Differences in plaque curvature in middle cerebral artery stenosis between acute stroke and non-acute stroke
Introduction
Intracranial atherosclerotic disease (ICAD) is a leading cause of ischemic stroke worldwide, and has a high prevalence among the Asian population (1,2). ICAD contributes to 30–50% of ischemic strokes in the Chinese population, and predominantly affects the middle cerebral artery (MCA) (2). In recent years, there has been a shift in the research focus on ICAD from the extent of stenosis to the vessel wall condition and plaque stability (3,4).
High-resolution magnetic resonance imaging (HR-MRI) is a non-invasive imaging method that can simultaneously display the degree of vascular stenosis, characteristics of plaques, and local changes in the vascular wall. Traditional parameters and features of plaques have already been reported by many scholars. For example, research has shown that symptomatic patients have a larger plaque area (PA), more positive remodeling (PR) vessels, and obvious enhanced plaques than asymptomatic patients (2,5-7). Further, using HR-MRI as a follow-up examination method, research has shown that plaque enhancement has a good predictive effect on stroke recurrence in ICAD patients (8,9). However, in addition to the characteristics of plaques, changes in hemodynamics at stenotic sites also have a significant effect on the occurrence and recurrence of stroke.
The computational fluid dynamics (CFD) model is a method used to obtain the hemodynamic parameters at the sites of vascular stenosis through imaging reconstruction (10,11). CFD model can be reconstructed based on computed tomography angiography or magnetic resonance angiography (MRA), and the primary hemodynamic parameter is wall shear stress (WSS). Previous studies have reported that high WSS in stenotic coronary arteries (12), carotid arteries (13), and intracranial arteries (10) is more likely to induce PR and atherosclerotic plaque rupture, triggering a cardiovascular event. However, in a previous study (14), we found that reconstructing the CFD model was time consuming. Woo et al. (15) obtained the value of WSS using GT software to process four-dimensional flow data; however, this method has not yet been widely implemented in practice, and its use in intracranial arteries is still not generally.
WSS change in the stenotic site is related to plaque morphology. Yang et al. (8) suggested that steepness (as a geometric morphological parameter and a reflection of the plaque curvature in the longitudinal direction) is a contributing factor to recurrent stroke. We conjectured that plaque steepness may indirectly reflect the luminal curvature, and may thus be associated with WSS. Therefore, we aimed to examine the potential and value of plaque curvature in the prediction of stroke occurrence. We hypothesized that a larger plaque curvature is more likely to cause ischemic stroke. We present this article in accordance with the STROBE reporting checklist (available at https://qims.amegroups.com/article/view/10.21037/qims-24-1552/rc).
Methods
Subjects and clinical data
The study was conducted in accordance with the Declaration of Helsinki (as revised in 2013). The research protocol was approved by the Ethics Committee of the Nanjing Medical University (No. NMU20200320). All enrolled patients provided verbal or written informed consent before they were examined.
From January 2020 to May 2024, 107 consecutive patients with suspected MCA atherosclerotic diseases were recruited from the Department of Neurology, Nanjing First Hospital, Nanjing, China. Patients experiencing drowsiness, dizziness, or limb weakness were recruited for the study if they met the following criteria: (I) had more than two atherosclerosis risk factors; (II) had no contraindications to magnetic resonance (MR) scans; (III) had unilateral stenosis in the M1 segment of the MCA (50–99% narrowing) on enhanced MRA imaging; (IV) had no ipsilateral internal carotid artery (ICA) stenosis ≥50%; (V) had no non-atherosclerotic vascular conditions, such as brain hemorrhage, vasculitis, moyamoya disease, dissection, or tumors; (VI) had no evidence of atrial fibrillation or cardioembolism; (VII) had high-quality images suitable for diagnosis and assessment; and (VIII) had an M1 segment of the MCA that ran straight.
A power analysis was conducted, and the sample size was determined using the “PS (Power and Sample Size Calculation software, Dupont and Plummer design)” to calculate the statistical power or sample size of our clinical study.
The demographic and clinical data of the patients were gathered from their medical records, including their sex, age, history of smoking (yes/no), alcoholism (yes/no), hypertension (yes/no), diabetes (yes/no), and blood glucose, glycated hemoglobin (HbA1c), total cholesterol, low-density lipoprotein (LDL), high-density lipoprotein (HDL), triglycerides, and homocysteine values. Patients with a history of past or current smoking were considered to have a cigarette smoking history; alcoholism was defined as consuming more than 2 U/day on average for men, or more than 1 U/day on average for women over the past year; hypertension was defined by a self-reported history of high blood pressure, average blood pressure levels >140/90 mmHg, or the use of antihypertensive medication; diabetes was identified by self-reported insulin or oral hypoglycemic medication usage, or recent physician-diagnosed diabetes based on elevated HbA1c (≥6.5%) (14).
