Multiparametric cardiovascular magnetic resonance in patients with myocarditis with consecutive follow-up and a comparison between non-COVID-19 and COVID-19-associated myocarditis
Introduction
Acute myocarditis is a common cardiovascular disease with high morbidity and mortality, accounting for 2–42% of sudden cardiac deaths in young adults and 9–16% of primary dilated cardiomyopathy in adults (1,2). Therefore, a comprehensive evaluation of cardiac remodeling, dysfunction, and myocardial injury is required for better disease management. Owing to the multimodality sequences of cardiac magnetic resonance (CMR) including T2 weighted imaging (T2WI), early gadolinium enhancement (EGE), late gadolinium enhancement (LGE), T1 mapping, T2 mapping, and other sequences, detecting myocardial edema, hyperemia, necrosis, and myocardial fibrosis, which play a unique role in diagnosis and prognosis, is possible (3,4); CMR is also listed in the guidelines as an essential examination for the clinical diagnosis of myocarditis (5,6). In fulminant and chronic myocarditis, the assessment of myocardial injury and dysfunction (7,8) is important, and studies have reported myocardial injury and impaired function using CMR in patients with myocarditis (9-11). However, research on the consecutive follow-up of patients with myocarditis to elucidate the recovery or progression pattern of cardiac function and myocardial characteristics is limited.
Coronavirus disease 2019 (COVID-19)-associated myocarditis (CAM) emerged in the past 4 years during the COVID-19 pandemic. Several studies have reported the diagnostic value of CMR in CAM (12,13), and the guidelines has been further clarified (14). Some studies have shown that residual myocardial damage with reduced cardiac function has been noted following recovery from CAM (15). Whether the pattern of myocardial damage and dysfunction development in CAM is consistent with that of non-COVID-19-associated myocarditis (NCAM) during follow-up remains unclear.
This study aimed to track myocardial characteristics and cardiac function in myocarditis with consecutive follow-up using CMR and compare between NCAM and CAM, and to investigate the clinical value of CMR in assessing myocardial injury and cardiac dysfunction during follow-up in patients with myocarditis. We present this article in accordance with the STROBE reporting checklist (available at https://qims.amegroups.com/article/view/10.21037/qims-24-1422/rc).
Methods
Patient population
The study was conducted in accordance with the Declaration of Helsinki (as revised in 2013). The study was approved by the institutional ethics committee of Affiliated Jinhua Hospital, Zhejiang University School of Medicine (No. 2019-171) and informed consent was taken from all the patients. Data of patients with NCAM during follow-up were collected from March 2013 to December 2022, of which short-term follow-up cases were collected from cases with clinically diagnosed acute myocarditis in Affiliated Jinhua Hospital, Zhejiang University School of Medicine between July 2020 and December 2022. All cases underwent follow-up CMR. The proposed diagnostic CMR criteria for myocarditis were based on the 2009 and 2018 Lake Louise Criteria (5,6), including at least two of the following criteria: (I) focal or diffuse high signal intensity on T2WI sequence; (II) presence of EGE; and (III) presence of LGE.
Patients with CAM during follow-up were enrolled between January 2023 and October 2023, and the diagnostic criteria for CAM were based on the 2022 American College of Cardiology Expert Consensus on CAM (14), which included patients with cardiac symptoms, such as chest pain, palpitation; elevated troponin levels; COVID-19 infection (tested positive by reverse transcription polymerase chain reaction or antigen); abnormal electrocardiogram or echocardiogram results without evidence of myocardial ischemia, such as acute coronary syndrome; and could not be explained by severe infections and acute respiratory distress syndrome. The following were the exclusion criteria in this study: claustrophobia, arrhythmia, and uncooperative breathing for which image quality was poor.
Overall, this study included 75 patients with NCAM during follow-up. Of the patients, 12 patients were followed up using CMR within 3 months (short-term follow-up), 12 were followed up with CMR over 6 months (medium- and long-term follow-up), respectively (11 patients underwent two consecutive follow-up CMR). Myocarditis patients, control cohort recruitment and exclusion flowchart were shown in Figure S1. The follow-up CMR time was 8–1,176 weeks after myocarditis, with a median examination time of 168 weeks. The median age of the patients was 40.25±15.45 years, and 31 patients were female. This study included 25 patients with CAM during follow-up. The follow-up CMR time was 2–9 weeks after myocarditis, and the median examination time was 4 weeks. The mean age was 34.48±16.96 years, and 10 patients were female. This study recruited 40 patients with normal CMR, echocardiography, electrocardiography, myocardial enzyme, cardiac Troponin I and 35 normal volunteers as the control group. Volunteers underwent non-contrast CMR. The mean age was 36.37±16.13 years, and 44 patients were female. In the control group, 25 patients were randomly selected as another control group to be compared with the CAM and NCAM short-term follow-up groups, respectively. The ethics committee of our hospital approved this study, and informed consent was obtained from all patients and volunteers before CMR.
Image acquisition
CMR images were acquired using a 3.0T magnetic resonance imaging (MRI) scanner (Achieva, Philips, The Netherlands) and a 3.0T MRI scanner (Architect, GE, USA) with a 16-channel phased-array torso coil and a 32-channel phased-array cardiac coil. The CMR protocols comprised two-chamber, three-chamber, four-chamber, and short-axis view cines, T2WI/triple inversion recovery (IR), T1 mapping, T2 mapping, EGE, and LGE sequences.
For T2WI/triple IR, T1, and T2 mapping sequences, basal, middle, and apical view images were acquired. T1 mapping was acquired using a motion-corrected Modified Look-Locker inversion-recovery sequence scheme, with protocol 5(3)3 and 4(1)3(1)2 for native T1 mapping. T2 mapping was obtained using Multi-Echo Grase Black-Blood Acquisition on Philips scanner. T2 mapping was generated using double IR fast spin echo sequence on GE scanner.
The following scanning parameters were the same for two scanners: slice thickness, 8 mm; gap, 2 mm; field of view, [300–360] mm × [300–360] mm; matrix size, [160–244] mm × [160–259] mm; voxel size, (2.0–2.5) mm × (2.0–2.5) mm × 8.0 mm; inversion time for EGE, 200–250 ms; inversion time for LGE, 370–420 ms.
The following scanning parameters were for Philips scanner. Cine sequence: bandwidth, 1,706 Hz/pixel; repetition time (TR)/echo time (TE), 3.0/1.5 ms; flip angle (FA), 45°. T2WI sequence: bandwidth, 503 Hz/pixel; TR/TE, 1,791.04/75.00 ms; FA, 90°. EGE and LGE sequences: bandwidth, 238 Hz/pixel; TR/TE, 6.13/3.0 ms; FA, 25°. T1 mapping sequence: bandwidth, 1,085 Hz/pixel; TR/TE, 2.14/1.00 ms; FA, 20°. T2 mapping sequence: bandwidth, 2,054 Hz/pixel; TR/TE, 779.22/(8.35–75.11) ms; FA, 90°.
