain and strain rate analysis
Apical four-chamber and two-chamber views cines (70–90 fps) of the LA apex were obtained. These images were imported in stored digital raw data format into EchoPAC version 203 software (GE HealthCare) for analysis. The 2D-STE analysis software was used to outline the LA endocardial surface in the dynamic 2D image when it was most distinct. The region of interest was automatically generated by the software, with the width of the region adjusted to include the entire layer of the myocardium. After running the program, the software automatically tracked the myocardial motion in the region of interest frame by frame to generate the strain and strain rate curves of the atrial wall. The global longitudinal strains (GLSs) (LA Ss, LA Se, LA Sa) and strain rates (LA SRs, LA SRe, LA SRa) of the LA wall were recorded during systole, early diastole, and late diastole, respectively, and the specific measurement methods were shown in Figure 2.
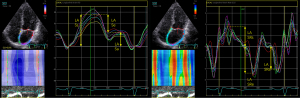
Statistical analysis
The statistical software SPSS 26.0 (IBM. Corp., Armonk, NY, USA) was used for data analysis. Continuous variables are presented as the mean ± standard deviation or as the median with interquartile range. One-way analysis of variance (ANOVA) was employed for comparisons between multiple groups when the data followed a normal distribution and variances were homogeneous. For homogeneous variances, the least significant difference (LSD) t-test method was used, while the Kruskal-Wallis test was applied for inhomogeneous variances. The Chi-squared test was used for comparing count data. General linear regression was conducted to examine the relationship between different variables and LA strain and strain rate. Multifactorial linear regression analyzed the relationship between LVMI and LA strain and strain rate. The first multivariate model was adjusted for age, systolic blood pressure (SBP), diastolic blood pressure (DBP), BSA, GFR, and BUN; the second model was adjusted for E peak, A peak, e’, E/e’, DT, LV GLS, LVEF, LVEDVI, and LAVI; the third model included all parameters from models I and II. Interobserver agreement was assessed by two independent researchers who randomly selected 20 participants. Intraobserver agreement was evaluated by the same researcher, who analyzed 20 participants twice. The intragroup correlation coefficient (ICC) and the Bland-Altman method were used to test intra- and interobserver agreement for LA strain and strain rate. All statistical tests were two-tailed, with P<0.05 considered statistically significant.
Results
Clinical and laboratory characteristics
The study included 236 participants: 166 patients with CKD (85 in the CKDN-LVH group and 81 in the CKDLVH group) and 70 healthy controls (CON group). The mean age of the patients with CKD was 53.88±13.68 years, with 103 males and 63 females. The clinical and laboratory characteristics of the groups are detailed in Table 1. There were no statistically significant differences in age, gender, BMI, or BSA between the groups (P>0.05). Compared to the CON group, the CKD group exhibited higher SBP and DBP, faster heart rates, lower GFR, and higher SCr and BUN levels (P<0.05).
Table 1
Variables | CON (n=70) | CKDN-LVH (n=85) | CKDLVH (n=81) | P |
---|---|---|---|---|
Age (years) | 51.11±9.53 | 53.27±13.21 | 54.52±14.22 | 0.252 |
Gender (male) | 33 (47.1) | 59 (69.4) | 44 (54.3) | 0.060 |
SBP (mmHg) | 117.56±9.58 | 134.08±19.08* | 149.46±17.14*# | <0.001 |
DBP (mmHg) | 75.57±8.37 | 84.14±11.66* | 87.28±12.92* | <0.001 |
BMI (kg/m2) | 23.26±3.41 | 23.26±3.41 | 24.19±4.77 | 0.145 |
BSA (m2) | 1.70±0.19 | 1.71±0.19 | 1.67±0.18 | 0.369 |
HR (bpm) | 67.94±9.43 | 74.72±11.19* | 71.98±11.26* | 0.001 |
GFR (mL/min/1.73 m2) | 102.20±16.48 | 29.37±23.14* | 15.14±18.04*# | <0.001 |
SCr (µmol/L) | 71.53±15.70 | 428.19±413.71* | 636.09±386.14*# | <0.001 |
BUN (mmol/L) | 4.94±3.12 | 16.01±10.84* | 20.99±11.31*# | <0.001 |
Continuous data are expressed as the mean ± standard deviation. Categorical data are expressed as number (%). *, P<0.05 indicates significant difference from the control group; #, P<0.05 indicates significant difference from the CKDN-LVH group. CON, control; CKD, chronic kidney disease; LVH, left ventricular hypertrophy; N-LVH, non-LVH; SBP, systolic blood pressure; DBP, diastolic blood pressure; BMI, body mass index; BSA, body surface area; HR, heart rate; GFR, glomerular filtration rate; SCr, serum creatinine; BUN, blood urea nitrogen.
TTE parameters and LV longitudinal strain
Table 2 summarizes the TTE parameters and LV longitudinal strain. In the CKDLVH group, LVEF, e’ average TDI, and GLS were lower compared to the other two groups, while LVMI, LVEDVI, E peak, A peak, and E/e’ were higher. The LA volume in the CKDLVH group was higher than that in the other groups, and each EF value of the LA of the CKDLVH group was lower compared to that of the other groups. LVMI, A peak, and E/e’ were higher in the CKDLVH group compared to the CON group, while the e’ average TDI, and GLS were lower compared to the CON group (P<0.05).
