HIF-1α expression is associated with the pathological response to neoadjuvant chemotherapy in pancreatic ductal adenocarcinoma patients and can be predicted using CECT features
Introduction
Neoadjuvant chemotherapy (NAC) has emerged as a viable regimen for patients with resectable and borderline resectable pancreatic ductal adenocarcinoma (PDAC). NAC increases the margin-negative resection rate, slows the development of micrometastasis, and identifies aggressive tumors, thus sparing patients from futile surgery (1). However, an accurate evaluation of the response to NAC is a prerequisite if patients are to reap these benefits.
Carbohydrate antigen 19-9 (CA19-9) is an important serum biomarker for the clinical assessment of the response to PDAC therapy; however, its low specificity limits its application (2). Due to the complex morphological and histological nature of PDAC, NAC-induced tumor cell injury in PDAC is mainly reflected by “isovolumetric” tissue replacement through fibrosis rather than volume loss (3). Therefore, the Response Evaluation Criteria in Solid Tumors cannot accurately assess the response of PDAC patients to NAC (4). In addition, PDAC is highly desmoplastic, and lacks a clear distinction between post-treatment fibrosis and pretreatment desmoplasia (5). Thus, fibrosis alone cannot be considered evidence of tumor regression. To date, the biomarkers for effective treatment in NAC regimens have not yet been identified (6).
PDAC cell nests are scattered and arranged in a dense fibrous matrix. Hypovascularity limits oxygen delivery to tumors, contributing to a microenvironment characterized by hypoxia (7). Hypoxia-inducible factor-1-alpha (HIF-1α) is considered a vital marker for assessing pathological responses to cancer, as it regulates the expression of genes that contributes to tumor cell survival, invasiveness, and angiogenesis, and is also the master transcriptional regulator of cellular oxygen homeostasis (8,9). Ramírez-Tortosa et al. (10) analyzed the predictive value of HIF-1α in breast cancer patients receiving NAC, and reported that more than one-third of patients were considered HIF-1α positive, and tumors overexpressing HIF-1α were more likely to achieve a complete pathological response. Conversely, in a study of cervical cancer, Zhu et al. (11) found that the expression of HIF-1α was negative in patients with a complete response and positive in patients with a partial response after NAC. However, to date, the relationship between the expression level of HIF-1α and the pathological response of PDAC patients receiving NAC has not been examined. Currently, HIF-1α expression is determined histopathologically from specimens obtained through biopsy or surgery. However, biopsy carries risks of pancreatitis and needle tract seeding, and is considered unreliable because of the heterogeneity of PDAC (12,13). Thus, reliable methods for evaluating the treatment response of PDAC patients to NAC need to be established (3).
Non-invasive medical imaging techniques play an integral role in the diagnosis, tumor node metastasis (TNM) staging, assessment of therapeutic efficacy, and prognostic prediction of PDAC. Due to its high temporal and spatial resolution and wide anatomical coverage, computed tomography (CT) is widely used as the preferred imaging modality for PDAC (14). Radiologists can determine the biological behavior and microenvironment of a tumor based on characteristics such as size, location, morphology, enhancement, and the degree of invasion of the surrounding tissues (15,16). However, to date, no relevant studies on the prediction of HIF-1α expression in tumors using contrast-enhanced computed tomography (CECT) appear to have been conducted. Thus, the present study sought to examine the correlation between HIF-1α expression and the pathological response of PDAC patients receiving NAC, and to predict HIF-1α expression using CECT features. We present this article in accordance with the STROBE reporting checklist (available at https://qims.amegroups.com/article/view/10.21037/qims-24-103/rc).
Methods
Patients and treatment management
We retrospectively collected the data of patients with pathologically confirmed PDAC who underwent NAC followed by radical surgery at three centers (Qilu Hospital of Shandong University, Shandong Provincial Hospital Affiliated to Shandong First Medical University and The Affiliated Hospital of Qingdao University) between January 2017 and February 2023. To be eligible for inclusion in this study, the patients had to meet the following inclusion criteria: (I) have received NAC treatment before surgery; (II) have intact paraffin-embedded tissue blocks and postoperative pathological results of PDAC available from the pathology department; (III) have complete clinical data; and (IV) have undergone pre- and post-NAC CECT, including complete 1 mm–thin slice reconstructed images of the non-enhanced phase (NP), arterial phase (AP), portal venous phase (PVP), and delay phase (DP). Patients were excluded from the study if they met any of the following exclusion criteria: (I) had a lesion too small to be clearly observed by CECT; (II) had unavailable CECT images or poor-quality images; (III) had received any type of anti-tumor therapy other than NAC before surgery; and (IV) had HIF-1α pathological section staining that failed to meet the assessment criteria. The screening process is shown in Figure 1. Among the patients, 40 received two to eight cycles of the nab-paclitaxel combined with gemcitabine (AG) regimen [gemcitabine 1,000 mg/m2 intravenous glucose tolerance test (IVGTT), days 1 and 8; albumin-bound paclitaxel 125 mg/m2 IVGTT, days 1 and 8; repeated every 3 weeks) (17), 10 patients received one to seven cycles of the gemcitabine and S-1 (tegafur, gimeracil and oteracil potassium capsules) (GS) regimen (gemcitabine 1,000 mg/m2 IVGTT, days 1 and 8; S-1 60, 80, or 100 mg/d according to body surface area) (18), and 8 patients received two to six cycles of the FOLFIRINOX regimen [oxaliplatin 85 mg/m2 IVGTT, day 1; irinotecan 180 mg/m2 IVGTT, day 1; calcium folinate 400 mg/m2 IVGTT, day 1; 5-fluorouracil (5-FU) 400 mg/m2 bolus day 1; followed by 5-FU 2,400 mg/m2 continuous infusion over 46 h; repeated every 2 weeks] (17). Additionally, the rate of change of the serum tumor markers CA19-9, carcinoembryonic antigen (CEA), and carbohydrate antigen 125 (CA125) were calculated, and are expressed as %Δ [(post-NAC – pre-NAC)/pre-NAC], where pre-NAC was the value of the last serum tumor markers before NAC, and post-NAC was the value of the last serum tumor markers before the operation.