The following scanning parameters were for GE scanner. Cine sequence: bandwidth, 390.63 Hz/pixel; TR/TE, 3.22/1.22 ms; FA, 45°. Triple IR sequence: bandwidth, 325.51 Hz/pixel; TR/TE, 2,033.90/65.34 ms; FA, 107°. EGE and LGE sequences: bandwidth, 139.49 Hz/pixel; TR/TE, 4.39/1.99 ms; FA, 20°. T1 mapping sequence: bandwidth, 781.25 Hz/pixel; TR/TE, 3/1.29 ms; FA, 35°. T2 mapping sequence: bandwidth, 651.02 Hz/pixel; TR/TE, 1,000/(10.95–93.09) ms; FA, 90°.
The contrast agent used was gadoteric acid meglumine (Hengrui Pharmaceuticals, China), with a dosage of 0.2 mmol/kg. LGE and post-contrast T1 mapping were acquired 10 min following intravenous contrast agent injection.
CMR analysis
CMR review and postprocessing were performed using a dedicated CMR software, CVI42 (version 6.0.2, Circle Cardiovascular Imaging, Canada). For myocardial edema, T2WI or Triple IR sequence was used for measuring the signal intensity of focal or whole myocardium and skeletal muscle. Myocardial edema was present when the signal intensity of the myocardium/skeletal muscle was >2. Myocardial hyperemia and necrosis were analyzed using EGE and LGE sequences by visual assessment, and quantitative assessment was automated generated together with manual adjustment. Auto-segmentation and manual adjustment were also applied in the analysis of left ventricular end-diastolic volume (LVEDV), right ventricular end-diastolic volume (RVEDV), left ventricular end-systolic volume (LVESV), right ventricular end-systolic volume (RVESV), left ventricular stroke volume (LVSV), right ventricular stroke volume (RVSV), left ventricular ejection fraction (LVEF), and right ventricular ejection fraction (RVEF). T1 mapping analysis was performed by using the T1 module, which generated the average native T1 value, post-contrast T1 value, and extracellular volume (ECV) parameters. T2 mapping analysis was performed by using the T2 module, which obtained the average T2 value. LV myocardial strain analysis was performed by the tissue tracking module using two-chamber, three-chamber, four-chamber, and short-axis view cines. The apex, left ventricular base, and boundary of the endocardium and epicardium at end diastole were defined automatically and subsequently acquired the global left ventricular myocardial strains, including global radial strain (GRS), global circumferential strain (GCS), and global longitudinal strain (GLS).
Statistical analysis
Quantitative data were described as mean ± standard deviation, and qualitative data were expressed as percentages. The normality of data distribution was analyzed using the Kolmogorov-Smirnov test. Independent student t-test was used for data with normal distribution, and nonparametric Mann-Whitney U-test for was used for non-normal distribution parameters. Qualitative data were analyzed using Chi-squared test or Fisher’s exact test. Comparisons between baseline, short-term follow-up, medium-, and long-term follow-up of the NCAM group were analyzed using repeated measures analysis of variance, and when “sphericity” was not satisfied, Greenhouse-Geisser or Huynh-Feldt corrections were used for between-group comparisons. Pearson or Spearman correlation analysis was used to analyze correlations between measures. The diagnostic efficacy of different parameters was tested using the area under the cure (AUC) via receiver operating characteristic analysis. When multiple parameters were combined to test the diagnostic efficacy, logistic regression was used for calculating the predicted probability of each parameter, which was sent to calculate the diagnostic efficacy using analysis. Statistical analyses and graphs were performed using Statistical Package for the Social Sciences (version 20.0; IBM Corp., Armonk, NY, USA) and MedCalc (version 18.2.1; MedCalc Software Ltd., Ostend, Belgium). P<0.05 was considered statistically significant.
Results
In this study, comparing the clinical baseline characteristics of patients with myocarditis revealed that patients with CAM during follow-up had a higher proportion with chest pain, elevated troponin levels, combined pneumonia, and a higher New York Heart Association classification. Of the 75 patients with NCAM, 40 underwent T1 mapping and T2 mapping sequences. Combining 2009 and 2018 CMR Lake Louise Criteria showed that 21 cases still met the diagnostic criteria for myocarditis. Of the 25 patients with CAM, 14 met the diagnosis criteria of myocarditis. Between these two groups, the CAM follow-up group had a higher proportion of patients with pericardial effusion, higher T2 values, and a higher proportion of patients who met CMR Lake Louise Criteria for myocarditis (Table 1 and Figure 1).
Table 1
Variable | NCAM (n=75) | CAM (n=25) | P value |
---|---|---|---|
Age (years) | 40.25±15.45 | 36.37±16.13 | 0.084 |
Gender | 0.197 | ||
Female | 31 [41.33] | 10 [40] | |
Male | 44 [58.67] | 15 [60] | |
Hypertension | 13 [17.33] | 3 [12] | 0.753 |
Diabetes mellitus | 3 [4] | 0 | 1.000 |
Coronary heart disease | 2 [2.67] | 1 [4] | 1.000 |
Chest pain | 29 [38.67] | 18 [72] | 0.004 |
Palpitation | 18 [24] | 3 [12] | 0.202 |
NYHA class | 1.12±0.46 | 1.60±0.87 | <0.001 |
Abnormal ECG | 39 [52] | 12 [48] | 0.730 |
Abnormal ECHO | 7 [9.33] | 3 [12] | 1.000 |
Elevated TnI | 9 [12] | 15 [60] | <0.001 |
Elevated CK/CKMB | 11 [14.67] | 6 [24] | 0.282 |
Elevated BNP | 8 [10.67] | 5 [20] | 0.229 |
Pneumonia | 5 [6.67] | 8 [32] | 0.001 |
CMR | |||
High T2 SI | 18 [24] | 11 [44] | 0.056 |
EGE | 20 [26.67] | 8 [32] | 0.607 |
LGE | 40 [53.33] | 13 [52] | 0.908 |
Pericardial effusion | 6 [8] | 12 [48] | <0.001 |
Native T1 (ms) | 1,302.30±97.65 | 1,273.56±90.32 | 0.383 |
Post contrast T1 (ms) | 572.08±87.05 | 590.00±67.30 | 0.349 |
ECV (%) | 30.98±4.82 | 31.72±4.58 | 0.607 |
T2 (ms) | 50.23±4.74 | 52.56±3.64 | 0.040 |
2009/2018 Lake Louise Criteria for myocarditis | 21 [28] | 14 [56] | 0.011 |
Data are presented as mean ± standard deviation or n [%]. For NCAM patients, 40 cases underwent T1 and T2 mapping sequences. CMR, cardiac magnetic resonance; CAM, COVID-19 associated myocarditis; NCAM, non-COVID-19 associated myocarditis; COVID-19, coronavirus disease 2019; NYHA, New York Heart Association; ECG, electrocardiography; ECHO, echocardiography; TnI, troponin I; CK, creatine kinase; CKMB, creatine kinase isoenzyme; BNP, brain natriuretic peptide; SI, signal intensity; EGE, early gadolinium enhancement; LGE, late gadolinium enhancement; ECV, extracellular volume.