Table 2
Variables | CON (n=70) | CKDN-LVH (n=85) | CKDLVH (n=81) | P |
---|---|---|---|---|
LVEF (%) | 68.72±6.05 | 68.86±5.83 | 66.43±6.42*# | 0.020 |
LVMI (g/m2) | 76.43±14.58 | 85.27±15.91* | 135.65±30.12*# | <0.001 |
LVEDVI (mL) | 57.80±10.66 | 58.65±11.81 | 74.45±16*# | <0.001 |
E peak (m/s) | 0.74±0.18 | 0.71±0.18 | 0.81±0.27*# | 0.013 |
A peak (m/s) | 0.62±0.16 | 0.83±0.19* | 0.93±0.23*# | <0.001 |
e’ average TDI (cm/s) | 10.51±2.19 | 7.89±2.09* | 6.3±2.17*# | <0.001 |
E/e’, mean | 7.13±1.62 | 9.45±2.92* | 13.56±4.77*# | <0.001 |
DT (seconds) | 192.30±28.23 | 205.35±40.3 | 206.28±43.31 | 0.078 |
GLS (%) | 19.58±2.25 | 17.42±1.91* | 16.7±2.5*# | <0.001 |
LAVImax (mL) | 21.41±5.54 | 23.53±8.45 | 36.27±14.3*# | <0.001 |
LAVIpre (mL) | 13.63±4.34 | 15.37±6.08 | 25.58±11*# | <0.001 |
LAVImin (mL) | 8.58±3.05 | 10.03±4.04 | 17.12±8.42*# | <0.001 |
LAVIt (mL) | 12.83±3.40 | 13.5±5.35 | 19.15±7.35*# | <0.001 |
LAVtEF (%) | 60.27±7.80 | 57.16±7.93 | 53.68±8.78*# | <0.001 |
LAVIp (mL) | 7.78±2.30 | 8.15±3.83 | 10.69±4.94*# | <0.001 |
LAVpEF (%) | 36.58±8.22 | 34.47±9.35 | 30.05±8.84* | <0.001 |
CVI (mL) | 26.86±6.99 | 26.63±7.28 | 30.02±9.68*# | 0.013 |
LAVIa (mL) | 5.05±1.93 | 5.35±2.71 | 8.46±4.03*# | <0.001 |
LAVaEF (%) | 37.05±9.63 | 34.51±8.72 | 33.76±9.48* | 0.079 |
Continuous data are expressed as the mean ± standard deviation. *, P<0.05 indicates significant difference from CON group; #, P<0.05 indicates significant difference from CKDN-LVH group. CON, control; CKD, chronic kidney disease; LVH, left ventricular hypertrophy; N-LVH, non-LVH; LVEF, left ventricular ejection fraction; LVMI, left ventricular mass index; LVEDVI, left ventricular end-diastolic volume index; E, early diastolic peak flow velocity; A, late diastolic peak flow velocity; e’, tissue Doppler early diastolic peak velocity; TDI, tissue Doppler imaging; DT, mitral early diastolic E peak deceleration time; GLS, left ventricular global longitudinal strain; LAVI, left atrial volume index; LAVImax, left atrial maximum volume index; LAVIpre, left atrial presystolic volume index; LAVImin, left atrial minimum volume index; LAVIt, left atrial total emptying volume index; LAVtEF, left atrial total emptying fraction; LAVIp, left atrial passive emptying volume index; LAVpEF, left atrial passive emptying fraction; CVI, conduit volume index; LAVIa, left atrial active emptying volume index; LAVaEF, left atrial active emptying fraction.
LA strain and strain rate analysis
Strain and strain rate were lower in the CKD group compared to the CON group. Specifically, LA Ss, LA Se, LA SRs, LA SRe, and LA SRa were even further reduced in the CKDLVH group as compared to the CKDN-LVH group (P<0.05). Figure 3 illustrates the pattern of LA strain and strain rate in three patients from different study groups, with detailed data provided in Figure 4 and Table S1.
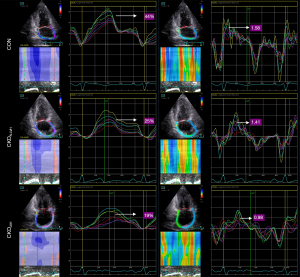
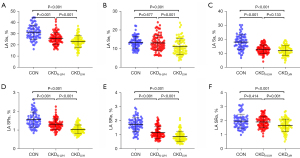
Regression analysis
The univariate correlations of LA strain and strain rate in the study population are presented in Tables 3,4. LVMI showed a negative correlation with LA Ss, LA Se, LA Sa, LA SRs, LA SRe, and LA SRa. LA strain and strain rate were negatively correlated with age, SBP, A peak, E/e’, and LAVI and were positively correlated with GFR and e’. Multivariate analysis confirmed that LVMI had an independent negative effect on all LA strain and strain rates (Table 5).
Table 3
Variables | LA Ss | LA Se | LA Sa | |||||
---|---|---|---|---|---|---|---|---|
R | P | R | P | R | P | |||
Age | −0.36 | <0.001 | −0.44 | <0.001 | −0.12 | 0.076 | ||
SBP | −0.38 | <0.001 | −0.19 | 0.004 | −0.37 | <0.001 | ||
DBP | −0.17 | 0.008 | −0.07 | 0.257 | −0.30 | <0.001 | ||
BMI | −0.12 | 0.133 | −0.11 | 0.142 | −0.05 | 0.569 | ||
BSA | −0.14 | 0.034 | −0.09 | 0.170 | −0.11 | 0.080 | ||
HR | −0.01 | 0.906 | −0.06 | 0.399 | −0.04 | 0.573 | ||
GFR | 0.50 | <0.001 | 0.20 | 0.002 | 0.52 | <0.001 | ||
SCr | −0.25 | <0.001 | −0.09 | 0.185 | −0.27 | <0.001 | ||
BUN | −0.28 | <0.001 | −0.12 | 0.064 | −0.28 | <0.001 | ||
LVEF | 0.15 | 0.019 | 0.04 | 0.546 | 0.18 | 0.006 | ||
LVMI | −0.46 | <0.001 | −0.24 | <0.001 | −0.43 | <0.001 | ||
LVEDVI | −0.25 | <0.001 | −0.09 | 0.162 | −0.27 | <0.001 | ||
E peak | 0.06 | 0.399 | 0.16 | 0.017 | −0.06 | 0.378 | ||
A peak | −0.36 | <0.001 | −0.28 | <0.001 | −0.20 | 0.002 | ||
e’ | 0.58 | <0.001 | 0.44 | <0.001 | 0.43 | <0.001 | ||
E/e’ | −0.44 | <0.001 | −0.28 | <0.001 | −0.36 | <0.001 | ||
DT | −0.10 | 0.136 | −0.17 | 0.012 | 0.02 | 0.797 | ||
LAVImax | −0.35 | <0.001 | −0.17 | 0.011 | −0.35 | <0.001 | ||
LAVIpre | −0.44 | <0.001 | −0.27 | <0.001 | −0.38 | <0.001 | ||
LAVImin | −0.47 | <0.001 | −0.26 | <0.001 | −0.43 | <0.001 |
SBP, systolic blood pressure; DBP, diastolic blood pressure; BMI, body mass index; BSA, body surface area; HR, heart rate; GFR, glomerular filtration rate; SCr, serum creatinine; BUN, blood urea nitrogen; LVEF, left ventricular ejection fraction; LVMI, left ventricular mass index; LVEDVI, left ventricular end-diastolic volume index; E, early diastolic peak flow velocity; A, late diastolic peak flow velocity; e’, tissue Doppler early diastolic peak velocity; DT, mitral valve early diastolic E peak deceleration time; LAVImax, left atrial maximum volume index; LAVIpre, left atrial presystolic volume index; LAVImin, left atrial minimum volume index; LA Ss, left atrial global longitudinal strain during systole; LA Se, left atrial global longitudinal strain during early diastole; LA Sa, left atrial global longitudinal strain during late diastole.