Magnetic resonance imaging (MRI) process
After being admitted for a week, the enrolled patients underwent standard MRI procedures, including axial T1-weighted imaging (T1WI), T2-weighted imaging, T2-fluid attenuated inversion recovery, diffusion-weighted imaging (DWI), apparent diffusion coefficient (ADC) imaging, and enhanced MRA. The HR-MRI scans were performed on the stenotic MCA using a 3-Tesla MR scanner (Ingenia, Philips Medical Systems, Netherlands) with an 8-channel head coil. Subsequently, the following scan parameters were used to perform black-blood pre-contrast T1WI and contrast-enhanced T1WI (T1WI + C) imaging perpendicular to the M1 segment of the MCA: (I) pre-contrast T1WI: echo time (TE), 9 ms; repetition time (TR), 1,000 ms; slice thickness, 0.6 mm; slice gap, 0 mm; slice number, 10; number of excitions (NEX), 2; matrix size, 180 mm × 144 mm; field of view (FOV), 80 mm × 80 mm; and acquisition time, 192 s; and (II) T1WI + C: TE, 9 ms; TR, 1,000 ms; slice thickness, 0.6 mm; slice gap, 0 mm; slice number, 10; NEX, 2; matrix size, 180 mm × 144 mm; FOV, 80 mm × 80 mm; and acquisition time, 192 s. A 15 mL bolus of gadodiamide (Kang Chen, Guangzhou, China) was injected intravenously at a speed of 2 mL/s, T1WI+C was performed 5 minutes after the administration of the contrast agent.
Analysis of MR images
The vessel wall parameters were measured based on the images on a Philips Intellispace Portal workstation. The short axial T1WI images were enlarged to 300%, and manual measurements of the lumen area (LA) and vessel area (VA) at both the reference location and the most narrowed lumen (MNL) of the MCA were performed. The reference site was chosen as the nearest segment free of plaques or with minimal disease proximal to the stenosis in the MCA. In cases in which a proximal reference site was unavailable, the adjacent distal site was used. The following formulas were used: wall area (WA) = VA − LA; PA = WAMNL − WAreference; remodeling index (RI) = VAMNL/VAreference; normalized wall index (NWI) = WA/VA; degree of stenosis = (1 − LAMNL/LAreference) × 100%. The parameters were calculated according to previous studies (3,16).
Patients showing hyperintense signals on DWI and hypointense signals on the ADC in the stenotic MCA region were categorized as experiencing acute stroke, and assigned to the symptomatic group, while the remaining patients were assigned to the asymptomatic group. Patients with scattered cortical acute stroke lesions were allocated to the artery-to-artery embolism (AAE) group, and patients with a single subcortical lesion with a spatial relationship between the perforating arteries and the margin of plaque on HR-MRI were allocated to the local branch occlusion (LBO) group (15,17). A RI ≥1.05 was defined as PR, 0.95< RI <1.05 was defined as non-remodeling, and a RI ≤0.95 was defined as negative remodeling (NR) (3,16). Plaque enhancement was graded as follows: grade 0: enhancement equal to or less than normal arterial walls; grade 1: enhancement greater than grade 0 but less than the pituitary infundibulum; and grade 2: enhancement equal to or greater than that of the pituitary infundibulum. Grades 1 and 2 were considered enhanced in this study (17,18).
The height of plaques in most stenotic sites was defined as H2, the midpoint from the beginning of the plaques to H2 was H1, and the midpoint from H2 to the ending of the plaques was H3. R12 and R32 are the ratio of H1/H2 and H3/H2, respectively. Two experienced radiologists, who were blinded to the clinical information of the patients, conducted the measurements. Subsequently, the average value was determined and applied. Both observers reached a consensus on the slices selected for measurement and the degree of plaque enhancement. In cases of disagreement, a joint reading was performed to reach a consensus.
Statistical analysis
The statistical analyses were performed using the SPSS 21.0 software package (Chicago, IL, USA). The normality of the distribution of the quantitative data was assessed using the Kolmogorov-Smirnov test. Normally distributed continuous data are expressed as the mean ± standard deviation, while non-normally distributed data are presented as the median and inter-quartile range. The continuous variables were compared between groups using the t-test, while the categorical variables were compared using the Pearson chi-square test. Non-normally distributed data were analyzed using the Mann-Whitney U test. Subsequently, the statistically significant indicators underwent multivariate logistic regression analyses for further examination. A P value less than 0.05 was considered statistically significant. The degree of inter-observer consistency in measurements was assessed using intraclass correlation coefficients (ICC) with a two-way random effects model for continuous variables across different observers. An ICC exceeding 0.75 indicated robust reproducibility among the observers (7).