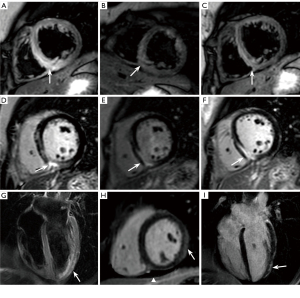
Comparing the CMR parameters between the NCAM follow-up and control groups (Table 2) showed increased LVEDV, LVESV, RVEDV, RVESV, and ECV in the NCAM follow-up group and decreased LVEF, RVEF, GRS, GCS, and GLS (Figure 2) post-contrast T1. Correlation analysis revealed that none of the correlations between CMR metrics were strong, with the best correlation coefficient between LVESV and GRS at −0.635, and correlation coefficients between the rest of the parameters were <0.6. The results of discriminative efficacy analysis based on CMR between the NCAM follow-up and control groups (Table 3) showed that among the traditional parameters of CMR volume and EF values, LVESV had the best AUC of 0.727, with 77.3% sensitivity and 61.3% specificity. Furthermore, among the new technical parameters of CMR including myocardial strain and mapping, GLS yielded the best AUC of 0.836, with 78.7% sensitivity and 84.0% specificity; in the multiparameter joint of RVEF, GRS, and GLS, the discriminative efficacy showed the best AUC of 0.847, with 80.0% sensitivity and 85.3% specificity (Figure 3).
Table 2
Variable | Control (n=75) | NCAM (n=75) | P value |
---|---|---|---|
LVEDV (mL) | 112.15±24.87 | 135.88±37.20 | <0.001 |
LVEDVi (mL/m2) | 68.94±12.94 | 78.91±19.06 | <0.001 |
LVESV (mL) | 42.80±12.11 | 61.65±38.33 | <0.001 |
LVESVi (mL/m2) | 26.02±6.85 | 35.68±20.83 | <0.001 |
LVSV (mL) | 70.70±15.67 | 74.23±16.86 | 0.186 |
LVSVi (mL/m2) | 42.94±7.94 | 43.23±8.88 | 0.831 |
LVEF (%) | 62.57±5.38 | 56.67±11.15 | 0.001 |
RVEDV (mL) | 117.19±27.88 | 128.86±27.74 | 0.011 |
RVEDVi (mL/m2) | 71.08±14.89 | 74.85±13.09 | 0.011 |
RVESV (mL) | 49.14±14.22 | 60.32±21.26 | <0.001 |
RVESVi (mL/m2) | 29.90±7.97 | 34.99±11.33 | 0.001 |
RVSV (mL) | 67.37±17.02 | 68.54±17.17 | 0.676 |
RVSVi (mL/m2) | 40.95±9.04 | 39.86±8.66 | 0.452 |
RVEF (%) | 58.34±4.88 | 53.72±8.89 | <0.001 |
LGE (g) | – | 0.52 (0–6.41) | – |
LGE_extent (%) | – | 0.60 (0–8.30) | – |
GRS (%) | 37.20±6.85 | 35.09±18.96 | 0.009 |
GCS (%) | (−) 19.78±2.24 | (−) 16.88±9.32 | 0.001 |
GLS (%) | (−) 15.73±2.15 | (−) 11.57±4.73 | <0.001 |
Native T1 (ms) | 1,274.70±62.74 | 1,302.30±97.65 | 0.137 |
Post contrast T1 (ms) | 621.80±122.56 | 572.08±87.05 | 0.040 |
ECV (%) | 28.35±2.92 | 30.98±4.82 | 0.029 |
T2 (ms) | 49.15±3.29 | 50.23±4.75 | 0.243 |
Data are presented as mean ± standard deviation or median (interquartile range). Forty cases of NCAM patients and 40 cases of control group underwent T1 and T2 mapping sequences. CMR, cardiac magnetic resonance; NCAM, non-COVID-19 associated myocarditis; COVID-19, coronavirus disease 2019; LVEDV, left ventricular end-diastolic volume; LVEDVi, left ventricular end-diastolic volume index; RVEDV, right ventricular end-diastolic volume; RVEDVi, right ventricular end-diastolic volume index; LVESV, left ventricular end-systolic volume; RVESV, right ventricular end-systolic volume; LVESVi, left ventricular end-systolic volume index; RVESVi, right ventricular end-systolic volume index; LVSV, left ventricular stroke volume; RVSV, right ventricular stroke volume; LVSVi, left ventricular stroke volume index; RVSVi, right ventricular stroke volume index; LVEF, left ventricular ejection fraction; RVEF, right ventricular ejection fraction; LGE, late gadolinium enhancement; GRS, global radial strain; GCS, global circumferential strain; GLS, global longitudinal strain; ECV, extracellular volume.
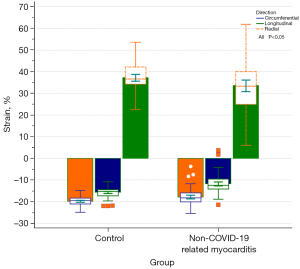
Table 3
Variable | AUC | P value | Criterion | Sensitivity (%) | Specificity (%) |
---|---|---|---|---|---|
LVEDV (mL) | 0.708 | 0.001 | 105.00 | 82.7 | 50.7 |
LVEDVi (mL/m2) | 0.671 | <0.001 | 70.00 | 69.3 | 60 |
LVESV (mL) | 0.727 | <0.001 | 42.00 | 77.3 | 61.3 |
LVESVi (mL/m2) | 0.704 | <0.001 | 24.30 | 85.3 | 49.3 |
LVEF (%) | 0.335 | 0.001 | 55.68 | 36.0 | 94.7 |
RVEDV (mL) | 0.620 | 0.011 | 98.00 | 92.0 | 30.7 |
RVEDVi (mL/m2) | 0.594 | 0.047 | 66.00 | 78.7 | 41.3 |
RVESV (mL) | 0.682 | <0.001 | 48.19 | 76.0 | 58.7 |
RVESVi (mL/m2) | 0.658 | 0.045 | 27.81 | 81.3 | 50.7 |
RVEF (%) | 0.323 | 0.044 | 52.96 | 45.3 | 90.7 |
GRS (%) | 0.376 | 0.047 | 33.53 | 52.0 | 78.7 |
GCS (%) | 0.664 | 0.045 | −17.43 | 42.7 | 92.0 |
GLS (%) | 0.836 | 0.034 | −14.3 | 78.7 | 84.0 |
RVEF + GRS + GLS | 0.847 | <0.001 | – | 80.0 | 85.3 |
Native T1 (ms) | 0.581 | 0.211 | 1,256.00 | 75.0 | 47.5 |
Post contrast T1 (ms) | 0.383 | 0.073 | 591 | 65.0 | 62.5 |
ECV (%) | 0.685 | 0.004 | 28.00 | 67.5 | 65.0 |
T2 (ms) | 0.550 | 0.439 | 51 | 30.0 | 87.5 |
The total patient number is 150 for control group and NCAM patients, in which 80 patients underwent T1 and T2 mapping sequences. CMR, cardiac magnetic resonance; NCAM, non-COVID-19 associated myocarditis; COVID-19, coronavirus disease 2019; LVEDV, left ventricular end-diastolic volume; LVEDVi, left ventricular end-diastolic volume index; RVEDV, right ventricular end-diastolic volume; RVEDVi, right ventricular end-diastolic volume index; LVESV, left ventricular end-systolic volume; RVESV, right ventricular end-systolic volume; LVESVi, left ventricular end-systolic volume index; RVESVi, right ventricular end-systolic volume index; LVEF, left ventricular ejection fraction; RVEF, right ventricular ejection fraction; GRS, global radial strain; GCS, global circumferential strain; GLS, global longitudinal strain; ECV, extracellular volume; AUC, area under the curve.