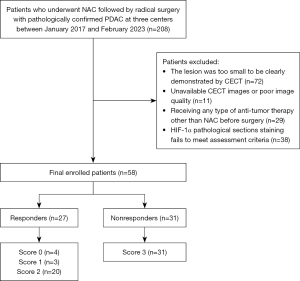
The study was conducted in accordance with the Declaration of Helsinki (as revised in 2013) and was approved by the Ethics Committee of Qilu Hospital, Shandong University (No. 2021/255). The requirement of individual consent for this retrospective analysis was waived.
Histology and pathological evaluation of response
Histological examinations of all patients were performed on slides stained with hematoxylin and eosin. Two pathologists with more than 6 years of clinical experience each, who were blinded to the clinical parameters, used a biological microscope (magnification, ×400; BX53, Olympus, Japan) to observe the images. In cases of disagreement, a senior physician with more than 10 years of clinical experience resolved the issue. Pathological evaluation outcomes and features included tumor differentiation, perineural invasion, microvascular invasion, nuclear-associated antigen (Ki-67), and TNM staging (19). The histopathological response of the PDAC patients following NAC was then assessed using the tumor regression grading system recommended by the College of American Pathologists (20). The PDAC patients who underwent surgical resection after NAC were divided into the following two groups: responders (who received a score of 0, indicating no viable cancer cells; a score of 1, indicating single cells or small groups of cancer cells; or a score of 2, indicating residual cancer outgrown by fibrosis); and non-responders (who received a score of 3, indicating minimal or no tumors killed or extensive residual cancer).
Immunohistochemistry
The tissue sections were deparaffinized with xylene and hydrated in gradient alcohol. The primary antibody HIF-1α (rabbit polyclonal antibody, diluted 1:200, Boster, Wuhan, PB9253) and Ki-67 (recombinant rabbit monoclonal antibody, diluted 1:5,000, HuaBio, Hangzhou, China; HA721115) were incubated overnight at 4 ℃. The sections were then treated with an immunodetection solution consisting of biotinylated secondary antibody for 60 minutes. A DAB kit (Zhong Shan-Golden Bridge Biological Technology Co., Ltd., Beijing, China; ZLI9018) was used as a chromogenic agent, and the nuclei of the sections were counterstained with hematoxylin. Simultaneously, the primary antibody was replaced with phosphate buffer as a negative control.
Evaluation of immunohistochemical staining
The immunohistochemical expression scoring was performed by two pathologists with more than 6 years of experience each, who were blinded to the clinicopathological data. Any disagreements were resolved by a senior physician with more than 10 years of experience. Five random fields were observed under a light microscope, and analyzed using a semi-quantitative method to evaluate the intensity of staining and the percentage of positively stained cells (21). Staining intensity was scored as follows: 0 (none), 1 (weak), 2 (moderate), and 3 (strong). While the percentage of positive staining in the five separate areas was scored as follows: 0 (<5%), 1 (5–25%), 2 (26–50%), 3 (51–75%), and 4 (>75%). The final weighted score was calculated by multiplying the staining intensity score with the percentage of positive staining. The mean value of the weighted score was 5; hence, a weighted score of 0, 1, 2, 3, or 4 was defined as low HIF-1α expression, and a weighted score of 6, 8, 9, or 12 was defined as high HIF-1α expression.
CECT scanning
All patients were instructed to fast for at least 8 hours before the CT examination. The scanning parameters for each center were as follows: center 1 (Brilliance iCT, Philips Healthcare, the Netherlands); tube voltage: 120 kV; tube current: auto mA; beam collimation: 128 mm × 0.625 mm; helical pitch: 0.993; matrix: 512×512; slice thickness: 5 mm; rotation time: 0.5 s; center 2 (CT750 HD; GE Healthcare, Wisconsin, USA); tube voltage: 120 kV; tube current: auto mA; slice thickness: 5 mm; matrix: 512×512; and center 3 (GE-Revolution EVO CT Scanner; Wisconsin, USA); tube voltage: 120 kV; tube current: auto mA; slice thickness: 5 mm; reconstruction interval: 1 mm; matrix: 512×512; rotation time: 0.5–0.8 s. After plain scanning, all patients were injected with 1.5 mL/kg of contrast agent (Ultravist 300 mg I/mL, Bayer Healthcare, Germany) through the elbow vein using a high-pressure syringe at an injection rate of 3 mL/s, followed by normal saline. The AP, PVP, and DP scans were performed at 25–30, 60–70, and 110–180 s, respectively, following the injection of contrast medium.
Imaging analysis
All findings on both the pre- and post-NAC multiphase CT scans were independently reviewed on the picture archiving and communication system by two radiologists (each with more than 7 years of experience in abdominal radiology). Any discrepancies between the results of the two reviewers were resolved by a third reviewer (with more than 10 years of experience). All the reviewers involved in the assessment were blinded to the clinical-surgical-pathologic results. According to the standardized reporting template in the Society of Abdominal Radiologists and the American Pancreatic Association Consensus Statement (22), the radiologists recorded the tumor location (22), tumor morphology (round-like/irregular) (22), necrosis (no enhancement of tumoral tissue in both the AP and PVP) (23), calcification (24), peritumoral retention cysts (25), tumor proximity adipose tissue (25), main pancreatic duct dilatation (≥3 mm) (26), bile duct dilatation (extrahepatic bile ducts >8 mm; intrahepatic bile ducts >2 mm) (27), and adjacent pancreatic parenchymal atrophy (pancreatic thickness ≤7 mm) (28), and assessed the tumor’s relationship with the celiac, superior mesenteric, and common hepatic arteries, and superior mesenteric and portal veins as abutment (tumor vessel contact ≤180°) or encasement (tumor vessel contact >180°) (22), and noted the presence of suspicious regional lymph nodes (a short axis >1 mm, a round morphology, heterogeneity, or central necrosis) (22). Subsequently, CT values [Hounsfield unit (HU)] measurements of the tumor in the NP, AP, PVP, and DP were performed on the CECT images. The region of interest (ROI) was drawn in the region where the tumor enhancement was most noticeable. The same plane of the image was selected for measurement whenever possible, and areas of visible necrosis, blood vessels, surrounding normal pancreatic tissue, and adipose tissue were avoided (Figure 2A-2E). The mean values of measurements repeated three times were used for analysis. The ratios of the NP, AP, PVP, and DP to the abdominal aorta were calculated separately to express the CT values for each phase. Pre-NAC was the CT value of the last CT examination before NAC, and post-NAC was the CT value of the last CT examination before surgery.