Table 4
Variables | LA SRs | LA SRe | LA SRa | |||||
---|---|---|---|---|---|---|---|---|
R | P | R | P | R | P | |||
Age | −0.24 | <0.001 | −0.52 | <0.001 | −0.10 | 0.142 | ||
SBP | −0.43 | <0.001 | −0.51 | <0.001 | −0.14 | 0.032 | ||
DBP | −0.18 | 0.006 | −0.21 | 0.001 | 0.00 | 0.981 | ||
BMI | −0.21 | 0.007 | −0.13 | 0.085 | −0.08 | 0.333 | ||
BSA | −0.03 | 0.686 | −0.13 | 0.043 | 0.05 | 0.934 | ||
HR | 0.20 | 0.003 | −0.07 | 0.265 | 0.33 | <0.001 | ||
GFR | 0.50 | <0.001 | 0.64 | <0.001 | 0.20 | 0.002 | ||
SCr | −0.31 | <0.001 | −0.40 | <0.001 | −0.11 | 0.107 | ||
BUN | −0.32 | <0.001 | −0.44 | <0.001 | −0.13 | 0.045 | ||
LVEF | 0.15 | <0.018 | 0.02 | 0.711 | 0.23 | <0.001 | ||
LVMI | −0.55 | <0.001 | −0.48 | <0.001 | −0.42 | <0.001 | ||
LVEDVI | −0.42 | <0.001 | −0.30 | <0.001 | −0.34 | <0.001 | ||
E peak | −0.12 | 00.065 | 0.17 | 0.008 | −0.34 | <0.001 | ||
A peak | −0.34 | <0.001 | −0.61 | <0.001 | 0.00 | 0.998 | ||
e’ | 0.54 | <0.001 | 0.82 | <0.001 | 0.13 | 0.045 | ||
E/e’ | −0.54 | <0.001 | −0.53 | <0.001 | −0.39 | <0.001 | ||
DT | −0.10 | 0.144 | −0.27 | <0.001 | 0.04 | 0.600 | ||
LAVImax | −0.52 | <0.001 | −0.40 | <0.001 | −0.43 | <0.001 | ||
LAVIpre | −0.56 | <0.001 | −0.49 | <0.001 | −0.45 | <0.001 | ||
LAVImin | −0.57 | <0.001 | −0.45 | <0.001 | −0.51 | <0.001 |
SBP, systolic blood pressure; DBP, diastolic blood pressure; BMI, body mass index; BSA, body surface area; HR, heart rate; GFR, glomerular filtration rate; SCr, serum creatinine; BUN, blood urea nitrogen; LVEF, left ventricular ejection fraction; LVMI, left ventricular mass index; LVEDVI, left ventricular end-diastolic volume index; E, early diastolic peak flow velocity; A, late diastolic peak flow velocity; e’, tissue Doppler early diastolic peak velocity; DT, mitral valve early diastolic E peak deceleration time; LAVImax, left atrial maximum volume index; LAVIpre, left atrial presystolic volume index; LAVImin, left atrial minimum volume index; LA SRs, left atrial global longitudinal strain rate during systole; LA SRe, left atrial global longitudinal strain rate during early diastole; LA SRa, left atrial global longitudinal strain rate during late diastole.