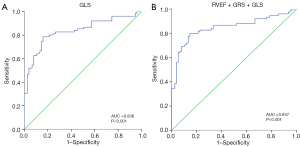
For patients with NCAM at different follow-up times including short-term follow-up and, medium-, and long-term follow-up (Table 4), the differences in CMR parameters between groups were not statistically significant. Subgroup comparisons indicated that the short-term follow-up showed decreased native T1 and T2 values compared with the medium- and long-term follow-up (Figure 4).
Table 4
Variable | Baseline (A) (n=11) | First follow up (short-term follow-up; B) (n=11) | Second follow-up (medium- and long-term follow-up; C) (n=11) | P value | |||
---|---|---|---|---|---|---|---|
All | A vs. B | A vs. C | B vs. C | ||||
LVEDV (mL) | 133.52±11.67 | 125.58±9.96 | 128.25±11.17 | 0.296 | 0.164 | 0.304 | 0.602 |
LVEDVi (mL/m2) | 76.76±2.70 | 73.14±3.81 | 73.99±3.42 | 0.434 | 0.214 | 0.358 | 0.785 |
LVESV (mL) | 60.09±5.72 | 56.46±4.47 | 57.39±6.19 | 0.469 | 0.280 | 0.354 | 0.768 |
LVESVi (mL/m2) | 34.58±1.80 | 32.97±1.89 | 33.20±2.67 | 0.599 | 0.378 | 0.894 | 0.894 |
LVSV (mL) | 73.43±6.81 | 69.12±6.79 | 70.85±5.81 | 0.585 | 0.497 | 0.313 | 0.752 |
LVSVi (mL/m2) | 42.18±1.90 | 40.17±2.89 | 40.79±1.23 | 0.733 | 0.533 | 0.356 | 0.838 |
LVEF (%) | 54.97±1.57 | 54.78±2.07 | 55.73±1.82 | 0.779 | 0.943 | 0.444 | 0.679 |
RVEDV (mL) | 133.75±13.55 | 130.27±10.81 | 132.50±11.79 | 0.762 | 0.530 | 0.828 | 0.449 |
RVEDVi (mL/m2) | 76.99±4.58 | 75.63±3.59 | 76.68±3.53 | 0.883 | 0.680 | 0.930 | 0.522 |
RVESV (mL) | 62.83±7.64 | 66.42±5.70 | 63.93±5.81 | 0.502 | 0.436 | 0.794 | 0.086 |
RVESVi (mL/m2) | 36.21±2.72 | 38.46±1.69 | 37.01±1.82 | 0.412 | 0.345 | 0.711 | 0.065 |
RVSV (mL) | 70.92±7.96 | 63.85±5.92 | 68.57±6.36 | 0.354 | 0.227 | 0.714 | 0.117 |
RVSVi (mL/m2) | 40.78±3.25 | 37.16±2.57 | 39.67±2.05 | 0.439 | 0.289 | 0.748 | 0.138 |
RVEF (%) | 52.87±2.27 | 48.82±1.75 | 51.71±0.96 | 0.256 | 0.211 | 0.664 | 0.070 |
LGE (g) | 7.69±3.12 | 7.65±3.00 | 10.33±4.70 | 0.582 | 0.988 | 0.472 | 0.311 |
LGE_extent (%) | 9.78±3.66 | 10.48±4.33 | 12.80±6.02 | 0.648 | 0.828 | 0.492 | 0.374 |
GRS (%) | 32.69±3.35 | 28.06±1.63 | 29.10±1.86 | 0.255 | 0.173 | 0.294 | 0.608 |
GCS (%) | (−) 16.90±0.77 | (−) 17.03±0.89 | (−) 17.45±0.54 | 0.778 | 0.881 | 0.384 | 0.662 |
GLS (%) | (−) 12.09±1.16 | (−) 12.36±0.91 | (−) 12.75±1.01 | 0.688 | 0.770 | 0.377 | 0.574 |
Native T1 (ms) | 1,264.10±12.66 | 1,256.50±18.63 | 1,248.30±17.87 | 0.348 | 0.584 | 0.284 | 0.007 |
Post contrast T1 (ms) | 610.60±19.18 | 641.80±16.86 | 647.70±29.91 | 0.091 | 0.050 | 0.109 | 0.715 |
ECV (%) | 29.70±1.27 | 29.20±1.22 | 29.90±1.68 | 0.605 | 0.273 | 0.853 | 0.466 |
T2 (ms) | 46.60±5.96 | 49.00±1.34 | 47.50±0.97 | 0.710 | 0.669 | 0.871 | 0.015 |
Data are presented as mean ± standard deviation. CMR, cardiac magnetic resonance; NCAM, COVID-19 associated myocarditis; LVEDV, left ventricular end-diastolic volume; LVEDVi, left ventricular end-diastolic volume index; RVEDV, right ventricular end-diastolic volume; RVEDVi, right ventricular end-diastolic volume index; LVESV, left ventricular end-systolic volume; RVESV, right ventricular end-systolic volume; LVESVi, left ventricular end-systolic volume index; RVESVi, right ventricular end-systolic volume index; LVSV, left ventricular stroke volume; RVSV, right ventricular stroke volume; LVSVi, left ventricular stroke volume index; RVSVi, right ventricular stroke volume index; LVEF, left ventricular ejection fraction; RVEF, right ventricular ejection fraction; LGE, late gadolinium enhancement; GRS, global radial strain; GCS, global circumferential strain; GLS, global longitudinal strain; ECV, extracellular volume.
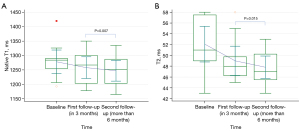
Comparison between the CAM short-term follow-up, NCAM short-term follow-up, and control groups (Table 5) showed that the CAM short-term follow-up group had increased LVEDV, LVESV, RVEDV, RVESV, ECV, and T2 and decreased RVEF, GCS, and GLS compared with the control group, and increased T2 in the CAM short-term follow-up group compared with that in the NCAM short-term follow-up group. LVESV and RVESV were increased; LVEF, RVEF, GRS, GCS, and GLS were decreased in the NCAM short-term follow-up group compared with those in the control group.