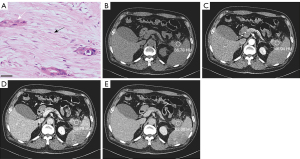
Statistical analysis
The Chi-square test was used to examine the association between the pathological response and the HIF-1α variables. Univariate and multivariate binary logistic regression analyses were conducted to identify the factors correlated with the PDAC patients’ pathological response to NAC, including clinical, pathological, and imaging characteristics. The receiver operating characteristic (ROC) curve was used to evaluate the optimal predictor, and the area under the curve (AUC), 95% confidence interval (CI) of the AUC, sensitivity, specificity, positive predictive value, negative predictive value, and Youden’s index were calculated. Low predictive efficacy was defined as 0.5< AUC ≤0.7, moderate predictive efficacy was defined as 0.7< AUC ≤0.9, and high predictive performance was defined as an AUC >0.9. The statistical analysis was carried out using SPSS software (version 26; IBM Corporation, USA) and Prism (version 9.5.0; GraphPad, Inc, San Diego, CA, USA). Patients with missing values were excluded from the subsequent analyses. The data for the continuous variables are reported as the mean ± standard deviation (SD), or as the median and range. The data for the continuous categorical variables are presented as the number of patients and percentage. P values <05 were considered statistically significant.
Results
Study population
The final study population comprised 58 patients. Among the patients, 27 (46.55%) responders and 31 (53.45%) non-responders were identified via histopathological examination, and 55.17% (n=32) of the patients were male and 44.83% (n=26) of the patients were female. The median patient age was 57 years (range, 30–70 years). Details of the patients’ characteristics are presented in Table 1.
Table 1
Variables | Values (n=58) |
---|---|
Gender | |
Male | 32 (55.17) |
Female | 26 (44.83) |
Age (years) | 56.66±8.52 |
Pathological response | |
0 | 4 (6.90) |
1 | 3 (5.17) |
2 | 20 (34.48) |
3 | 31 (53.45) |
Regimen of NAC | |
AG | 40 (68.97) |
GS | 10 (17.24) |
FOLFIRINOX | 8 (13.79) |
Nuclear HIF-1α expression | |
Nuclear HIF-1α-low | 28 (48.28) |
Nuclear HIF-1α-high | 30 (51.72) |
Data are presented as the mean ± standard deviation or number (percentage). NAC, neoadjuvant chemotherapy; AG, gemcitabine plus albumin-bound paclitaxel; GS, gemcitabine plus S-1; FOLFIRINOX, oxaliplatin irinotecan calcium folinate and 5-FU; HIF-1α, hypoxia-inducible factor-1-alpha; 5-FU, 5-fluorouracil.
Expression of HIF-1α in PDAC patients after NAC for different pathologic responses
Intratumoral HIF-1α in PDAC after NAC was expressed in the cytoplasm, nucleus, and stroma. Nuclear HIF-1α and cytoplasmic HIF-1α expression were higher in the responders than the non-responders, and the differences were statistically significant (P<0.001 and P=0.036, respectively). However, there was no statistically significant difference in HIF-1α expression in overall (cytoplasmic and nuclear) and PDAC stromal tissues (P=0.864 and P=0.636, respectively). The results are illustrated in Figure 3A-3F and Table 2.
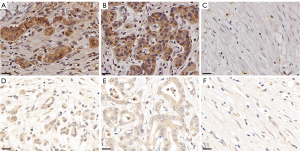
Table 2
Staining site | HIF-1α positive rate | Responders, n (%) | Non-responders, n (%) | P value |
---|---|---|---|---|
Nucleus | Low, ≤4 | 5 (18.52) | 23 (74.19) | <0.001 |
High, ≥6 | 22 (81.48) | 8 (25.81) | ||
Cytoplasm | Low, ≤4 | 2 (7.41) | 9 (29.03) | 0.036 |
High, ≥6 | 25 (92.59) | 22 (70.97) | ||
Stroma | Low, ≤4 | 18 (66.67) | 20 (64.52) | 0.864 |
High, ≥6 | 9 (33.33) | 11 (35.48) | ||
Overall | Low, ≤4 | 8 (29.63) | 11 (35.48) | 0.636 |
High, ≥6 | 19 (70.37) | 20 (64.52) |
HIF-1α, hypoxia-inducible factor-1α.
Relationship between patient characteristics and the PDAC pathological response following NAC
The multivariate analysis revealed that only %ΔCA19-9 [odds ratio (OR) =9.005, P=0.037], tumor differentiation (OR =0.005, P=0.044), and nuclear HIF-1α expression (OR =0.352, P=0.018) were independent predictors for pathological response following NAC, and the other clinical characteristics were not statistically significant (Table 3).