Table 5
Variables | Model 1 | Model 2 | Model 3 | |||||
---|---|---|---|---|---|---|---|---|
β | P | β | P | β | P | |||
LA Ss | ||||||||
LVMI | −0.29 | <0.001 | −0.21 | <0.001 | −0.20 | 0.001 | ||
Age | −0.34 | <0.001 | – | – | −0.35 | <0.001 | ||
SBP | 0.02 | 0.797 | – | – | 0.08 | 0.923 | ||
A peak | – | – | 0.06 | 0.441 | 0.20 | 0.010 | ||
E/e’ | – | – | 0.13 | 0.431 | 0.24 | 0.880 | ||
LAVI | – | – | −0.14 | 0.080 | −0.13 | 0.088 | ||
LA Se | ||||||||
LVMI | −0.20 | 0.001 | −0.08 | 0.416 | −0.16 | 0.113 | ||
Age | −0.40 | <0.001 | – | – | −0.32 | <0.001 | ||
SBP | −0.12 | 0.211 | – | – | −0.06 | 0.548 | ||
A peak | – | – | −0.08 | 0.322 | −0.01 | 0.940 | ||
E/e’ | – | – | −0.26 | 0.184 | −0.14 | 0.453 | ||
LAVI | – | – | −0.08 | 0.399 | −0.08 | 0.358 | ||
LA Sa | ||||||||
LVMI | −0.25 | <0.001 | −0.26 | <0.001 | −0.13 | 0.040 | ||
Age | −0.11 | 0.079 | – | – | −0.21 | 0.003 | ||
SBP | 0.13 | 0.134 | – | – | 0.06 | 0.493 | ||
A peak | – | – | 0.15 | 0.025 | 0.29 | 0.001 | ||
E/e’ | – | – | 0.40 | 0.108 | 0.16 | 0.370 | ||
LAVI | – | – | −0.13 | 0.141 | −0.11 | 0.184 | ||
LA SRs | – | – | – | – | – | – | ||
LVMI | −0.42 | <0.001 | −0.20 | 0.009 | −0.20 | 0.009 | ||
Age | −0.15 | 0.010 | – | – | −0.15 | 0.027 | ||
SBP | −0.12 | 0.167 | – | – | −0.05 | 0.590 | ||
A peak | – | – | 0.09 | 0.222 | 0.16 | 0.051 | ||
E/e’ | – | – | 0.01 | 0.929 | −0.05 | 0.777 | ||
LAVI | – | – | −0.23 | 0.004 | −0.23 | 0.004 | ||
LA SRe | ||||||||
LVMI | −0.16 | <0.001 | −0.10 | 0.108 | −0.06 | 0.311 | ||
Age | −0.46 | <0.001 | – | – | −0.24 | <0.001 | ||
SBP | −0.08 | 0.235 | – | – | 0.05 | 0.390 | ||
A peak | – | – | −0.24 | <0.001 | −0.14 | 0.012 | ||
E/e’ | – | – | 0.27 | 0.018 | 0.18 | 0.097 | ||
LAVI | – | – | −0.15 | 0.007 | −0.14 | 0.011 | ||
LA SRa | ||||||||
LVMI | −0.46 | <0.001 | −0.18 | 0.028 | −0.20 | 0.011 | ||
Age | −0.04 | 0.529 | – | – | −0.29 | <0.001 | ||
SBP | 0.05 | 0.619 | – | – | 0.01 | 0.890 | ||
A peak | – | – | 0.35 | <0.001 | 0.44 | <0.001 | ||
E/e’ | – | – | −0.19 | 0.296 | −0.24 | 0.184 | ||
LAVI | – | – | −0.21 | 0.018 | −0.22 | 0.013 |
Model 1: adjustment for age, systolic blood pressure (SBP), diastolic blood pressure (DBP), body surface area (BSA), glomerular filtration rate (GFR), and blood urea nitrogen (BUN); Model 2: adjustment for early diastolic peak flow velocity (E peak), late diastolic peak flow velocity (A peak), tissue Doppler early diastolic peak velocity (e’), E/e’, mitral valve early diastolic E peak deceleration time (DT), left ventricular global longitudinal strain (LV GLS), left ventricular ejection fraction (LVEF), left ventricular end-diastolic volume index (LVEDVI), and left atrial volume index(LAVI); Model 3: including all parameters of model 1 and model 2. LA Ss, left atrial global longitudinal strain during systole; LA Se, left atrial global longitudinal strain during early diastole; LA Sa, left atrial global longitudinal strain during late diastole; LA SRs, left atrial global longitudinal strain rate during systole; LA SRe, left atrial global longitudinal strain rate during early diastole; LA SRa, left atrial global longitudinal strain rate during late diastole; LVMI, left ventricular mass index; SBP, systolic blood pressure; E, early diastolic peak flow velocity; A, late diastolic peak flow velocity; e’, tissue Doppler early diastolic peak velocity; LAVI, left atrial volume index.
Intraobserver and interobserver variability analysis
Intraobserver and interobserver agreement were evaluated for each LA strain and strain rate indicator. The intraobserver ICC values for LA Ss, LA Se, LA Sa, LA SRs, LA SRe, and LA SRa were 0.948 [95% confidence interval (CI): 0.873–0.979], 0.961 (95% CI: 0.905–0.985), 0.902 (95% CI: 0.769–0.960), 0.814 (95% CI: 0.588–0.922), 0.920 (95% CI: 0.809–0.967), and 0.874 (95% CI: 0.709–0.948), respectively (Figure 5A-5F). The interobserver ICC values were 0.918 (95% CI: 0.806–0.967), 0.972 (95% CI: 0.932–0.989), 0.833 (95% CI: 0.626–0.931), 0.858 (95% CI: 0.676–0.941), 0.947 (95% CI: 0.871–0.979), and 0.869 (95% CI: 0.699–0.946), respectively (Figure 5G-5L). All parameters had intra- and interobserver ICC values above 0.75, indicating good repeatability.
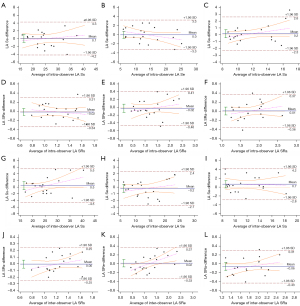
Discussion
Principal findings
This study primarily focused on using LA strain and strain rate techniques to evaluate LA function in patients with CKD. Several key findings emerged. First, conventional ultrasound did not show abnormal LA volume in the CKDN-LVH group compared to the CON group, but strain and strain rate indices were changed, with reductions in all except LA Se and LA SRa. The LA strain and strain rate were further reduced in the CKDLVH group compared to the CKDN-LVH group. These metrics were negatively correlated with age, SBP, A peak, E/e’, LAVI, and LVMI but positively correlated with GFR and e’. LVMI had an independent negative impact on all LA strain and strain rates (26).
The role of LA volume in CKD
The study found that LA volume was increased in the CKDLVH group compared to the CKDN-LVH group, with no significant difference between the CKDN-LVH group and the CON group. This suggests that LAVI is closely associated with LVH in patients with CKD, as LVH is a common change in uremic cardiomyopathy and leads to elevated LAVI (27). LAVI is also an independent risk factor for cardiovascular events in patients with CKD (28,29). In a study involving 49 patients with CKD (stages 3–5), LAVI was significantly higher in the CKD group relative to LVMI and was a predictor of adverse cardiac events. Moreover, patients with LAVI >32 mL/m2 have significantly lower event-free survival than those with normal (<28 mL/m2) or mildly dilated (28–32 mL/m2) LAVI (30). Another study involving 210 nondialysis patients with CKD (stages 4–5) found that LAVI assessed by TTE was independently associated with new-onset atrial fibrillation (31). Atrial fibrillation is linked to an increased risk of stroke, congestive heart failure, myocardial infarction, CKD progression, and death in patients with CKD (32,33). LAVI, similar to LA and LV strain analyses, is a reliable indicator of myocardial involvement in patients with CKD and is superior to E/e’ for evaluating LV diastolic function (34).