Table 5
Variable | Control group (A) (n=25) | Short-term follow-up CAM group (B) (n=25) | Short-term follow-up NCAM group (C) (n=12) | P value | ||
---|---|---|---|---|---|---|
A vs. B | A vs. C | B vs. C | ||||
LVEDV (mL) | 115.55±30.50 | 142.40±45.62 | 126.01±28.60 | 0.018 | 0.326 | 0.263 |
LVEDVi (mL/m2) | 67.42±11.84 | 81.24±27.19 | 73.42±10.94 | 0.026 | 0.148 | 0.222 |
LVESV (mL) | 44.13±14.40 | 65.36±45.77 | 55.59±13.20 | 0.035 | 0.026 | 0.476 |
LVESVi (mL/m2) | 25.76±6.59 | 37.80±29.30 | 32.51±6.01 | 0.111 | 0.005 | 0.399 |
LVSV (mL) | 71.46±18.81 | 77.00±20.79 | 70.42±20.29 | 0.328 | 0.879 | 0.370 |
LVSVi (mL/m2) | 41.66±6.93 | 43.52±9.85 | 40.91±8.61 | 0.444 | 0.778 | 0.438 |
LVEF (%) | 62.39±5.84 | 57.28±13.73 | 55.61±6.84 | 0.317 | 0.008 | 0.127 |
RVEDV (mL) | 118.79±33.80 | 141.68±41.36 | 130.99±31.44 | 0.037 | 0.301 | 0.435 |
RVEDVi (mL/m2) | 69.10±13.81 | 79.72±17.89 | 76.08±10.36 | 0.023 | 0.130 | 0.519 |
RVESV (mL) | 49.07±17.23 | 70.44±25.94 | 66.01±17.21 | 0.002 | 0.011 | 0.604 |
RVESVi (mL/m2) | 28.68±7.83 | 39.68±13.00 | 38.38±6.43 | 0.002 | 0.002 | 0.721 |
RVSV (mL) | 69.64±18.12 | 71.16±22.21 | 64.98±20.29 | 0.793 | 0.485 | 0.421 |
RVSVi (mL/m2) | 40.62±6.97 | 40.00±10.03 | 37.70±9.08 | 0.801 | 0.287 | 0.505 |
RVEF (%) | 59.16±4.46 | 51.28±10.73 | 49.21±7.87 | 0.006 | <0.001 | 0.436 |
LGE (g) | – | 0.68±1.43; 1.40 (0–15.84) | 6.56±8.95; 0.14 (0–0.72) | – | – | 0.133 |
LGE_extent (%) | – | 0.59±1.13; 1.8 (0–17.20) | 9.00±12.87; 0.25 (0–0.54) | – | – | 0.116 |
GRS (%) | 35.95±6.77 | 33.14±11.57 | 30.43±8.75 | 0.299 | 0.042 | 0.479 |
GCS (%) | (–) 20.00±2.33 | (–) 16.99±5.04 | (–) 16.98±2.76 | 0.011 | 0.001 | 0.992 |
GLS (%) | (–) 16.09±2.78 | (–) 12.95±4.85 | (–) 12.43±2.60 | 0.006 | <0.001 | 0.671 |
Native T1 (ms) | 1,272.48±57.25 | 1,273.56±90.33 | 1,251.00±58.73 | 0.960 | 0.297 | 0.437 |
Post contrast T1 (ms) | 620.16±103.69 | 590.00±67.30 | 627.67±58.56 | 0.228 | 0.817 | 0.106 |
ECV (%) | 27.92±2.71 | 31.72±4.58 | 29.50±3.78 | 0.001 | 0.310 | 0.142 |
T2 (ms) | 48.36±2.91 | 52.56±3.64 | 49.08±3.84 | <0.001 | 0.856 | 0.008 |
Data are presented as mean ± standard deviation or median (range). CMR, cardiac magnetic resonance; NCAM, COVID-19 associated myocarditis; LVEDV, left ventricular end-diastolic volume; LVEDVi, left ventricular end-diastolic volume index; RVEDV, right ventricular end-diastolic volume; RVEDVi, right ventricular end-diastolic volume index; LVESV, left ventricular end-systolic volume; RVESV, right ventricular end-systolic volume; LVESVi, left ventricular end-systolic volume index; RVESVi, right ventricular end-systolic volume index; LVSV, left ventricular stroke volume; RVSV, right ventricular stroke volume; LVSVi, left ventricular stroke volume index; RVSVi, right ventricular stroke volume index; LVEF, left ventricular ejection fraction; RVEF, right ventricular ejection fraction; LGE, late gadolinium enhancement; GRS, global radial strain; GCS, global circumferential strain; GLS, global longitudinal strain; ECV, extracellular volume.
Correlation analysis between the CMR indicators of the NCAM short-term follow-up group showed that GCS was moderately correlated with RVEF and ECV, with coefficients of −0.696 and 0.743, respectively. Correlation analysis between the CMR indicators of the CAM short-term follow-up group showed that LVEF correlated well with GRS, GCS, and GLS, with coefficients of −0.852, −0.860, and −0.698, respectively. Moreover, the correlation between RVEF and GRS, GCS, and GLS was good, with coefficients of −0.839, −0.811, and −0.747, respectively (Table S1). The receiver operating characteristic analysis of discriminative efficacy between the CAM short-term follow-up and control groups showed that left ventricular end-systolic volume index (LVESVi) had the best AUC of 0.758, with 80% sensitivity and 64% specificity. The analysis showed that GLS had the best AUC of 0.910, with 91.67% sensitivity and 92.00% specificity (Table S2). For the diagnostic efficacy of the CAM and NCAM short-term follow-up groups, the T2 value was the sole parameter that reached statistical significance, with an AUC of 0.772, 76% sensitivity, and 83% specificity (Figure 5). The discriminative power analysis of the joint multiparameter between the CAM short-term follow-up and control groups was performed, and the logistic regression analysis showed that the predictive probability of all parameters was not statistically significant and that they could not be used for constructing the joint multiparameter to further improve the discriminative power.
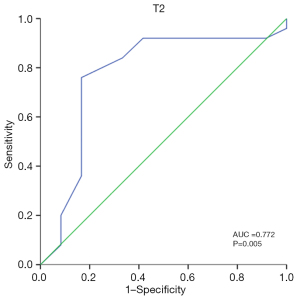
Discussion
This study revealed several significant findings as follows: (I)the NCAM short-term follow-up group exhibited residual myocardial injury, ventricular remodeling, and dysfunction; (II) comparison between the medium- and long-term follow-up and short-term follow-up groups in patients with NCAM showed recovery of myocardial edema; however, the improvement in ventricular remodeling and dysfunction was not significant; (III) at short-term follow-up, patients with CAM during following showed more severe myocardial edema than those with NCAM.
Patients with CAM during follow-up had a higher New York Heart Association classification than those with NCAM during follow-up, which was likely correlated with a higher proportion of comorbid pneumonia in the CAM follow-up group. Regarding CMR characteristics, the CAM follow-up group had a higher proportion of pericardial effusion and Lake Louise Criteria diagnosis, as well as higher T2 values. The patients in the CAM short-term follow-up group were followed for a shorter period, and most of them underwent CMR because of symptoms of chest pain and palpitations. Clinical attention to the late recovery and management of myocarditis is relatively low, and the comprehensive evaluation of disease recovery is frequently performed by echocardiography, electrocardiography, troponin level determination, and brain natriuretic peptide test. For myocarditis, CMR has unique advantages particularly in its ability to demonstrate myocardial edema, hyperemia, or necrosis (13,16). Additionally, it plays a significant role not only during acute myocarditis but also during subacute onset and subsequent follow-up (13,17). At CMR follow-up, myocardial edema, hyperemia, and fibrosis can still be observed in a subset of patients, and the CMR performance of some patients can still meet the 2018 CMR Lake Louise Criteria, suggesting that myocardial injury has not fully recovered.
One study (18) showed that in patients with COVID-19 vaccine-associated myocarditis, myocardial edema was noted in 25% of patients at the short-term follow-up (up to 25 days) after disease onset, with residual myocardial fibrosis confirmed by medium- and long-term follow-up CMR. Another study (19) noted a reduction in the extent of delayed myocardial enhancement and improvement in cardiac function in patients with COVID-19 vaccine-associated myocarditis at the 6-month medium-term follow-up. Patients with CAM had more severe cardiac function impairment and worse prognosis than those with COVID-19 vaccine-associated myocarditis (20). However, the difference in prognosis between patients with CAM and NCAM did not show statistical significance (21). In our study, three patients with NCAM during follow-up and one patient with CAM during follow-up presented with fulminant manifestations at disease onset. Fulminant myocarditis is less frequent, more severe, and has a worse prognosis (22). During follow-up of the patients with fulminant myocarditis, only one patient with NCAM developed enlarged heart and decreased cardiac function, possibly predicting a poorer prognosis; the CMR characteristics and cardiac function of the remaining three patients were normal, suggesting a good prognosis. This finding may also be related to the small number of follow-up cases of fulminant myocarditis included in our study.