Table 3
Variables | Univariate analysis | Multivariate analysis | |||
---|---|---|---|---|---|
OR (95% CI) | P | OR (95% CI) | P | ||
Gender (male/female) | 1.813 (0.633–5.191) | 0.267 | |||
Age (year) | 0.938 (0.876–1.004) | 0.064 | |||
BMI (kg/m2) | 0.998 (0.833–1.196) | 0.986 | |||
Diabetes (yes/no) | 1.486 (0.397–5.555) | 0.556 | |||
Hypertension (yes/no) | 0.327 (0.095–1.125) | 0.076 | |||
Smoking (yes/no) | 0.818 (0.268–2.493) | 0.724 | |||
Drinking (yes/no) | 0.810 (0.274–2.396) | 0.703 | |||
Post-NAC lymphocyte ratio | 0.989 (0.943–1.037) | 0.650 | |||
Post-NAC monocyte ratio | 1.050 (0.919–1.200) | 0.474 | |||
Post-NAC neutrophil ratio | 1.025 (0.994–1.057) | 0.118 | |||
Post-NAC albumin (g/L) | 0.987 (0.918–1.061) | 0.730 | |||
Post-NAC globulin (g/L) | 1.025 (0.923–1.139) | 0.640 | |||
Post-NAC white-ball ratio | 0.936 (0.283–3.100) | 0.914 | |||
Pre-NAC CA19-9 (U/mL) | 0.998 (0.996–1.000) | 0.050 | |||
Post-NAC CA19-9 (U/mL) | 1.002 (0.999–1.006) | 0.229 | |||
%ΔCA19-9 | 3.345 (1.121–9.982) | 0.030 | 9.005 (1.145–70.788) | 0.037 | |
Pre-NAC CA125 (U/mL) | 1.001 (0.997–1.005) | 0.663 | |||
Post-NAC CA125 (U/mL) | 0.998 (0.991–1.006) | 0.702 | |||
%ΔCA125 | 0.914 (0.704–1.187) | 0.502 | |||
Pre-NAC CEA (ng/mL) | 0.983 (0.955–1.012) | 0.250 | |||
Post-NAC CEA (ng/mL) | 0.996 (0.984–1.007) | 0.470 | |||
%ΔCEA | 1.006 (0.643–1.574) | 0.978 | |||
Surgical time (hour) | 1.173 (0.872–1.577) | 0.291 | |||
Blood transfusion (yes/no) | 2.354 (0.817–6.779) | 0.113 | |||
Regimen of NAC (AG/other) | 0.636 (0.205–1.975) | 0.434 | |||
Differentiation (well/poor) | 0.222 (0.061–0.814) | 0.023 | 0.005 (0.000–0.863) | 0.044 | |
Perineural invasion (yes/no) | 2.088 (0.631–6.905) | 0.228 | |||
Microvascular invasion (yes/no) | 1.667 (0.404–6.870) | 0.480 | |||
Pathologic T staging (T1–T2/T3–T4) | 1.225 (0.338–4.443) | 0.758 | |||
Pathologic N staging (N0/N1–N2) | 1.917 (0.428–8.584) | 0.395 | |||
Maximal diameter (mm) | 1.011 (0.709–1.440) | 0.953 | |||
Ki-67 | 1.036 (0.984–1.091) | 0.180 | |||
Nuclear HIF-1α expression | 0.626 (0.506–0.775) | <0.001 | 0.352 (0.148–0.838) | 0.018 | |
Cytoplasmic HIF-1α expression | 0.770 (0.633–0.937) | 0.009 | 0.836 (0.615–1.137) | 0.255 | |
Stromal HIF-1α expression | 1.026 (0.896–1.176) | 0.707 | |||
Overall HIF-1α expression | 0.866 (0.723–1.037) | 0.118 |
OR, odds ratio; CI, confidence interval; BMI, body mass index; NAC, neoadjuvant chemotherapy; CA19-9, carbohydrate antigen 19-9; CA125, carbohydrate antigen 125; CEA, carcinoembryonic antigen; %Δ, [(post-NAC − pre-NAC)/pre-NAC]; AG, gemcitabine plus albumin-bound paclitaxel; GS, gemcitabine plus S-1; FOLFIRINOX, oxaliplatin irinotecan calcium folinate and 5-FU; HIF-1α, hypoxia-inducible factor-1α; PDAC, pancreatic ductal adenocarcinoma; 5-FU, 5-fluorouracil.
Analysis of the predictive value of %ΔCA19-9, tumor differentiation, and nuclear HIF-1α expression in post-NAC efficacy
The predictive performance of nuclear HIF-1α expression, %ΔCA19-9, and tumor differentiation for predicting the post-NAC pathological response is shown in Figure 4 and Table 4. Among them, nuclear HIF-1α expression had high predictive performance with an AUC of 0.873. The %ΔCA19-9 and tumor differentiation had low predictive efficacy with AUCs of 0.692 and 0.659, respectively. Additionally, a multivariate linear regression analysis based on the degree of the pathological response of PDAC patients after NAC revealed a negative correlation between nuclear HIF-1α expression and tumor differentiation (regression coefficients: 0.518 and 0.326, respectively) and a positive correlation between %ΔCA19-9 (regression coefficient: 0.337).
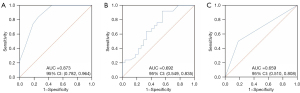
Table 4
Clinical features | AUC (95% CI) | Sensitivity | Specificity | PPV | NPV | Youden index |
---|---|---|---|---|---|---|
Nuclear HIF-1α expression | 0.873 (0.782–0.964) | 0.839 | 0.741 | 0.788 | 0.800 | 0.579 |
Tumor differentiation | 0.659 (0.510–0.808) | 0.500 | 0.818 | 0.789 | 0.545 | 0.318 |
%ΔCA19-9 | 0.692 (0.549–0.835) | 0.920 | 0.444 | 0.605 | 0.857 | 0.364 |
CI, confidence interval; %Δ, [(post-NAC − pre-NAC)/pre-NAC]; CA19-9, carbohydrate antigen 19-9; AUC, area under the curve; PPV, positive predicted value; NPV, negative predicted value; ROC, receiver operating characteristic; NAC, neoadjuvant chemotherapy; HIF-1α, hypoxia-inducible factor-1-alpha.
Comparison and predictive performance of CECT features between the nuclear HIF-1α-high and -low expression groups
In the univariate logistic regression analysis, the (post-NAC − pre-NAC) AP (OR =1.016, P=0.004) and the (post-NAC − pre-NAC) DP (OR =1.005, P=0.007) were the CT features predictive of nuclear HIF-1α, while in the multivariate logistic regression analysis, the (post-NAC − pre-NAC) AP was the only independent feature predictive of nuclear HIF-1α (OR =1.012, P=0.020). The results for the other CT features, including location, morphologic evaluation, tumor necrosis, calcification, peritumoral retention cysts, fatty infiltration, arterial invasion, venous invasion, pancreatic duct dilatation, bile duct dilatation, pancreatic atrophy, suspicious regional lymph nodes, and other CT values, were not statistically significant (Table 5). Further, a comparative analysis of the CT features of the (post-NAC − pre-NAC) AP and the (post-NAC − pre-NAC) DP was conducted between the nuclear HIF-1α-high and -low expression groups, and the results showed that the CT change value was significantly higher in the nuclear HIF-1α-high expression group than the nuclear HIF-1α-low expression group (P<0.001 and P=0.003, respectively) (Figure 5A,5B).