The role of LA strain and strain rate in CKD
The study found that LA volume in the CKDN-LVH group did not show significant changes, while patients in the CKDLVH group exhibited a notable increase in LA volume. However, LA strain and strain rate indicators were reduced in the CKD group, with the CKDLVH group showing even greater reductions compared to the CKDN-LVH group. This suggests that LA strain and strain rates are already abnormal in patients with CKD with normal LA volume, indicating that functional changes in the LA occur before structural changes. In a study of 33 patients with CKD (stage 3), both global systolic strain (GS) and strain rate of the left atrium were reduced in the CKD group, despite the LAVI being similar in the CKD group combined with hypertension (HT) and the HT group. This implies that changes in LA function may precede LA enlargement even in disease states (35). LA GS and strain rate may serve as more sensitive noninvasive tools for detecting cardiovascular involvement in CKD. Thus, using strain and strain rate parameters to evaluate LA function is feasible, and these parameters have become sensitive indicators for assessing LA function (36-38). LA strain is increasingly being adopted as a surrogate for LV diastolic dysfunction (39).
The LA strain index also predicts poor outcomes in patients with CKD. In a study of 76 patients with CKD (stage 3), LA GS was the most sensitive predictor of CKD presence, outperforming LAVI. Adding LA strain to conventional echocardiographic parameters significantly improved the ability to detect cardiovascular lesions (C statistic value =0.65 vs. C-statistic value =0.84; P<0.001). Another study reported that increased LAVI, decreased LV GLS, and presence of CKD were independent predictors of LA strain, while LVMI, E/e’ ratio, and CKD were predictors of LAVI (16). In a study involving 243 patients with CKD (stages 3–4), reduced LA strain rate was found to be an independent predictor of cardiovascular death and major adverse cardiovascular events (MACEs). Multivariate regression analysis of univariate predictors identified LA strain rate as the only independent predictor of primary and secondary endpoints. Receiver operating characteristic curve analysis indicated that LA strain rate was a stronger predictor of adverse events [area under the curve (AUC) =0.84] compared to the Framingham (AUC =0.58) and atherosclerotic CVD (AUC =0.59) risk scores (40).
Relationship between LVMI and LA function
In our study, LVMI was negatively correlated with LA strain and strain rate. Additionally, LA strain and strain rate were negatively correlated with age, SBP, A peak, E/e’, and LAVI and was positively correlated with GFR and e’. Multivariate analysis confirmed that LVMI had an independent negative impact on all LA strain and strain rates.
LVH is a risk factor for cardiac disease and all-cause mortality (41,42). It is common in CKD, with its incidence increasing as renal function declines (43). Factors contributing to LVH in patients with CKD include HT, phosphate metabolism disorders, uremic toxins, and chronic inflammation (44-46). LVH involves specific remodeling changes at both the macro- and microlevels, including the activation of apoptotic signaling and metabolic pathways. These changes can increase extracellular matrix production and lead to intercellular fibrosis in cardiomyocytes, resulting in systolic and diastolic dysfunction (3) and the formation of arrhythmogenic substrates (47). LVH is strongly associated with LA volume and function (48,49). Increased LA stiffness (50) and fibrosis (51) in patients with CKD reduce LA contraction function, leading to an enlarged atrial volume.
Study limitations
There are several limitations to this study that should be addressed. First, we employed a prospective design, and the follow-up portion of this study is ongoing and will be completed in the future. However, due to incomplete follow-up data, statistical analysis of a segment of the data has not been conducted. Therefore, the prognostic significance of the findings is unclear and needs to be verified by further studies. Second, participants were only enrolled from a single center and constituted a relatively small sample size. Therefore, a study with a larger sample size to validate our findings is currently underway. Third, strain echocardiographic imaging involves a number of drawbacks, such as intervendor variability, the dependence on the operator’s experience, differences in frame rate and loading conditions, and external mechanical factors (52-55). Fourth, the study did not stage CKD, which could have affected the statistical results for patients with different CKD stages. Fifth, EchoPAC does not yet have a speckle-tracking technique specifically designed for LA studies. Consequently, a speckle-tracking technique designed for the LV was used. However, earlier studies have confirmed that it is feasible to measure LA strain using this software (51,56,57).
Conclusions
LA strain and strain rate are valuable indicators for detecting early changes in LA function in patients with CKD. LVMI, which has an independent negative effect on both LA strain and strain rate, is significantly correlated with these measures and may also be a predictor of cardiovascular events.
Acknowledgments
None.
Footnote
Reporting Checklist: The authors have completed the STROBE reporting checklist. Available at https://qims.amegroups.com/article/view/10.21037/qims-24-1537/rc
Funding: This research was funded by
Conflicts of Interest: All authors have completed the ICMJE uniform disclosure form (available at https://qims.amegroups.com/article/view/10.21037/qims-24-1537/coif). The authors have no conflicts of interest to declare.
Ethical Statement: The authors are accountable for all aspects of the work in ensuring that questions related to the accuracy or integrity of any part of the work are appropriately investigated and resolved. The study was conducted in accordance with the Declaration of Helsinki (as revised in 2013). The study was approved by the Provincial Ethics Committee of Shenzhen People’s Hospital (No. LL-KY-2021282). All individuals provided written informed consent to participate in this prospective study.
Open Access Statement: This is an Open Access article distributed in accordance with the Creative Commons Attribution-NonCommercial-NoDerivs 4.0 International License (CC BY-NC-ND 4.0), which permits the non-commercial replication and distribution of the article with the strict proviso that no changes or edits are made and the original work is properly cited (including links to both the formal publication through the relevant DOI and the license). See: https://creativecommons.org/licenses/by-nc-nd/4.0/.