Results
Demographic and clinical characteristics of patients
After 35 patients were excluded (12 patients with moving artifacts; 18 patients with ipsilateral ICA stenosis ≥50%; 3 patients with brain tumors; and 2 patients with brain hemorrhages), 107 patients were enrolled in the study (Figure 1). There were 43 patients in the asymptomatic group (25 men, 18 women; mean age: 57±12 years), and 64 patients in the symptomatic group (33 men, 31 women; mean age: 68±10 years). No statistically significant differences were observed between the asymptomatic and symptomatic groups in terms of sex, smoking status, alcoholism, hypertension, diabetes mellitus, age, blood glucose levels, total cholesterol, triglycerides, HDL, LDL, HbA1c, and homocysteine levels (Table 1). More enhanced plaques were observed in the symptomatic group than the asymptomatic group (52 vs. 14, P=0.001). Of the patients in the symptomatic group, 31 were allocated to the AAE group, 25 to the LBO group, and 8 to the mixed-mechanism group.
Table 1
Variables | Asymptomatic group (n=43) | Symptomatic group (n=64) | P value |
---|---|---|---|
Sex (male) | 25 | 33 | 0.503a |
Smoker | 27 | 30 | 0.105a |
Alcoholism | 23 | 28 | 0.323a |
Hypertension | 30 | 39 | 0.349a |
Diabetes mellitus | 15 | 20 | 0.694a |
Enhanced plaques | 14 | 52 | 0.001*a |
Age (years) | 57±12 | 68±10 | 0.290b |
Blood glucose (mmol/L) | 5.87±1.69 | 6.58±1.45 | 0.462b |
Total cholesterol (mmol/L) | 4.66±1.34 | 4.79±1.27 | 0.685b |
Triglycerides (mmol/L) | 2.17±1.01 | 2.85±0.73 | 0.232b |
HDL (mmol/L) | 0.91±0.38 | 1.36±0.46 | 0.155b |
LDL (mmol/L) | 2.90±1.05 | 3.11±1.17 | 0.769b |
HbA1c (%) | 7.24±1.44 | 7.86±1.75 | 0.521b |
Homocysteine (µmol/L) | 12.05±4.17 | 14.33±3.64 | 0.184b |
Data are presented as the number or mean ± standard deviation. a, the P values were obtained using the Pearson chi-square test. b, the P value was obtained using the t-test. *, P<0.05. HDL, high-density lipoprotein; LDL, low-density lipoprotein; HbA1c, glycated hemoglobin.
Vessel wall and plaque quantitative data for the asymptomatic and symptomatic groups
The inter-observer agreement for the manual measurements and judgement of enhancement was strong, and exhibited a robust level of consistency, as reflected by an ICC ranging from 0.907–0.964 (P<0.001). The symptomatic group (Figure 2) had a larger PA (5.79±2.16 vs. 4.74±1.58, P=0.007), a larger RI (1.08±0.10 vs. 0.94±0.10, P<0.001), and more enhanced plaques (52 vs. 14, P=0.001) in most narrowed sites than the asymptomatic group (Figure 3). Additionally, the symptomatic group had a smaller H1 (1.42±0.33 vs. 1.96±0.49, P<0.001), H3 (1.57±0.36 vs. 1.95±0.42, P<0.001), R12 (P<0.001), and R32 (P<0.001) (Table 2 and Figure 4).