In the NCAM follow-up group, left and right ventricular volumes were increased and ejection fraction were decreased compared with the control group; however, the difference was slight, and most indices including ejection fraction remained within the normal range, suggesting that subclinical left ventricular remodeling and dysfunction can be present in this group of patients. The presence of subclinical left ventricular dysfunction was further confirmed by the finding of a decrease in global strain using the CMR feature tracking technique. The T1 mapping technique showed a decrease in post-contrast T1 values and an increase in ECV values, which may be associated with a small amount of myocardial fibrosis following myocarditis. Previous studies (23,24) showed that acute myocarditis can lead to impaired CMR myocardial strain and increased ECV. Comparing between the results of other studies and ours revealed that myocardial damage and ventricular dysfunction in patients with NCAM during follow-up CMR had mildly abnormal parameters of ventricular volume, ejection fraction, myocardial strain, and ECV, suggesting the presence of myocardial fibrosis in the sequelae of myocarditis and reduction in degree. Studies have shown that myocardial strain and ejection fraction in patients with acute myocarditis correlate well with myocardial edema and delayed enhancement (25-27); however, in the follow-up period, myocardial strain and ejection fraction did not correlate well with delayed enhancement, and the correlation coefficient of GRS was the largest, which was −0.546. The possible reason for this finding is that during the follow-up period of recovery, both myocardial LGE and ventricular dysfunction were mild, and the functional parameters were susceptible to interference from other factors, including the baseline disease. During the follow-up in patients with NCAM, myocardial strain was helpful in differentiating the NCAM follow-up group from the control group, and the efficacy of strain was better in GLS but poor in GRS and GCS. However, the discriminability of GLS, GCS, and GRS were excellent between acute myocarditis and normal cohorts (26). During the follow-up, recovery of strain is observed but still with mild impairment, which is slightly different from the normal control group. This finding is likely the main reason for the decrease in efficacy and suggests that GLS was a more stable and sensitive indicator for detecting early dysfunction than GRS and GCS, which was also more severely impaired and slower to recover. Despite the diagnostic and evaluative values of the parameters based on the mapping technique in acute myocarditis (23), the discriminative value in the NCAM follow-up and control groups was not good. ECV yielded the best AUC of 0.685, indicating that a marked difference was not observed between the NCAM follow-up and control groups and suggesting better recovery of the myocardial injury. Combined RVEF, GRS, and GLS multiparameter showed better diagnostic efficacy than sole GLS; however, the difference was subtle compared with the diagnostic efficacy of GLS. This finding suggested that RVEF and GRS did not markedly improve the overall efficacy, and the impairment of GLS was the most prominent, which had the greatest impact on the efficacy.
Some studies (9,10) have shown that patients with NCAM during follow-up had impaired myocardial strain compared with normal controls via echocardiogram, whereas the recovery from the myocardial injury was unclear. In our study, we performed serial follow-up CMR, which was a more reliable method for assessing the recovery or progression of myocardial injury and ventricular dysfunction. In this study, no statistically significant difference in myocardial characteristics, structure, and function was observed between the three different follow-up timepoints at baseline, 3 months, and 6 months of acute myocarditis, suggesting that most parameters of the cardiac remodeling, myocardial injury, and dysfunction caused by acute myocarditis are not completely reversible. Although without statistically significant difference, LGE extent was higher at first and follow-up compared to baseline, there were four acute myocarditis patients who evolved to chronic myocarditis, this could influence the results when the sample size was 12, and LGE extent alterations was opposite to other studies (2-4). This finding is likely related to the mild cardiac remodeling, myocardial injury, and dysfunction in several cases, and the subtle differences during follow-up are easily affected by other factors, including the positioning of scan and normal fluctuations in functional assessment in different conditions. The medium- and long-term follow-up groups showed decreased native T1 and T2 values compared with the short-term follow-up group, suggesting that myocardial injury has not yet recovered in the short-term, and the degree of recovery was more significant after 6 months. In particular, the short-term T2 value was higher than the baseline level although without a statistical difference, indicating that myocardial edema was still present, or different positioning level, scanners, and monitoring myocardial injury remained necessary, which was conducive to a more accurate and effective clinical management.
In recent years, CMR can be used for evaluating myocardial edema, hyperemia, and necrosis in CAM, as well as residual myocardial damage and decreased cardiac function (23); however, whether a difference exists between NCAM and CAM in the course of follow-up remains unclear. At the short-term follow-up, the CAM and NCAM groups showed increased ventricular volume, decreased ejection fraction, and myocardial strain compared with the control group; however, the difference between the CAM and NCAM groups was not statistically significant. Notably, LVEF remained in the normal range in both case groups; however, myocardial strain was impaired, suggesting subclinical dysfunction. This finding is consistent with those noted in patients with acute myocarditis (26,27). At the short-term follow-up, RVEF was near the lower limit of normal or mildly reduced, suggesting a more persistent impairment of right ventricular function by myocarditis. Studies showed that in patients who recovered from COVID-19, an increase in ECV values was noted compared with the control group (28,29). In our study, the CAM group had higher ECV and T2 values than the NCAM group, indicating a more significant myocardial edema, which also indicated that ECV was more robust between scanners than native and post T1 values. In discriminating between the two case groups, only T2 values reached a good discriminating ability, which may be related to the fact that some patients in the CAM group were followed up for a shorter period (2–4 weeks), whereas those in the NCAM group were followed up for 3 months. The best discriminator between the two case groups and the control group was GLS, indicating that GLS continues to be of optimal value in determining subclinical dysfunction during short-term follow-up for patients with CAM and NCAM.
The extent of LGE decreased during follow-up (19), and LGE in patients with COVID-19 vaccine-associated myocarditis existed through short- and medium-term follow-up (11); however, whether a difference exists when compared with patients with NCAM during follow-up has not been clarified. One study on children with COVID-19 vaccine-associated myopericarditis revealed no evidence of active inflammation or ventricular dysfunction on intermediate CMR (30); however, minority had persistent LGE. Intermediate findings in COVID-19 vaccine-associated myopericarditis revealed less LGE burden compared with classic myocarditis. However, some studies have shown that there was little difference in CMR performance and clinical outcomes between CAM and NCAM during acute onset (31). Moreover, there were studies suggesting that patients with COVID-19 vaccine-associated myocarditis experience less severe myocardial injury than those with CAM (21). The NCAM short-term follow-up group had more LGE and a slight increase in consecutive follow-up than the CAM short-term follow-up group; however, no statistical significance was noted. In addition to the small sample size of this study, the CMR parameters of severe or chronic myocarditis may have affected the overall results.