References
- Sarnak MJ, Levey AS, Schoolwerth AC, Coresh J, Culleton B, Hamm LL, McCullough PA, Kasiske BL, Kelepouris E, Klag MJ, Parfrey P, Pfeffer M, Raij L, Spinosa DJ, Wilson PWAmerican Heart Association Councils on Kidney in Cardiovascular Disease, High Blood Pressure Research, Clinical Cardiology, and Epidemiology and Prevention. Kidney disease as a risk factor for development of cardiovascular disease: a statement from the American Heart Association Councils on Kidney in Cardiovascular Disease, High Blood Pressure Research, Clinical Cardiology, and Epidemiology and Prevention. Circulation 2003;108:2154-69. [Crossref] [PubMed]
- GBD Chronic Kidney Disease Collaboration. Global, regional, and national burden of chronic kidney disease, 1990-2017: a systematic analysis for the Global Burden of Disease Study 2017. Lancet 2020;395:709-33. [Crossref] [PubMed]
- Segall L, Nistor I, Covic A. Heart failure in patients with chronic kidney disease: a systematic integrative review. Biomed Res Int 2014;2014:937398. [Crossref] [PubMed]
- Elliott AD, Ariyaratnam J, Howden EJ, La Gerche A, Sanders P. Influence of exercise training on the left atrium: implications for atrial fibrillation, heart failure, and stroke. Am J Physiol Heart Circ Physiol 2023;325:H822-36. [Crossref] [PubMed]
- Bhatt A, Flink L, Lu DY, Fang Q, Bibby D, Schiller NB. Exercise physiology of the left atrium: quantity and timing of contribution to cardiac output. Am J Physiol Heart Circ Physiol 2021;320:H575-83. [Crossref] [PubMed]
- Thomas L, Marwick TH, Popescu BA, Donal E, Badano LP. Left Atrial Structure and Function, and Left Ventricular Diastolic Dysfunction: JACC State-of-the-Art Review. J Am Coll Cardiol 2019;73:1961-77. [Crossref] [PubMed]
- Triposkiadis F, Pieske B, Butler J, Parissis J, Giamouzis G, Skoularigis J, Brutsaert D, Boudoulas H. Global left atrial failure in heart failure. Eur J Heart Fail 2016;18:1307-20. [Crossref] [PubMed]
- Tripepi G, Benedetto FA, Mallamaci F, Tripepi R, Malatino L, Zoccali C. Left atrial volume monitoring and cardiovascular risk in patients with end-stage renal disease: a prospective cohort study. J Am Soc Nephrol 2007;18:1316-22. [Crossref] [PubMed]
- Sciacqua A, Perticone M, Tripepi G, Miceli S, Tassone EJ, Grillo N, Carullo G, Sesti G, Perticone F. Renal disease and left atrial remodeling predict atrial fibrillation in patients with cardiovascular risk factors. Int J Cardiol 2014;175:90-5. [Crossref] [PubMed]
- Chan MY, Wong HB, Ong HY, Yeo TC. Prognostic value of left atrial size in chronic kidney disease. Eur J Echocardiogr 2008;9:736-40. [Crossref] [PubMed]
- Kimura K, Takenaka K, Ebihara A, Okano T, Uno K, Fukuda N, Ando J, Fujita H, Morita H, Yatomi Y, Nagai R. Speckle tracking global strain rate E/E' predicts LV filling pressure more accurately than traditional tissue Doppler E/E'. Echocardiography 2012;29:404-10. [Crossref] [PubMed]
- Amundsen BH, Helle-Valle T, Edvardsen T, Torp H, Crosby J, Lyseggen E, Støylen A, Ihlen H, Lima JA, Smiseth OA, Slørdahl SA. Noninvasive myocardial strain measurement by speckle tracking echocardiography: validation against sonomicrometry and tagged magnetic resonance imaging. J Am Coll Cardiol 2006;47:789-93. [Crossref] [PubMed]
- Pastore MC, De Carli G, Mandoli GE, D'Ascenzi F, Focardi M, Contorni F, Mondillo S, Cameli M. The prognostic role of speckle tracking echocardiography in clinical practice: evidence and reference values from the literature. Heart Fail Rev 2021;26:1371-81. [Crossref] [PubMed]
- Morariu VI, Arnautu DA, Morariu SI, Popa AM, Parvanescu T, Andor M, Abhinav S, David VL, Ionescu A, Tomescu MC. 2D speckle tracking: a diagnostic and prognostic tool of paramount importance. Eur Rev Med Pharmacol Sci 2022;26:3903-10. [Crossref] [PubMed]
- Pastore MC, Mandoli GE, Contorni F, Cavigli L, Focardi M, D'Ascenzi F, Patti G, Mondillo S, Cameli M. Speckle Tracking Echocardiography: Early Predictor of Diagnosis and Prognosis in Coronary Artery Disease. Biomed Res Int 2021;2021:6685378. [Crossref] [PubMed]
- Kadappu KK, Abhayaratna K, Boyd A, French JK, Xuan W, Abhayaratna W, Thomas L. Independent Echocardiographic Markers of Cardiovascular Involvement in Chronic Kidney Disease: The Value of Left Atrial Function and Volume. J Am Soc Echocardiogr 2016;29:359-67. [Crossref] [PubMed]
- Saito M, Okayama H, Yoshii T, Higashi H, Morioka H, Hiasa G, Sumimoto T, Inaba S, Nishimura K, Inoue K, Ogimoto A, Shigematsu Y, Hamada M, Higaki J. Clinical significance of global two-dimensional strain as a surrogate parameter of myocardial fibrosis and cardiac events in patients with hypertrophic cardiomyopathy. Eur Heart J Cardiovasc Imaging 2012;13:617-23. [Crossref] [PubMed]
- McIntyre CW, Odudu A, Eldehni MT. Cardiac assessment in chronic kidney disease. Curr Opin Nephrol Hypertens 2009;18:501-6. [Crossref] [PubMed]
- Park M, Hsu CY, Li Y, Mishra RK, Keane M, Rosas SE, Dries D, Xie D, Chen J, He J, Anderson A, Go AS, Shlipak MGChronic Renal Insufficiency Cohort (CRIC) Study Group. Associations between kidney function and subclinical cardiac abnormalities in CKD. J Am Soc Nephrol 2012;23:1725-34. [Crossref] [PubMed]