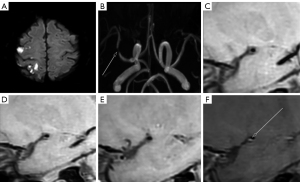
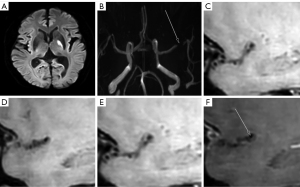
Table 2
Variables | Asymptomatic group (n=43) | Symptomatic group (n=64) | P value |
---|---|---|---|
Vessel areaMNL (mm2) | 14.21±2.89 | 14.46±2.89 | 0.725a |
Lumen areaMNL (mm2) | 2.84±0.84 | 2.37±0.81 | 0.006*a |
Vessel areareference (mm2) | 15.35±3.62 | 13.48±3.50 | 0.009*a |
Lumen areareference (mm2) | 8.70±2.50 | 7.20±1.95 | 0.001*a |
Wall areaMNL (mm2) | 11.38±2.33 | 12.08±3.54 | 0.259a |
Wall areareference (mm2) | 6.64±1.74 | 6.28±2.20 | 0.371a |
NWIMNL | 0.80±0.04 | 0.83±0.05 | 0.004*a |
NWIreference | 0.43±0.07 | 0.46±0.08 | 0.102a |
Plaque areaMNL (mm2) | 4.74±1.58 | 5.79±2.16 | 0.007*a |
Degree of stenosis (%) | 66.65±7.82 | 67.13±8.49 | 0.771a |
RI | 0.94±0.10 | 1.08±0.10 | <0.001*a |
H1 | 1.96±0.49 | 1.42±0.33 | <0.001*a |
H2 | 2.76±0.47 | 2.58±0.51 | 0.067a |
H3 | 1.95±0.42 | 1.57±0.36 | <0.001*a |
R12 | 0.73 (0.64–0.77) | 0.56 (0.48–0.62) | <0.001*b |
R32 | 0.71 (0.65–0.76) | 0.61 (0.54–0.70) | <0.001*b |
Data are expressed as the mean ± standard deviation or the median and inter-quartile range. *, P<0.05. a, the P value was obtained using the t-test. b, data were compared using the Mann-Whitney U test. H2 represents the height of plaque at the most stenotic site; H1 denotes the midpoint from beginning of the plaques to H2; H3 indicates the midpoint from H2 to the ending of the plaques; R12, H1/H2; R32, H3/H2. MNL, most narrowed lumen; Reference, reference site; NWI, normalized wall index; RI, remodeling index.
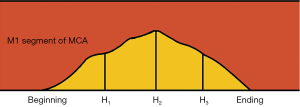
Multivariate logistic regression analyses of plaque characteristics
The multivariate logistic regression analysis showed that the three models demonstrated excellent predictive performance with area under the curve (AUC) values of 0.878 [95% confidence interval (CI): 0.807–0.949, P<0.001], 0.925 (95% CI: 0.874–0.976, P<0.001), and 0.961 (95% CI: 0.952–0.994, P<0.001), respectively. Model 3 (RI + PA + enhancement + H1 + H2 + H3 + R12 + R32), which was a combination of Models 1 and 2, showed the best predictive efficacy (Table 3 and Figure 5).
Table 3
Model | 95% CI | P value | AUC value |
---|---|---|---|
Model 1 (RI + PA + EP) | 0.807–0.949 | <0.001* | 0.878 |
Model 2 (H1 + H2 + H3 + R12 + R32) | 0.874–0.976 | <0.001* | 0.925 |
Model 3 (Model 1 + Model 2) | 0.952–0.994 | <0.001* | 0.961 |
*, P<0.05. H2 represents the height of plaque at the most stenotic site; H1 denotes the midpoint from beginning of the plaques to H2; H3 indicates the midpoint from H2 to the ending of the plaques; R12, H1/H2; R32, H3/H2. RI, remodeling index; PA, plaque area; EP, enhanced plaques; CI, confidence interval; AUC, area under the curve.
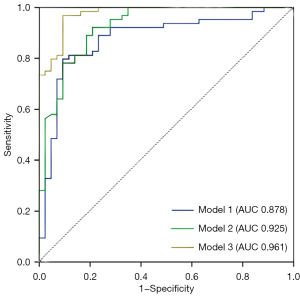
Quantitative data of the vessel wall and plaques in the AAE and LBO groups
The number of superior/posterior plaques in the LBO group was greater than that in the AAE group (16 vs. 10, P=0.018). Other quantitative data such as the VA, LA, WA, NWI, PA, degree of stenosis, RI, H1, H2, H3, R12, and R32 showed no significant differences (Table 4).