Table 5
Variables | Univariate analysis | Multivariate analysis | |||
---|---|---|---|---|---|
OR (95% CI) | P | OR (95% CI) | P | ||
Location (head/body, tail) | 1.133 (0.402–3.193) | 0.813 | |||
Morphologic evaluation (round/irregular) | 1.222 (0.412–3.622) | 0.717 | |||
Tumor necrosis (yes/no) | 0.667 (0.135–3.285) | 0.618 | |||
Calcification (yes/no) | 1.929 (0.165–22.528) | 0.600 | |||
Peritumoral retention cysts (yes/no) | 0.929 (0.122–7.080) | 0.943 | |||
Fatty infiltration (yes/no) | 0.530 (0.153–1.844) | 0.318 | |||
Arterial invasion (yes/no) | 0.810 (0.263–2.490) | 0.712 | |||
Venous invasion (yes/no) | 1.021 (0.356–2.926) | 0.969 | |||
Pancreatic duct dilatation (yes/no) | 0.833 (0.288–2.414) | 0,737 | |||
Bile duct dilatation (yes/no) | 0.574 (0.203–1.623) | 0.295 | |||
Pancreatic atrophy (yes/no) | 1.125 (0.395–3.201) | 0.825 | |||
Suspicious regional lymph nodes (yes/no) | 1.917 (0.428–8.584) | 0.395 | |||
Post-NAC NP (HU) | 0.997 (0.995–1.000) | 0.090 | |||
Post-NAC AP (HU) | 1.003 (0.993–1.013) | 0.527 | |||
Post-NAC PVP (HU) | 1.001 (0.996–1.006) | 0.740 | |||
Post-NAC DP (HU) | 1.002 (0.998–1.005) | 0.339 | |||
(Post-NAC − pre-NAC) NP (HU) | 0.999 (0.997–1.001) | 0.469 | |||
(Post-NAC – pre-NAC) AP (HU) | 1.016 (1.005–1.027) | 0.004 | 1.012 (1.002–1.023) | 0.020 | |
(Post-NAC − pre-NAC) PVP (HU) | 1.000 (0.996–1.004) | 0.997 | |||
(Post-NAC − pre-NAC) DP (HU) | 1.005 (1.001–1.009) | 0.007 | 1.004 (1.000–1.008) | 0.059 | |
Post-NAC AP − post-NAC NP (HU) | 1.003 (1.000–1.006) | 0.070 | |||
Post-NAC PVP − post-NAC NP (HU) | 1.002 (1.000–1.005) | 0.090 | |||
Post-NAC PVP − post-NAC AP (HU) | 1.000 (0.995–1.005) | 0.982 | |||
Post-NAC DP − post-NAC NP (HU) | 1.002 (1.000–1.004) | 0.067 | |||
Post-NAC DP − post-NAC AP (HU) | 1.001 (0.998–1.005) | 0.404 | |||
Post-NAC DP − post-NAC PVP (HU) | 1.001 (0.998–1.004) | 0.455 |
OR, odds ratio; CI, confidence interval; NAC, neoadjuvant chemotherapy; NP, nonenhanced phase; AP, arterial phase; PVP, portal venous phase; DP, delayed phase; HU, Hounsfield unit; CECT, contrast-enhanced computed tomography; HIF-1α, hypoxia-inducible factor-1-alpha.
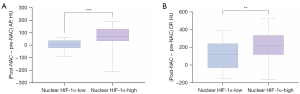
Discussion
HIF-1α belongs to the PAS (PER-ARNT-SIM) ubfamily of the basic helix-loop-helix family of transcription factors (29). Its expression is regulated by changes in the oxygen concentration in hypoxic tissues, and it is ubiquitously expressed in hypoxic tissues (30). PDAC is among the most hypoxic of all cancers (31). HIF-1α staining in PDAC was shown in the nucleus, cytoplasm and stroma as measured by immunohistochemistry as reported in previous studies (32-34). This finding is in line with our study. We found that HIF-1α was significantly higher in responders than in non-responders. This is likely because NAC usually induces extensive fibrosis in the tumor, adjacent pancreatic parenchyma, and peripancreatic tissues, and is particularly true for PDAC patients who showed marked response to NAC. Notably, this has been observed in PDAC patients who show a marked response to NAC (35). As stromal density and microvascular density are positive correlation (36). Severe fibrosis after NAC cooperates with the abnormal architecture of the intratumoral vasculature, causing vascular leakage, poor perfusion, and the formation of hypoxic areas (37). In addition, nuclear expression was shown to be an independent predictor of pathological response in the multifactorial logistic regression analysis. This could be due to the fact that in hypoxia, HIF-1α subunits are stabilized and translocated to the nucleus where they form a heterodimer with a subunit of HIF-1β encoded by the aryl hydrocarbon receptor nuclear translocator gene and bind to hypoxia response elements within the regulatory elements of the target genes to induce transcription (38).
In investigating the predictive performance of the CECT features for nuclear HIF-1α expression, we found that the (post-NAC − pre-NAC) AP was an independent predictor of HIF-1α expression. PDAC has different histopathological features, resulting in variations in CT enhancement patterns, such as differences in tumor vascularization and differences in the degree of interstitial fibrosis (39). Angiogenesis in solid tumor PDAC is initiated by vascular endothelial growth factor (VEGF), which is released by tumor cells in a hypoxic environment in response to HIF-1α (40). VEGF is commonly overexpressed in PDAC, leading to an increase in the number and density of micro vessels (34). Additionally, increased vascular space and/or increased blood flow in tumors with increased micro vessel numbers and density enhance the AP (41). Another possible explanation for the increased posttherapy perfusion is that slowing down the process of extracellular matrix accumulation in PDAC after NAC loosens the perivascular extracellular matrix scaffold, resulting in an increase in blood flow and blood volume on perfusion CT after NAC compared to that before NAC (42). In a hypoxic environment, HIF-1α activates VEGF, which binds to its receptor VEGFR-2 to stimulate the expression of the related transcription factors Twist and Snail. This results in the downregulation of the tight junction proteins between the epithelial cells, and the upregulation of the proteins related to angiogenesis. These proteins then arrange tumor cells through duct-like adhesions to form duct-like structures together with the extracellular matrix; eventually, these ducts infiltrate and extend into the vascular network for the blood transport of tumor cells. This process is called vasculogenic mimicry (VM) (43). Benjakul et al. (44) confirmed that blood vessel-like structures composed of cancer cells have a function similar to vessel perfusion in PDAC. Hence, the high microvascular numbers, density, and VM in PDAC appear to enhance the vascular perfusion characteristics, thereby contributing to the enhancement of the AP after NAC (45).
Additionally, in the univariate logistic regression analysis, we also found that the (post-NAC − pre-NAC) DP was able to predict nuclear HIF-1α expression. As mentioned above, fibrosis is induced in PDAC after NAC treatment. Fibers can widen the extracellular space and slow down the clearance of contrast medium, which is manifested as DP enhancement (46).