There are some limitations in this study. First, it was a single-center study with a small sample size, and some bias may exist. Second, the number of cases with complete consecutive CMR data at baseline, 3 months, and 6 months was small, with only 11 cases; therefore, our results should be further confirmed by the results of multicenter studies with larger sample sizes. Third, this study used two scanners of 3.0T MRI for CMR imaging, which may affect the normal range of T1 and T2 mapping results. Different field strength, sequences may also affect T1 and T2 mapping parameters and thresholds (32). Additionally, the follow-up time of CMR in patients with CAM was inconsistent and varied considerably, and the presence of COVID-19 re-infections remained unknown owing to the fact that occurred during the COVID-19 pandemic. At last, different aetiologies and therapeutic managements could influence cardiac function.
Conclusions
In conclusion, in the follow-up of myocarditis, patients with NCAM developed residual myocardial injury, cardiac remodeling, and dysfunction, and patients who underwent medium- and long-term follow-up had improved myocardial edema compared with those who underwent short-term follow-up by CMR. In patients who underwent short-term follow-up of myocarditis, those with CAM showed more severe myocardial edema than those with NCAM. This study revealed the pattern of cardiac structure, function, and myocardial characteristics in the consecutive follow-up of myocarditis, which may contribute to the clinical assessment and management of follow-up for patients with myocarditis.
Acknowledgments
Funding: This study was funded by a project grant from
Footnote
Reporting Checklist: The authors have completed the STROBE reporting checklist. Available at https://qims.amegroups.com/article/view/10.21037/qims-24-1422/rc
Conflicts of Interest: All authors have completed the ICMJE uniform disclosure form (available at https://qims.amegroups.com/article/view/10.21037/qims-24-1422/coif). The authors have no conflicts of interest to declare.
Ethical Statement: The authors are accountable for all aspects of the work in ensuring that questions related to the accuracy or integrity of any part of the work are appropriately investigated and resolved. The study was conducted in accordance with the Declaration of Helsinki (as revised in 2013). The study was approved by the institutional ethics committee of Affiliated Jinhua Hospital, Zhejiang University School of Medicine (No. 2019-171) and informed consent was taken from all the patients.
Open Access Statement: This is an Open Access article distributed in accordance with the Creative Commons Attribution-NonCommercial-NoDerivs 4.0 International License (CC BY-NC-ND 4.0), which permits the non-commercial replication and distribution of the article with the strict proviso that no changes or edits are made and the original work is properly cited (including links to both the formal publication through the relevant DOI and the license). See: https://creativecommons.org/licenses/by-nc-nd/4.0/.
References
- Mavrogeni S, Spargias C, Bratis C, Kolovou G, Markussis V, Papadopoulou E, Constadoulakis P, Papadimitropoulos M, Douskou M, Pavlides G, Cokkinos D. Myocarditis as a precipitating factor for heart failure: evaluation and 1-year follow-up using cardiovascular magnetic resonance and endomyocardial biopsy. Eur J Heart Fail 2011;13:830-7. [Crossref] [PubMed]
- Caforio AL, Pankuweit S, Arbustini E, Basso C, Gimeno-Blanes J, Felix SB, et al. Current state of knowledge on aetiology, diagnosis, management, and therapy of myocarditis: a position statement of the European Society of Cardiology Working Group on Myocardial and Pericardial Diseases. Eur Heart J 2013;34:2636-48, 2648a-2648d.
- Schulz-Menger J, Bluemke DA, Bremerich J, Flamm SD, Fogel MA, Friedrich MG, Kim RJ, von Knobelsdorff-Brenkenhoff F, Kramer CM, Pennell DJ, Plein S, Nagel E. Standardized image interpretation and post-processing in cardiovascular magnetic resonance - 2020 update: Society for Cardiovascular Magnetic Resonance (SCMR): Board of Trustees Task Force on Standardized Post-Processing. J Cardiovasc Magn Reson 2020;22:19. [Crossref] [PubMed]
- Blissett S, Chocron Y, Kovacina B, Afilalo J. Diagnostic and prognostic value of cardiac magnetic resonance in acute myocarditis: a systematic review and meta-analysis. Int J Cardiovasc Imaging 2019;35:2221-9. [Crossref] [PubMed]
- Friedrich MG, Sechtem U, Schulz-Menger J, Holmvang G, Alakija P, Cooper LT, White JA, Abdel-Aty H, Gutberlet M, Prasad S, Aletras A, Laissy JP, Paterson I, Filipchuk NG, Kumar A, Pauschinger M, Liu PInternational Consensus Group on Cardiovascular Magnetic Resonance in Myocarditis. Cardiovascular magnetic resonance in myocarditis: A JACC White Paper. J Am Coll Cardiol 2009;53:1475-87. [Crossref] [PubMed]
- Ferreira VM, Schulz-Menger J, Holmvang G, Kramer CM, Carbone I, Sechtem U, Kindermann I, Gutberlet M, Cooper LT, Liu P, Friedrich MG. Cardiovascular Magnetic Resonance in Nonischemic Myocardial Inflammation: Expert Recommendations. J Am Coll Cardiol 2018;72:3158-76. [Crossref] [PubMed]
- Ammirati E, Veronese G, Cipriani M, Moroni F, Garascia A, Brambatti M, Adler ED, Frigerio M. Acute and Fulminant Myocarditis: a Pragmatic Clinical Approach to Diagnosis and Treatment. Curr Cardiol Rep 2018;20:114. [Crossref] [PubMed]
- Ammirati E, Frigerio M, Adler ED, Basso C, Birnie DH, Brambatti M, Friedrich MG, Klingel K, Lehtonen J, Moslehi JJ, Pedrotti P, Rimoldi OE, Schultheiss HP, Tschöpe C, Cooper LT Jr, Camici PG. Management of Acute Myocarditis and Chronic Inflammatory Cardiomyopathy: An Expert Consensus Document. Circ Heart Fail 2020;13:e007405. [Crossref] [PubMed]
- Caspar T, Fichot M, Ohana M, El Ghannudi S, Morel O, Ohlmann P. Late Detection of Left Ventricular Dysfunction Using Two-Dimensional and Three-Dimensional Speckle-Tracking Echocardiography in Patients with History of Nonsevere Acute Myocarditis. J Am Soc Echocardiogr 2017;30:756-62. [Crossref] [PubMed]
- Manalo R, Bourget L, Garg A, Swaminathan S. Does myocardial strain remain abnormal long after normalization of ejection fraction in patients with acute myocarditis? Echocardiography 2019;36:609-12. [Crossref] [PubMed]