Table 4
Variables | AAE group (n=31) | LBO group (n=25) | t value | P value |
---|---|---|---|---|
Vessel areaMNL (mm2) | 13.82±4.05 | 15.28±3.86 | −1.37 | 0.176a |
Lumen areaMNL (mm2) | 2.29±0.76 | 2.44±0.84 | −0.66 | 0.509a |
Vessel areareference (mm2) | 12.61±3.59 | 14.29±3.19 | −1.84 | 0.072a |
Lumen areareference (mm2) | 6.79±2.04 | 7.48±1.53 | −1.42 | 0.162a |
Wall areaMNL (mm2) | 11.52±3.72 | 12.84±3.67 | −1.33 | 0.189a |
Wall areareference (mm2) | 5.82±2.17 | 6.81±2.40 | −1.62 | 0.111a |
NWIMNL | 0.83±0.05 | 0.83±0.06 | −0.36 | 0.718a |
NWIreference | 0.46±0.09 | 0.47±0.09 | −0.40 | 0.689a |
Plaque areaMNL (mm2) | 5.70±2.53 | 6.03±2.00 | −0.54 | 0.595a |
Degree of stenosis (%) | 66.06±7.62 | 67.92±9.77 | −0.80 | 0.428a |
RI | 1.10±0.11 | 1.07±0.08 | 1.36 | 0.180a |
H1 | 1.34±0.31 | 1.52±0.33 | −1.94 | 0.064a |
H2 | 2.45±0.44 | 2.72±0.55 | −1.99 | 0.057a |
H3 | 1.50±0.30 | 1.65±0.40 | −1.53 | 0.132a |
R12 | 0.56 (0.46–0.63) | 0.56 (0.51–0.59) | 0.779b | |
R32 | 0.62 (0.57–0.70) | 0.57 (0.54–0.69) | 0.483b | |
Superior/posterior plaques | 10 | 16 | 0.018*c | |
Enhanced plaques | 28 | 17 | 0.080d |
Data are expressed as the mean ± standard deviation, or the median and inter-quartile range. *, P<0.05. a, the P value was obtained using the t-test. b, the data were compared using the Mann-Whitney U test. c, the data were compared using the Pearson χ2 tests. d, the P value was obtained using the corrected chi-square test. H2 represents the height of plaque at the most stenotic site; H1 denotes the midpoint from beginning of the plaques to H2; H3 indicates the midpoint from H2 to the ending of the plaques; R12, H1/H2; R32, H3/H2. AAE, artery-to-artery embolism; LBO, local branch occlusion; MNL, most narrowed lumen; Reference, reference site; NWI, normalized wall index; RI, remodeling index.
Discussion
This appears to be the first study to explore the relationship between plaques and acute ischemic stroke from the perspective of plaque curvature. We found that the symptomatic group had a larger PA, RI, and NWIMNL, more enhanced plaques, and a smaller H1, H3, R12, and R32 than the asymptomatic group. The multivariate logistic regression analysis showed that the RI + PA + enhancement + H1 + H2 + H3 + R12 + R32 model had the best predictive efficacy. Meanwhile, the AAE group had a greater number of superior/posterior plaques than the LBO group.
The application of HR-MRI in ICAD is advanced and widely employed, and has been the subject of a relatively large amount of research. The VA, LA, WA, and PA were measurement parameters commonly used in early research (19,20). Similar to a previous study (16,19,20), we found that the symptomatic group had a larger PA; however, the LAMNL, VAreference, and LAreference were smaller in the symptomatic group than the asymptomatic group, and there were also some differences between other studies (14,21). To reduce the differences caused by individualization between the VA and LA, scholars have used the NWI to enhance comparability among vascular wall measurement parameters (22). We also found differences in the VAreference and LAreference between the two groups; however, the lack of a statistically significant difference in the NWIreference indicated that the differences might not be clinically significant, and might have been caused by individual variations. Similar to previous studies (3,22), we found that the NWIMNL, which reflects the plaque burden in the stenotic site, was larger in the symptomatic group than the asymptomatic group. Enhancement in plaques is believed to indicate localized active inflammation stemming from the accumulation of macrophages and neutrophils. This phenomenon has been acknowledged to be a sign of plaque vulnerability in MCA (8,9,17,23). Thus, our results were consistent with previous findings (8,9,17,23).
Vascular stenosis can lead to alterations in local hemodynamics, and the effect on hemodynamic parameters is particularly pronounced when the stenosis severity exceeds 50% (24). WSS is the principal parameter of hemodynamics, represents the tangential stress exerted by blood flow on the arterial wall, and is the primary force acting on the endothelial surface (25). In addition to the characteristics of plaques at the site of vascular stenosis, the local features of WSS are also related to the occurrence and recurrence of strokes (10,14,15). Meanwhile, the luminal curvature is a crucial factor influencing the local VSS (26), and the luminal curvature in the stenotic site depends on the plaque morphology. Thus, we conjectured that plaque curvature may reflect the luminal curvature. The surface morphology of plaques is complex and varied, and can currently only be represented using relatively simple parameters. Yang et al. (8) suggested that steepness is a geometric morphometric parameter that indicates the luminal curvature along the longitudinal axis, and used height/length to represent steepness.