CA19-9 is a well-known serum biomarker linked to PDAC; however, its ability to predict the pathological response following NAC is disputed. Perri et al. (47) discovered that CA19-9 levels in PDAC patients after NAC were negatively correlated with the major pathological response, while Truty et al. (48) reported that normal levels of CA19-9 (<37 U/mL) after NAC could predict the pathological response to PDAC. However, in our multivariate logistic regression analysis, the NAC pathologic response was significantly associated with %ΔCA19-9, independent of the post-NAC CA19-9 levels. This is most likely due to the significant individual differences in the post-NAC CA19-9 levels, which made it impossible to accurately assess the efficacy of NAC. The %ΔCA19-9 indicated alterations in CA19-9 levels before and after NAC, with the exclusion of individual differences in patients (49). Therefore, in our view, %ΔCA19-9 was more valuable in assessing the pathological response of PDAC patients after NAC. Additionally, a large proportion of patients with PDAC may either be non-secretors or present with normal CA19-9 levels. Thus, the use of CA19-9 as a tool for assessing NAC response in PDAC patients remained limited, and needs to be further investigated, and standardized for optimal cut-off values (2).
Our study had several limitations. First, despite the strict inclusion and exclusion criteria, selection bias was inevitable due to the retrospective nature of the study. Second, the regimens and periods of NAC varied. Many patients only received a couple of NAC cycles; however, unless the level of unacceptable toxicity was reached, some patients received NAC for relatively long durations, until the tumors shrunk and margin-negative resection was deemed possible. Third, the sample size was relatively small. Despite these limitations, our study, which used real-world data, has important clinical value. Nevertheless, a multicenter, prospective study with a larger data set should be conducted to address the limitations of this study.
Conclusions
In this present study, we found that HIF-1α expression was the best predictor of the pathological response of PDAC patients to NAC, and the CECT feature of the (post-NAC − pre-NAC) AP had the best ability to predict HIF-1α expression among the different features. This study provides valuable information for assessing the pathological response to NAC of patients with PDAC and is expected to guide future therapies.
Acknowledgments
Funding: This research was supported by
Footnote
Reporting Checklist: The authors have completed the STROBE reporting checklist. Available at https://qims.amegroups.com/article/view/10.21037/qims-24-103/rc
Conflicts of Interest: All authors have completed the ICMJE uniform disclosure form (available at https://qims.amegroups.com/article/view/10.21037/qims-24-103/coif). The authors have no conflicts of interest to declare.
Ethical Statement: The authors are accountable for all aspects of the work in ensuring that questions related to the accuracy or integrity of any part of the work are appropriately investigated and resolved. The study was conducted in accordance with the Declaration of Helsinki (as revised in 2013) and was approved by the Ethics Committee of Qilu Hospital, Shandong University (No. 2021/255). The requirement of individual consent for this retrospective analysis was waived.
Open Access Statement: This is an Open Access article distributed in accordance with the Creative Commons Attribution-NonCommercial-NoDerivs 4.0 International License (CC BY-NC-ND 4.0), which permits the non-commercial replication and distribution of the article with the strict proviso that no changes or edits are made and the original work is properly cited (including links to both the formal publication through the relevant DOI and the license). See: https://creativecommons.org/licenses/by-nc-nd/4.0/.
References
- Springfeld C, Ferrone CR, Katz MHG, Philip PA, Hong TS, Hackert T, Büchler MW, Neoptolemos J. Neoadjuvant therapy for pancreatic cancer. Nat Rev Clin Oncol 2023;20:318-37. [Crossref] [PubMed]
- Valukas C, Chawla A. Measuring response to neoadjuvant therapy using biomarkers in pancreatic cancer: a narrative review. Chin Clin Oncol 2022;11:30. [Crossref] [PubMed]
- Yoo J, Lee JM, Joo I, Lee DH, Yoon JH, Yu MH, Jang JY, Lee SH. Post-neoadjuvant treatment pancreatic cancer resectability and outcome prediction using CT, (18)F-FDG PET/MRI and CA 19-9. Cancer Imaging 2023;23:49. [Crossref] [PubMed]
- Baliyan V, Kordbacheh H, Parakh A, Kambadakone A. Response assessment in pancreatic ductal adenocarcinoma: role of imaging. Abdom Radiol (NY) 2018;43:435-44. [Crossref] [PubMed]
- Hemmings C, Connor S. Pathological assessment of tumour regression following neoadjuvant therapy in pancreatic carcinoma. Pathology 2020;52:621-6. [Crossref] [PubMed]
- Verbeke C, Löhr M, Karlsson JS, Del Chiaro M. Pathology reporting of pancreatic cancer following neoadjuvant therapy: challenges and uncertainties. Cancer Treat Rev 2015;41:17-26. [Crossref] [PubMed]
- Sherman MH, Beatty GL. Tumor Microenvironment in Pancreatic Cancer Pathogenesis and Therapeutic Resistance. Annu Rev Pathol 2023;18:123-48. [Crossref] [PubMed]
- Colbert LE, Fisher SB, Balci S, Saka B, Chen Z, Kim S, El-Rayes BF, Adsay NV, Maithel SK, Landry JC, Curran WJ Jr. High nuclear hypoxia-inducible factor 1 alpha expression is a predictor of distant recurrence in patients with resected pancreatic adenocarcinoma. Int J Radiat Oncol Biol Phys 2015;91:631-9. [Crossref] [PubMed]