- Samimisedeh P, Jafari Afshar E, Tayebi A, Rastad H. Post-acute midterm follow-up cardiac MRI findings and clinical outcomes in patients with COVID-19 vaccine-associated myocarditis: a comprehensive systematic review and meta-analysis. Infect Dis (Lond) 2024;56:193-205. [Crossref] [PubMed]
- Haussner W, DeRosa AP, Haussner D, Tran J, Torres-Lavoro J, Kamler J, Shah K. COVID-19 associated myocarditis: A systematic review. Am J Emerg Med 2022;51:150-5. [Crossref] [PubMed]
- Atri L, Morgan M, Harrell S, AlJaroudi W, Berman AE. Role of cardiac magnetic resonance imaging in the diagnosis and management of COVID-19 related myocarditis: Clinical and imaging considerations. World J Radiol 2021;13:283-93. [Crossref] [PubMed]
- Writing Committee. Gluckman TJ, Bhave NM, Allen LA, Chung EH, Spatz ES, Ammirati E, Baggish AL, Bozkurt B, Cornwell WK 3rd, Harmon KG, Kim JH, Lala A, Levine BD, Martinez MW, Onuma O, Phelan D, Puntmann VO, Rajpal S, Taub PR, Verma AK. 2022 ACC Expert Consensus Decision Pathway on Cardiovascular Sequelae of COVID-19 in Adults: Myocarditis and Other Myocardial Involvement, Post-Acute Sequelae of SARS-CoV-2 Infection, and Return to Play: A Report of the American College of Cardiology Solution Set Oversight Committee. J Am Coll Cardiol 2022;79:1717-56. [Crossref] [PubMed]
- Pan C, Zhang Z, Luo L, Wu W, Jia T, Lu L, Liu WV, Qin Y, Hu F, Ding X, Qin P, Qian L, Chen J, Li S. Cardiac T1 and T2 Mapping Showed Myocardial Involvement in Recovered COVID-19 Patients Initially Considered Devoid of Cardiac Damage. J Magn Reson Imaging 2021;54:421-8. [Crossref] [PubMed]
- Viskin D, Topilsky Y, Aviram G, Mann T, Sadon S, Hadad Y, Flint N, Shmilovich H, Banai S, Havakuk O. Myocarditis Associated With COVID-19 Vaccination: Echocardiography, Cardiac Tomography, and Magnetic Resonance Imaging Findings. Circ Cardiovasc Imaging 2021;14:e013236. [Crossref] [PubMed]
- Salerno M, Kwong RY. CMR in the Era of COVID-19: Evaluation of Myocarditis in the Subacute Phase. JACC Cardiovasc Imaging 2020;13:2340-2. [Crossref] [PubMed]
- Galea N, Cundari G, Di Dedda E, Chimenti C, Aquaro GD, Barison A, et al. Short term outcome of myocarditis and pericarditis following COVID-19 vaccines: a cardiac magnetic resonance imaging study. Int J Cardiovasc Imaging 2023;39:1031-43. [Crossref] [PubMed]
- Shiyovich A, Plakht Y, Witberg G, Aviv Y, Shafir G, Kornowski R, Hamdan A. Myocarditis Following COVID-19 Vaccination: A Follow-up Magnetic Resonance Imaging Study. JACC Cardiovasc Imaging 2022;15:2006-7. [Crossref] [PubMed]
- Vaiyani D, Elias MD, Biko DM, Whitehead KK, Harris MA, Partington SL, Fogel MA. Patients with Post-COVID-19 Vaccination Myocarditis Have More Favorable Strain in Cardiac Magnetic Resonance Than Those With Viral Myocarditis. Pediatr Cardiol 2023;44:1108-17. [Crossref] [PubMed]
- Ishisaka Y, Watanabe A, Aikawa T, Kanaoka K, Takagi H, Wiley J, Yasuhara J, Kuno T. Overview of SARS-CoV-2 infection and vaccine associated myocarditis compared to non-COVID-19-associated myocarditis: A systematic review and meta-analysis. Int J Cardiol 2024;395:131401. [Crossref] [PubMed]
- Guglin ME, Etuk A, Shah C, Ilonze OJ. Fulminant Myocarditis and Cardiogenic Shock Following COVID-19 Infection Versus COVID-19 Vaccination: A Systematic Literature Review. J Clin Med 2023;12:1849. [Crossref] [PubMed]
- Eichhorn C, Greulich S, Bucciarelli-Ducci C, Sznitman R, Kwong RY, Gräni C. Multiparametric Cardiovascular Magnetic Resonance Approach in Diagnosing, Monitoring, and Prognostication of Myocarditis. JACC Cardiovasc Imaging 2022;15:1325-38. [Crossref] [PubMed]
- Khanna S, Amarasekera AT, Li C, Bhat A, Chen HHL, Gan GCH, Ugander M, Tan TC. The utility of cardiac magnetic resonance imaging in the diagnosis of adult patients with acute myocarditis: a systematic review and meta-analysis. Int J Cardiol 2022;363:225-39. [Crossref] [PubMed]
- Luetkens JA, Schlesinger-Irsch U, Kuetting DL, Dabir D, Homsi R, Doerner J, Schmeel FC, Fimmers R, Sprinkart AM, Naehle CP, Schild HH, Thomas D. Feature-tracking myocardial strain analysis in acute myocarditis: diagnostic value and association with myocardial oedema. Eur Radiol 2017;27:4661-71. [Crossref] [PubMed]
- Chen X, Hu H, Pan J, Shu J, Hu Y, Yu R. Performance of cardiovascular magnetic resonance strain in patients with acute myocarditis. Cardiovasc Diagn Ther 2020;10:725-37. [Crossref] [PubMed]
- Urmeneta Ulloa J, Martínez de Vega V, Álvarez Vázquez A, Andreu-Vázquez C, Thuissard-Vasallo IJ, Recio Rodríguez M, Pizarro G, Cabrera JÁ. Comparative Cardiac Magnetic Resonance-Based Feature Tracking and Deep-Learning Strain Assessment in Patients Hospitalized for Acute Myocarditis. J Clin Med 2023;12:1113. [Crossref] [PubMed]
- Li X, Wang H, Zhao R, Wang T, Zhu Y, Qian Y, Liu B, Yu Y, Han Y. Elevated Extracellular Volume Fraction and Reduced Global Longitudinal Strains in Participants Recovered from COVID-19 without Clinical Cardiac Findings. Radiology 2021;299:E230-40. [Crossref] [PubMed]
- Luetkens JA, Isaak A, Zimmer S, Nattermann J, Sprinkart AM, Boesecke C, Rieke GJ, Zachoval C, Heine A, Velten M, Duerr GD. Diffuse Myocardial Inflammation in COVID-19 Associated Myocarditis Detected by Multiparametric Cardiac Magnetic Resonance Imaging. Circ Cardiovasc Imaging 2020;13:e010897. [Crossref] [PubMed]
- Dove ML, Slesnick TC, Oster ME, Hashemi S, Patel T, Wilson HC. Cardiac Magnetic Resonance Findings of Coronavirus Disease 2019 (COVID-19) Vaccine-Associated Myopericarditis at Intermediate Follow-Up: A Comparison with Classic Myocarditis. J Pediatr 2023;260:113462. [Crossref] [PubMed]
- Kim SG, Lee JY, Jeong WG, Lee JE, Kim YH. Cardiac Magnetic Resonance Imaging Findings and Clinical Features of COVID-19 Vaccine-Associated Myocarditis, Compared With Those of Other Types of Myocarditis. J Korean Med Sci 2024;39:e42. [Crossref] [PubMed]
- Messroghli DR, Moon JC, Ferreira VM, Grosse-Wortmann L, He T, Kellman P, Mascherbauer J, Nezafat R, Salerno M, Schelbert EB, Taylor AJ, Thompson R, Ugander M, van Heeswijk RB, Friedrich MG. Clinical recommendations for cardiovascular magnetic resonance mapping of T1, T2, T2* and extracellular volume: A consensus statement by the Society for Cardiovascular Magnetic Resonance (SCMR) endorsed by the European Association for Cardiovascular Imaging (EACVI). J Cardiovasc Magn Reson 2017;19:75. [Crossref] [PubMed]