In the present study, we used the height of plaques in the most stenotic site (H2), the middle point from the beginning of the plaques to H2 (H1), and the middle point from H2 to the ending of the plaques (H3) to reflect the curvature of the plaque as much as possible. To reduce the diversity in the absolute height differences between the plaques and increase comparability, we also used ratios like R12 (H1/H2) and R32 (H3/H2) to compare the symptomatic and asymptomatic groups. We found that the symptomatic group had a smaller H1, H3, R12, and R32 than the asymptomatic group, but found no statistically significant difference in the H2 between the two groups. A large R12 and R32 indicated that the plaque curvature was small; conversely, a small R12 and R32 indicated that the plaque curvature was large. The results of our study supported our hypothesis that a larger plaque curvature is more likely to cause ischemic stroke.
We also extracted more imaging information from traditional HR-MRI. The use of these three-point measurements and the plaque height ratio can indirectly demonstrate variations in WSS at the stenosis MCA, especially in hospitals lacking advanced WSS measurement capabilities. We had hoped to find some convenient and quick parameters to evaluate the risk of plaque in patients more quickly; although these parameters are simple and not comprehensive. We sought to provide parameters with as much diagnostic value as possible to clinicians and patients with limited images.
Various mechanisms contributed to ischemic stroke resulting from MCA atherosclerosis, including AAE (which is most common in patients with intracranial arterial stenosis), LBO, in situ thrombosis, and a combination of these mechanisms. AAE is thought to be caused by the instability or surface rupture of plaques, leading to the formation of microemboli that block downstream microcirculation; while LBO is thought to be caused by plaques blocking the opening of a branch artery or by disease of the branch artery (15,17). In our study, the AAE group had more enhanced plaques than the LBO group, but the difference was not statistically significant; however, this might be due to the small sample size of our study. The LBO group had more superior/posterior plaques than the AAE group, which is consistent with the findings of previous studies (3,19,20,27).
Our study had several limitations. First, it had a small sample size; thus, future studies with a larger number of subjects need to be conducted. Our research is still ongoing, and more cases will be examined in the future to further validate our research findings. Second, the exclusion of patients with MCA stenosis less than 50% limits the generalizability of our results. Third, the manual assessment of vessel wall and plaque parameters led to unavoidable measurement errors. Imaging experts in plaque analysis performed the measurements to minimize any measurement errors as much as possible. The future promotion and application of automatic measurement software will enable the resolution of manual measurement issues. Fourth, our study did not analyze plaque signal, pathological information and the length of plaque, which should be examined in future research. Fifth, we only analyzed two types of ischemic stroke resulting from MCA atherosclerosis. More types and mechanisms of infarction, and more cases need to be further investigated in the future. Sixth, the M1 segment of the MCA cannot be absolutely straight, which would inevitably have had some effect on our measurements. We hope that more precise parameters can be discovered in the future. Finally, measuring plaque height at three points and calculating their ratio may not fully capture the morphological features of the plaques. These factors should be addressed in future research.
Conclusions
The symptomatic group had a larger PA, RI, and NWIMNL, more enhanced plaques, and a smaller H1, H3, R12, and R32 than the asymptomatic group. An association was found between plaque curvature and ischemic stroke, such that larger plaque curvature was more likely to cause ischemic stroke. Ischemic stroke resulting from MCA atherosclerosis had different mechanisms. The LBO group had more superior/posterior plaques than the AAE group.
Acknowledgments
None.
Footnote
Reporting Checklist: The authors have completed the STROBE reporting checklist. Available at https://qims.amegroups.com/article/view/10.21037/qims-24-1552/rc
Funding: This study was funded by
Conflicts of Interest: All authors have completed the ICMJE uniform disclosure form (available at https://qims.amegroups.com/article/view/10.21037/qims-24-1552/coif). The authors have no conflicts of interest to declare.
Ethical Statement: The authors are accountable for all aspects of the work in ensuring that questions related to the accuracy or integrity of any part of the work are appropriately investigated and resolved. The study was conducted in accordance with the Declaration of Helsinki (as revised in 2013). The research protocol was approved by the Ethics Committee of the Nanjing Medical University (No. NMU20200320). All recruited patients provided verbal or written informed consent before they were examined.
Open Access Statement: This is an Open Access article distributed in accordance with the Creative Commons Attribution-NonCommercial-NoDerivs 4.0 International License (CC BY-NC-ND 4.0), which permits the non-commercial replication and distribution of the article with the strict proviso that no changes or edits are made and the original work is properly cited (including links to both the formal publication through the relevant DOI and the license). See: https://creativecommons.org/licenses/by-nc-nd/4.0/.