- Liu YW, Su CT, Huang YY, Yang CS, Huang JW, Yang MT, Chen JH, Tsai WC. Left ventricular systolic strain in chronic kidney disease and hemodialysis patients. Am J Nephrol 2011;33:84-90. [Crossref] [PubMed]
- Stevens PE, Levin A. Kidney Disease: Improving Global Outcomes Chronic Kidney Disease Guideline Development Work Group Members. Evaluation and management of chronic kidney disease: synopsis of the kidney disease: improving global outcomes 2012 clinical practice guideline. Ann Intern Med 2013;158:825-30. [Crossref] [PubMed]
- ESH/ESC Task Force for the Management of Arterial Hypertension. 2013 Practice guidelines for the management of arterial hypertension of the European Society of Hypertension (ESH) and the European Society of Cardiology (ESC): ESH/ESC Task Force for the Management of Arterial Hypertension. J Hypertens 2013;31:1925-38. [Crossref] [PubMed]
- Teichholz LE, Kreulen T, Herman MV, Gorlin R. Problems in echocardiographic volume determinations: echocardiographic-angiographic correlations in the presence of absence of asynergy. Am J Cardiol 1976;37:7-11. [Crossref] [PubMed]
- Lang RM, Badano LP, Mor-Avi V, Afilalo J, Armstrong A, Ernande L, Flachskampf FA, Foster E, Goldstein SA, Kuznetsova T, Lancellotti P, Muraru D, Picard MH, Rietzschel ER, Rudski L, Spencer KT, Tsang W, Voigt JU. Recommendations for cardiac chamber quantification by echocardiography in adults: an update from the American Society of Echocardiography and the European Association of Cardiovascular Imaging. Eur Heart J Cardiovasc Imaging 2015;16:233-70. [Crossref] [PubMed]
- Toutouzas K, Trikas A, Pitsavos C, Barbetseas J, Androulakis A, Stefanadis C, Toutouzas P. Echocardiographic features of left atrium in elite male athletes. Am J Cardiol 1996;78:1314-7. [Crossref] [PubMed]
- Hussain K, Nso N, Tsourdinis G, Haider S, Mian R, Sanagala T, Erwin JP 3rd, Pursnani A. A systematic review and meta-analysis of left atrial strain in hypertrophic cardiomyopathy and its prognostic utility. Curr Probl Cardiol 2024;49:102146. [Crossref] [PubMed]
- Mark PB, Johnston N, Groenning BA, Foster JE, Blyth KG, Martin TN, Steedman T, Dargie HJ, Jardine AG. Redefinition of uremic cardiomyopathy by contrast-enhanced cardiac magnetic resonance imaging. Kidney Int 2006;69:1839-45. [Crossref] [PubMed]
- Parfrey PS, Foley RN, Harnett JD, Kent GM, Murray DC, Barre PE. Outcome and risk factors for left ventricular disorders in chronic uraemia. Nephrol Dial Transplant 1996;11:1277-85.
- Levy D, Garrison RJ, Savage DD, Kannel WB, Castelli WP. Prognostic implications of echocardiographically determined left ventricular mass in the Framingham Heart Study. N Engl J Med 1990;322:1561-6. [Crossref] [PubMed]
- Hee L, Nguyen T, Whatmough M, Descallar J, Chen J, Kapila S, French JK, Thomas L. Left atrial volume and adverse cardiovascular outcomes in unselected patients with and without CKD. Clin J Am Soc Nephrol 2014;9:1369-76. [Crossref] [PubMed]
- Hakamäki M, Hellman T, Lankinen R, Koivuviita N, Pärkkä J, Kallio P, Kiviniemi T, Airaksinen KEJ, Järvisalo MJ, Metsärinne K. Elevated Troponin T and Enlarged Left Atrium Are Associated with the Incidence of Atrial Fibrillation in Patients with CKD Stage 4-5. Nephron 2021;145:71-7. [Crossref] [PubMed]
- Bansal N, Xie D, Sha D, Appel LJ, Deo R, Feldman HI, He J, Jamerson K, Kusek JW, Messe S, Navaneethan SD, Rahman M, Ricardo AC, Soliman EZ, Townsend R, Go AS. Cardiovascular Events after New-Onset Atrial Fibrillation in Adults with CKD: Results from the Chronic Renal Insufficiency Cohort (CRIC) Study. J Am Soc Nephrol 2018;29:2859-69. [Crossref] [PubMed]
- Bansal N, Fan D, Hsu CY, Ordonez JD, Marcus GM, Go AS. Incident atrial fibrillation and risk of end-stage renal disease in adults with chronic kidney disease. Circulation 2013;127:569-74. [Crossref] [PubMed]
- Bokhari SR, Mansur A, Khan Assir MZ, Ittifaq A, Sarwar S. Echocardiographic Evaluation of Left Atrial Volume Index in Patients with Chronic Kidney Disease. Saudi J Kidney Dis Transpl 2020;31:750-8. [Crossref] [PubMed]
- Kadappu KK, Kuncoro AS, Hee L, Aravindan A, Spicer ST, Suryanarayanan G, Xuan W, Boyd A, French JK, Thomas L. Chronic kidney disease is independently associated with alterations in left atrial function. Echocardiography 2014;31:956-64. [Crossref] [PubMed]
- Di Salvo G, Caso P, Lo Piccolo R, Fusco A, Martiniello AR, Russo MG, D'Onofrio A, Severino S, Calabró P, Pacileo G, Mininni N, Calabró R. Atrial myocardial deformation properties predict maintenance of sinus rhythm after external cardioversion of recent-onset lone atrial fibrillation: a color Doppler myocardial imaging and transthoracic and transesophageal echocardiographic study. Circulation 2005;112:387-95. [Crossref] [PubMed]
- Inaba Y, Yuda S, Kobayashi N, Hashimoto A, Uno K, Nakata T, Tsuchihashi K, Miura T, Ura N, Shimamoto K. Strain rate imaging for noninvasive functional quantification of the left atrium: comparative studies in controls and patients with atrial fibrillation. J Am Soc Echocardiogr 2005;18:729-36. [Crossref] [PubMed]