- Jin X, Dai L, Ma Y, Wang J, Liu Z. Implications of HIF-1α in the tumorigenesis and progression of pancreatic cancer. Cancer Cell Int 2020;20:273. [Crossref] [PubMed]
- Ramírez-Tortosa CL, Alonso-Calderón R, Gálvez-Navas JM, Pérez-Ramírez C, Quiles JL, Sánchez-Rovira P, Jiménez-Morales A, Ramírez-Tortosa M. Hypoxia-Inducible Factor-1 Alpha Expression Is Predictive of Pathological Complete Response in Patients with Breast Cancer Receiving Neoadjuvant Chemotherapy. Cancers (Basel) 2022;14:5393. [Crossref] [PubMed]
- Zhu P, Ou Y, Dong Y, Xu P, Yuan L. Expression of VEGF and HIF-1α in locally advanced cervical cancer: potential biomarkers for predicting preoperative radiochemotherapy sensitivity and prognosis. Onco Targets Ther 2016;9:3031-7. [Crossref] [PubMed]
- Sennerstam RB, Franzén BSH, Wiksell HOT, Auer GU. Core-needle biopsy of breast cancer is associated with a higher rate of distant metastases 5 to 15 years after diagnosis than FNA biopsy. Cancer Cytopathol 2017;125:748-56. [Crossref] [PubMed]
- Wang KX, Ben QW, Jin ZD, Du YQ, Zou DW, Liao Z, Li ZS. Assessment of morbidity and mortality associated with EUS-guided FNA: a systematic review. Gastrointest Endosc 2011;73:283-90. [Crossref] [PubMed]
- Rhee H, Park MS. The Role of Imaging in Current Treatment Strategies for Pancreatic Adenocarcinoma. Korean J Radiol 2021;22:23-40. [Crossref] [PubMed]
- Lee ES, Lee JM. Imaging diagnosis of pancreatic cancer: a state-of-the-art review. World J Gastroenterol 2014;20:7864-77. [Crossref] [PubMed]
- Koay EJ, Lee Y, Cristini V, Lowengrub JS, Kang Y, Lucas FAS, et al. A Visually Apparent and Quantifiable CT Imaging Feature Identifies Biophysical Subtypes of Pancreatic Ductal Adenocarcinoma. Clin Cancer Res 2018;24:5883-94. [Crossref] [PubMed]
- Health Commission Of The People's Republic Of China N. National guidelines for diagnosis and treatment of pancreatic cancer 2022 in China (English version). Chin J Cancer Res 2022;34:238-55. [Crossref] [PubMed]
- Ueno H, Ioka T, Ikeda M, Ohkawa S, Yanagimoto H, Boku N, et al. Randomized phase III study of gemcitabine plus S-1, S-1 alone, or gemcitabine alone in patients with locally advanced and metastatic pancreatic cancer in Japan and Taiwan: GEST study. J Clin Oncol 2013;31:1640-8. [Crossref] [PubMed]
- van Roessel S, Kasumova GG, Verheij J, Najarian RM, Maggino L, de Pastena M, et al. International Validation of the Eighth Edition of the American Joint Committee on Cancer (AJCC) TNM Staging System in Patients With Resected Pancreatic Cancer. JAMA Surg 2018;153:e183617.
- Matsuda Y, Ohkubo S, Nakano-Narusawa Y, Fukumura Y, Hirabayashi K, Yamaguchi H, Sahara Y, Kawanishi A, Takahashi S, Arai T, Kojima M, Mino-Kenudson M. Objective assessment of tumor regression in post-neoadjuvant therapy resections for pancreatic ductal adenocarcinoma: comparison of multiple tumor regression grading systems. Sci Rep 2020;10:18278. [Crossref] [PubMed]
- Yan B, Ma QF, Tan WF, Cai HN, Li YL, Zhou ZG, Dai X, Zhu FX, Xiong YJ, Xu M, Guo YL, Gao H, Hu JB, Wu XF. Expression of HIF-1α is a predictive marker of the efficacy of neoadjuvant chemotherapy for locally advanced cervical cancer. Oncol Lett 2020;20:841-9. [Crossref] [PubMed]
- Al-Hawary MM, Francis IR, Chari ST, Fishman EK, Hough DM, Lu DS, Macari M, Megibow AJ, Miller FH, Mortele KJ, Merchant NB, Minter RM, Tamm EP, Sahani DV, Simeone DM. Pancreatic ductal adenocarcinoma radiology reporting template: consensus statement of the Society of Abdominal Radiology and the American Pancreatic Association. Radiology 2014;270:248-60. [Crossref] [PubMed]
- Kim DW, Lee SS, Kim SO, Kim JH, Kim HJ, Byun JH, Yoo C, Kim KP, Song KB, Kim SC. Estimating Recurrence after Upfront Surgery in Patients with Resectable Pancreatic Ductal Adenocarcinoma by Using Pancreatic CT: Development and Validation of a Risk Score. Radiology 2020;296:541-51. [Crossref] [PubMed]
- Mohamed A Jr, Ayav A, Belle A, Orry X, Chevaux JB, Laurent V. Pancreatic cancer in patients with chronic calcifying pancreatitis: Computed tomography findings - a retrospective analysis of 48 patients. Eur J Radiol 2017;86:206-12. [Crossref] [PubMed]
- Youn SY, Rha SE, Jung ES, Lee IS. Pancreas ductal adenocarcinoma with cystic features on cross-sectional imaging: radiologic-pathologic correlation. Diagn Interv Radiol 2018;24:5-11. [Crossref] [PubMed]
- Shi Y, Liu Y, Gao F, Liu Y, Tao S, Li Y, Glaser KJ, Ehman RL, Guo Q. Pancreatic Stiffness Quantified with MR Elastography: Relationship to Postoperative Pancreatic Fistula after Pancreaticoenteric Anastomosis. Radiology 2018;288:476-84. [Crossref] [PubMed]
- Park HJ, Kim HJ, Kim KW, Kim SY, Choi SH, You MW, Hwang HS, Hong SM. Comparison between neuroendocrine carcinomas and well-differentiated neuroendocrine tumors of the pancreas using dynamic enhanced CT. Eur Radiol 2020;30:4772-82. [Crossref] [PubMed]
- Tirkes T, Shah ZK, Takahashi N, Grajo JR, Chang ST, Venkatesh SK, Conwell DL, Fogel EL, Park W, Topazian M, Yadav D, Dasyam AKConsortium for the Study of Chronic Pancreatitis, Diabetes, and Pancreatic Cancer. Reporting Standards for Chronic Pancreatitis by Using CT, MRI, and MR Cholangiopancreatography: The Consortium for the Study of Chronic Pancreatitis, Diabetes, and Pancreatic Cancer. Radiology 2019;290:207-15. [Crossref] [PubMed]