References
- Wang Y, Zhao X, Liu L, Soo YO, Pu Y, Pan Y, Wang Y, Zou X, Leung TW, Cai Y, Bai Q, Wu Y, Wang C, Pan X, Luo B, Wong KSCICAS Study Group. Prevalence and outcomes of symptomatic intracranial large artery stenoses and occlusions in China: the Chinese Intracranial Atherosclerosis (CICAS) Study. Stroke 2014;45:663-9. [Crossref] [PubMed]
- Yang R, Yuan J, Chen X, Xie X, Ye Z, Qin C. Vessel wall magnetic resonance imaging of symptomatic middle cerebral artery atherosclerosis: A systematic review and meta-analysis. Clin Imaging 2022;90:90-6. [Crossref] [PubMed]
- Wang T, Li C, Li S, Tang P, Guo Q, Fang L. High-resolution magnetic resonance imaging features of time-of-flight magnetic resonance angiography signal loss and its relevance to ischemic stroke. Quant Imaging Med Surg 2024;14:6820-9. [Crossref] [PubMed]
- Song JW, Pavlou A, Xiao J, Kasner SE, Fan Z, Messé SR. Vessel Wall Magnetic Resonance Imaging Biomarkers of Symptomatic Intracranial Atherosclerosis: A Meta-Analysis. Stroke 2021;52:193-202. [Crossref] [PubMed]
- Zhang DF, Chen YC, Chen H, Zhang WD, Sun J, Mao CN, Su W, Wang P, Yin X. A High-Resolution MRI Study of Relationship between Remodeling Patterns and Ischemic Stroke in Patients with Atherosclerotic Middle Cerebral Artery Stenosis. Front Aging Neurosci 2017;9:140. [Crossref] [PubMed]
- Zhang X, Chen L, Li S, Shi Z, Tian X, Peng W, Chen S, Zhan Q, Liu Q, Lu J. Enhancement Characteristics of Middle Cerebral Arterial Atherosclerotic Plaques Over Time and Their Correlation With Stroke Recurrence. J Magn Reson Imaging 2021;53:953-62. [Crossref] [PubMed]
- Zhang D, Wang M, Wu L, Zhao Y, Wang S, Yin X, Wu X. Assessing the characteristics and diagnostic value of plaques for patients with acute stroke using high-resolution magnetic resonance imaging. Quant Imaging Med Surg 2022;12:1529-38. [Crossref] [PubMed]
- Yang D, Liu J, Yao W, Huang K, Zhou C, Bi J, Cheng X, Ma M, Zhu W, Zhang J, Zhang L, Cai J, Teng Z, Du J, Liu X. The MRI enhancement ratio and plaque steepness may be more accurate for predicting recurrent ischemic cerebrovascular events in patients with intracranial atherosclerosis. Eur Radiol 2022;32:7004-13. [Crossref] [PubMed]
- Lv Y, Ma X, Zhao W, Ju J, Yan P, Li S, Xue Y, Sui Y, Shao S, Sun Q, Qiu C. Association of plaque characteristics with long-term stroke recurrence in patients with intracranial atherosclerotic disease: a 3D high-resolution MRI-based cohort study. Eur Radiol 2024;34:3022-31. [Crossref] [PubMed]
- Leng X, Lan L, Ip HL, Abrigo J, Scalzo F, Liu H, et al. Hemodynamics and stroke risk in intracranial atherosclerotic disease. Ann Neurol 2019;85:752-64. [Crossref] [PubMed]
- El Sayed R, Park CC, Shah Z, Nahab FB, Haussen DC, Allen JW, Oshinski JN. Assessment of Complex Flow Patterns in Patients With Carotid Webs, Patients With Carotid Atherosclerosis, and Healthy Subjects Using 4D Flow MRI. J Magn Reson Imaging 2024;59:2001-10. [Crossref] [PubMed]
- Samady H, Eshtehardi P, McDaniel MC, Suo J, Dhawan SS, Maynard C, Timmins LH, Quyyumi AA, Giddens DP. Coronary artery wall shear stress is associated with progression and transformation of atherosclerotic plaque and arterial remodeling in patients with coronary artery disease. Circulation 2011;124:779-88. [Crossref] [PubMed]
- Han N, Ma Y, Li Y, Zheng Y, Wu C, Gan T, Li M, Ma L, Zhang J. Imaging and Hemodynamic Characteristics of Vulnerable Carotid Plaques and Artificial Intelligence Applications in Plaque Classification and Segmentation. Brain Sci 2023;13:143. [Crossref] [PubMed]
- Zhang D, Wu X, Tang J, Wang P, Chen GZ, Yin X. Hemodynamics is associated with vessel wall remodeling in patients with middle cerebral artery stenosis. Eur Radiol 2021;31:5234-42. [Crossref] [PubMed]