- Boyd AC, Richards DA, Marwick T, Thomas L. Atrial strain rate is a sensitive measure of alterations in atrial phasic function in healthy ageing. Heart 2011;97:1513-9. [Crossref] [PubMed]
- Sitges M, Ajmone Marsan N, Cameli M, D'Andrea A, Carvalho RF, Holte E, Michalski B, Podlesnikar T, Popescu BA, Schulz-Menger J, Stankovic I, Haugaa KH, Dweck MR. EACVI survey on the evaluation of left ventricular diastolic function. Eur Heart J Cardiovasc Imaging 2021;22:1098-105. [Crossref] [PubMed]
- Gan GCH, Kadappu KK, Bhat A, Fernandez F, Gu KH, Cai L, Byth K, Eshoo S, Thomas L. Left Atrial Strain Is the Best Predictor of Adverse Cardiovascular Outcomes in Patients with Chronic Kidney Disease. J Am Soc Echocardiogr 2021;34:166-75. [Crossref] [PubMed]
- Charytan D. Is left ventricular hypertrophy a modifiable risk factor in end-stage renal disease. Curr Opin Nephrol Hypertens 2014;23:578-85. [Crossref] [PubMed]
- Lewis AA, Ayers CR, Selvin E, Neeland I, Ballantyne CM, Nambi V, Pandey A, Powell-Wiley TM, Drazner MH, Carnethon MR, Berry JD, Seliger SL, DeFilippi CR, de Lemos JA. Racial Differences in Malignant Left Ventricular Hypertrophy and Incidence of Heart Failure: A Multicohort Study. Circulation 2020;141:957-67. [Crossref] [PubMed]
- Levin A, Singer J, Thompson CR, Ross H, Lewis M. Prevalent left ventricular hypertrophy in the predialysis population: identifying opportunities for intervention. Am J Kidney Dis 1996;27:347-54. [Crossref] [PubMed]
- Gansevoort RT, Correa-Rotter R, Hemmelgarn BR, Jafar TH, Heerspink HJ, Mann JF, Matsushita K, Wen CP. Chronic kidney disease and cardiovascular risk: epidemiology, mechanisms, and prevention. Lancet 2013;382:339-52. [Crossref] [PubMed]
- London GM. Cardiovascular disease in chronic renal failure: pathophysiologic aspects. Semin Dial 2003;16:85-94. [Crossref] [PubMed]
- Chan CT, Arab S, Carasso S, Moravsky G, Li GH, Liu PP, Rakowski H. Impact of frequent nocturnal hemodialysis on myocardial mechanics and cardiomyocyte gene expression. Circ Cardiovasc Imaging 2012;5:474-80. [Crossref] [PubMed]
- Chiu DY, Sinha S, Kalra PA, Green D. Sudden cardiac death in haemodialysis patients: preventative options. Nephrology (Carlton) 2014;19:740-9. [Crossref] [PubMed]
- Glassock RJ, Pecoits-Filho R, Barberato SH. Left ventricular mass in chronic kidney disease and ESRD. Clin J Am Soc Nephrol 2009;4:S79-91. [Crossref] [PubMed]
- Nakanishi K, Jin Z, Russo C, Homma S, Elkind MS, Rundek T, Tugcu A, Sacco RL, Di Tullio MR. Association of chronic kidney disease with impaired left atrial reservoir function: A community-based cohort study. Eur J Prev Cardiol 2017;24:392-8. [Crossref] [PubMed]
- Hassanin N, Alkemary A. Detection of Left Atrium Myopathy Using Two-Dimensional Speckle Tracking Echocardiography in Patients with End-Stage Renal Disease on Dialysis Therapy. Echocardiography 2016;33:233-41. [Crossref] [PubMed]
- Antoni ML, ten Brinke EA, Atary JZ, Marsan NA, Holman ER, Schalij MJ, Bax JJ, Delgado V. Left atrial strain is related to adverse events in patients after acute myocardial infarction treated with primary percutaneous coronary intervention. Heart 2011;97:1332-7. [Crossref] [PubMed]
- Mirea O, Pagourelias ED, Duchenne J, Bogaert J, Thomas JD, Badano LP, Voigt JU. EACVI-ASE-Industry Standardization Task Force. Intervendor Differences in the Accuracy of Detecting Regional Functional Abnormalities: A Report From the EACVI-ASE Strain Standardization Task Force. JACC Cardiovasc Imaging 2018;11:25-34. [Crossref] [PubMed]
- Negishi T, Negishi K, Thavendiranathan P, Cho GY, Popescu BA, Vinereanu D, Kurosawa K, Penicka M, Marwick TH. SUCCOUR Investigators. Effect of Experience and Training on the Concordance and Precision of Strain Measurements. JACC Cardiovasc Imaging 2017;10:518-22. [Crossref] [PubMed]
- Rösner A, Barbosa D, Aarsæther E, Kjønås D, Schirmer H, D'hooge J. The influence of frame rate on two-dimensional speckle-tracking strain measurements: a study on silico-simulated models and images recorded in patients. Eur Heart J Cardiovasc Imaging 2015;16:1137-47. [Crossref] [PubMed]
- Sonaglioni A, Nicolosi GL, Granato A, Bonanomi A, Rigamonti E, Lombardo M. Influence of chest wall conformation on reproducibility of main echocardiographic indices of left ventricular systolic function. Minerva Cardiol Angiol 2024;72:111-24. [Crossref] [PubMed]
- Cameli M, Caputo M, Mondillo S, Ballo P, Palmerini E, Lisi M, Marino E, Galderisi M. Feasibility and reference values of left atrial longitudinal strain imaging by two-dimensional speckle tracking. Cardiovasc Ultrasound 2009;7:6. [Crossref] [PubMed]
- Pagola J, González-Alujas T, Flores A, Muchada M, Rodriguez-Luna D, Seró L, Rubiera M, Boned S, Ribó M, Alvarez-Sabin J, Evangelista A, Molina CA. Left atria strain is a surrogate marker for detection of atrial fibrillation in cryptogenic strokes. Stroke 2014;45:e164-6. [Crossref] [PubMed]