- Toledo RA, Jimenez C, Armaiz-Pena G, Arenillas C, Capdevila J, Dahia PLM. Hypoxia-Inducible Factor 2 Alpha (HIF2α) Inhibitors: Targeting Genetically Driven Tumor Hypoxia. Endocr Rev 2023;44:312-22. [Crossref] [PubMed]
- Cowman SJ, Koh MY. Revisiting the HIF switch in the tumor and its immune microenvironment. Trends Cancer 2022;8:28-42. [Crossref] [PubMed]
- Koong AC, Mehta VK, Le QT, Fisher GA, Terris DJ, Brown JM, Bastidas AJ, Vierra M. Pancreatic tumors show high levels of hypoxia. Int J Radiat Oncol Biol Phys 2000;48:919-22. [Crossref] [PubMed]
- Schwartz DL, Bankson JA, Lemos R Jr, Lai SY, Thittai AK, He Y, Hostetter G, Demeure MJ, Von Hoff DD, Powis G. Radiosensitization and stromal imaging response correlates for the HIF-1 inhibitor PX-478 given with or without chemotherapy in pancreatic cancer. Mol Cancer Ther 2010;9:2057-67. [Crossref] [PubMed]
- Kitada T, Seki S, Sakaguchi H, Sawada T, Hirakawa K, Wakasa K. Clinicopathological significance of hypoxia-inducible factor-1alpha expression in human pancreatic carcinoma. Histopathology 2003;43:550-5. [Crossref] [PubMed]
- Couvelard A, O'Toole D, Leek R, Turley H, Sauvanet A, Degott C, Ruszniewski P, Belghiti J, Harris AL, Gatter K, Pezzella F. Expression of hypoxia-inducible factors is correlated with the presence of a fibrotic focus and angiogenesis in pancreatic ductal adenocarcinomas. Histopathology 2005;46:668-76. [Crossref] [PubMed]
- Erstad DJ, Sojoodi M, Taylor MS, Jordan VC, Farrar CT, Axtell AL, et al. Fibrotic Response to Neoadjuvant Therapy Predicts Survival in Pancreatic Cancer and Is Measurable with Collagen-Targeted Molecular MRI. Clin Cancer Res 2020;26:5007-18. [Crossref] [PubMed]
- Koyasu S, Tsuji Y, Harada H, Nakamoto Y, Nobashi T, Kimura H, Sano K, Koizumi K, Hamaji M, Togashi K. Evaluation of Tumor-associated Stroma and Its Relationship with Tumor Hypoxia Using Dynamic Contrast-enhanced CT and (18)F Misonidazole PET in Murine Tumor Models. Radiology 2016;278:734-41. [Crossref] [PubMed]
- Korbecki J, Simińska D, Gąssowska-Dobrowolska M, Listos J, Gutowska I, Chlubek D, Baranowska-Bosiacka I. Chronic and Cycling Hypoxia: Drivers of Cancer Chronic Inflammation through HIF-1 and NF-κB Activation: A Review of the Molecular Mechanisms. Int J Mol Sci 2021;22:10701. [Crossref] [PubMed]
- Wicks EE, Semenza GL. Hypoxia-inducible factors: cancer progression and clinical translation. J Clin Invest 2022;132:e159839. [Crossref] [PubMed]
- Seo W, Kim YC, Min SJ, Lee SM. Enhancement parameters of contrast-enhanced computed tomography for pancreatic ductal adenocarcinoma: Correlation with pathologic grading. World J Gastroenterol 2020;26:4151-8. [Crossref] [PubMed]
- Hexige S, Ardito-Abraham CM, Wu Y, Wei Y, Fang Y, Han X, Li J, Zhou P, Yi Q, Maitra A, Liu JO, Tuveson DA, Lou W, Yu L. Identification of novel vascular projections with cellular trafficking abilities on the microvasculature of pancreatic ductal adenocarcinoma. J Pathol 2015;236:142-54. [Crossref] [PubMed]
- Hattori Y, Gabata T, Matsui O, Mochizuki K, Kitagawa H, Kayahara M, Ohta T, Nakanuma Y. Enhancement patterns of pancreatic adenocarcinoma on conventional dynamic multi-detector row CT: correlation with angiogenesis and fibrosis. World J Gastroenterol 2009;15:3114-21. [Crossref] [PubMed]
- Hamdy A, Ichikawa Y, Toyomasu Y, Nagata M, Nagasawa N, Nomoto Y, Sami H, Sakuma H. Perfusion CT to Assess Response to Neoadjuvant Chemotherapy and Radiation Therapy in Pancreatic Ductal Adenocarcinoma: Initial Experience. Radiology 2019;292:628-35. [Crossref] [PubMed]
- Wei X, Chen Y, Jiang X, Peng M, Liu Y, Mo Y, Ren D, Hua Y, Yu B, Zhou Y, Liao Q, Wang H, Xiang B, Zhou M, Li X, Li G, Li Y, Xiong W, Zeng Z. Mechanisms of vasculogenic mimicry in hypoxic tumor microenvironments. Mol Cancer 2021;20:7. [Crossref] [PubMed]
- Benjakul N, Prakobphol N, Tangshewinsirikul C, Dulyaphat W, Svasti J, Charngkaew K, Kangsamaksin T. Notch signaling regulates vasculogenic mimicry and promotes cell morphogenesis and the epithelial-to-mesenchymal transition in pancreatic ductal adenocarcinoma. PLoS One 2022;17:e0279001. [Crossref] [PubMed]
- Annese T, Tamma R, Ruggieri S, Ribatti D. Angiogenesis in Pancreatic Cancer: Pre-Clinical and Clinical Studies. Cancers (Basel) 2019;11:381. [Crossref] [PubMed]
- Moraes GL, Higgins CB, Ordovas KG. Delayed enhancement magnetic resonance imaging in nonischemic myocardial disease. J Thorac Imaging 2013;28:84-92; quiz 93-5. [Crossref] [PubMed]
- Perri G, Prakash L, Wang H, Bhosale P, Varadhachary GR, Wolff R, Fogelman D, Overman M, Pant S, Javle M, Koay E, Herman J, Kim M, Ikoma N, Tzeng CW, Lee JE, Katz MHG. Radiographic and Serologic Predictors of Pathologic Major Response to Preoperative Therapy for Pancreatic Cancer. Ann Surg 2021;273:806-13. [Crossref] [PubMed]
- Truty MJ, Kendrick ML, Nagorney DM, Smoot RL, Cleary SP, Graham RP, Goenka AH, Hallemeier CL, Haddock MG, Harmsen WS, Mahipal A, McWilliams RR, Halfdanarson TR, Grothey AF. Factors Predicting Response, Perioperative Outcomes, and Survival Following Total Neoadjuvant Therapy for Borderline/Locally Advanced Pancreatic Cancer. Ann Surg 2021;273:341-9. [Crossref] [PubMed]
- Heger U, Sun H, Hinz U, Klaiber U, Tanaka M, Liu B, Sachsenmaier M, Springfeld C, Michalski CW, Büchler MW, Hackert T. Induction chemotherapy in pancreatic cancer: CA 19-9 may predict resectability and survival. HPB (Oxford) 2020;22:224-32. [Crossref] [PubMed]