- Woo HG, Kim HG, Lee KM, Ha SH, Jo H, Heo SH, Chang DI, Liebeskind DS, Kim BJ. Wall Shear Stress Associated with Stroke Occurrence and Mechanisms in Middle Cerebral Artery Atherosclerosis. J Stroke 2023;25:132-40. [Crossref] [PubMed]
- Xiao J, Padrick MM, Jiang T, Xia S, Wu F, Guo Y, Gonzalez NR, Li S, Schlick KH, Dumitrascu OM, Maya MM, Diniz MA, Song SS, Lyden PD, Li D, Yang Q, Fan Z. Acute ischemic stroke versus transient ischemic attack: Differential plaque morphological features in symptomatic intracranial atherosclerotic lesions. Atherosclerosis 2021;319:72-8. [Crossref] [PubMed]
- Ryu WS, Schellingerhout D, Lee H, Lee KJ, Kim CK, Kim BJ, Chung JW, Lim JS, Kim JT, Kim DH, Cha JK, Sunwoo L, Kim D, Suh SI, Bang OY, Bae HJ, Kim DE. Deep Learning-Based Automatic Classification of Ischemic Stroke Subtype Using Diffusion-Weighted Images. J Stroke 2024;26:300-11. [Crossref] [PubMed]
- Qiao Y, Zeiler SR, Mirbagheri S, Leigh R, Urrutia V, Wityk R, Wasserman BA. Intracranial plaque enhancement in patients with cerebrovascular events on high-spatial-resolution MR images. Radiology 2014;271:534-42. [Crossref] [PubMed]
- Ouyang F, Liu J, Wu Q, Chen J, Xu Z, Lv L, Wang B, Li J, Yu N, Zeng X. Relationship between the intravascular enhancement sign on three-dimensional T1-weighted turbo spin echo and intraluminal thrombus in middle cerebral artery atherosclerosis. Eur J Radiol 2024;176:111495. [Crossref] [PubMed]
- Zheng W, Zhou H, Li Y, Fan Z, Wang B, Wang Y, Zhang L, Wu X, Qin J, Wang X. High-resolution MR vessel wall imaging and cardiovascular health for evaluating the occurrence of ischemic stroke. Eur J Radiol 2024;178:111646. [Crossref] [PubMed]
- Won SY, Cha J, Choi HS, Kim YD, Nam HS, Heo JH, Lee SK. High-Resolution Intracranial Vessel Wall MRI Findings Among Different Middle Cerebral Artery Territory Infarction Types. Korean J Radiol 2022;23:333-42. [Crossref] [PubMed]
- Hou Z, Zhang Z, Yan L, You J, Wan M, Song J, Pan Y, Hui F, Miao Z, Lou X, Wang Y, Jing J, Ma N. Association of residual stenosis after balloon angioplasty with vessel wall geometries in intracranial atherosclerosis. J Neurointerv Surg 2022;14:762-6. [Crossref] [PubMed]
- Li J, Tian Y, Shi Y, Cui Y, Lian J, Liu P. Association of vulnerable plaques with white matter hyperintensities on high-resolution magnetic resonance imaging. Quant Imaging Med Surg 2024;14:3606-18. [Crossref] [PubMed]
- Corban MT, Eshtehardi P, Suo J, McDaniel MC, Timmins LH, Rassoul-Arzrumly E, Maynard C, Mekonnen G, King S 3rd, Quyyumi AA, Giddens DP, Samady H. Combination of plaque burden, wall shear stress, and plaque phenotype has incremental value for prediction of coronary atherosclerotic plaque progression and vulnerability. Atherosclerosis 2014;232:271-6. [Crossref] [PubMed]
- Kheloufi M, Vion AC, Hammoutene A, Poisson J, Lasselin J, Devue C, Pic I, Dupont N, Busse J, Stark K, Lafaurie-Janvore J, Barakat AI, Loyer X, Souyri M, Viollet B, Julia P, Tedgui A, Codogno P, Boulanger CM, Rautou PE. Endothelial autophagic flux hampers atherosclerotic lesion development. Autophagy 2018;14:173-5. [Crossref] [PubMed]
- Dahal S, Raja AY, Searle E, Colgan FE, Crighton JS, Roake J, Saba L, Gieseg S, Butler APH. Components of carotid atherosclerotic plaque in spectral photon-counting CT with histopathologic comparison. Eur Radiol 2023;33:1612-9. [Crossref] [PubMed]
- Zhang DF, Wu XY, Zhang WD, Wang M, Yin X, Chen YC. The Relationship between Patterns of Remodeling and Degree of Enhancement in Patients with Atherosclerotic Middle Cerebral Artery Stenosis: A High-Resolution MRI Study. Neurol India 2021;69:1663-9. [Crossref] [PubMed]