Optimized contrast enhancement and homogeneity in aortic CT angiography: bolus tracking with personalized post-trigger delay
Introduction
The prevalence of aortic disease increases annually, with patients in critical condition experiencing high rates of death and disability (1). Computed tomography angiography (CTA) of the aorta is the preferred modality for diagnosing aortic diseases due to its high diagnostic sensitivity and specificity (2,3).
The clinical manifestations of aortic diseases exhibit considerable heterogeneity, with acute aortic conditions demonstrating a pronounced early mortality risk, yet some aortic diseases may be less symptomatic or asymptomatic (4). Therefore, accurate evaluation of the aorta assumes paramount importance in guiding therapeutic interventions and prognostic outcomes for patients with aortic diseases, underscoring the need for high-quality CTA images.
Attaining high-quality CTA images requires prominent arterial enhancement. In CTA imaging, contrast enhancement is influenced by several factors (5-7), including contrast injection rate, injection duration, concentration of injected iodine, technical parameters such as tube potential, and patient factors, especially cardiac output and peripheral resistance. Discrepancies in patient time–concentration curves under the same injection and acquisition protocol persist due to variations in the timing of peak aortic enhancement influenced by factors such as left ventricular ejection fraction, coronary artery disease, cardiac cycle duration, and other variables (6,8). Therefore, precise timing of image acquisition during the arterial phase is a pivotal requirement.
Bolus tracking, commonly employed for determining scan timing, offers several advantages including reduced contrast agent dosage, decreased radiation exposure, and expedited workflow efficiency (9). Monitoring computed tomography (CT) attenuation across a predetermined area until it exceeds the trigger threshold, followed by image acquisition after a fixed post-trigger delay (PTD). Although it represents an improvement over the previous fixed timing without monitoring, there is still significant potential for enhancement in personalized applications. This is due to the time difference between reaching the threshold and reaching the peak arterial enhancement among patients. Particularly in patients with coexisting cardiovascular disorders and aneurysms, the slow blood flow speed in the aorta may cause premature initiation of image acquisition due to a fixed delay, leading to completion before the peak of arterial enhancement.
In the process of exploring the personalization of delay time, previous retrospective studies have attempted to administer PTD based on specific formulas for different indications (10-12). Some researchers have used the double region of interest (ROI) timing bolus or femoral artery test injection method to calculate the scan timing (13,14), which could introduce variation from different operators or complicate the workflow. Accordingly, in addition to achieving personalization, the PTD should also be automatic because the patient’s cardiac output information is generally not available prior to image acquisition (15).
To tackle these challenges, we used an innovative bolus tracking algorithm that employs a convolution-based algorithm to automatically derive a personalized PTD from the contrast injection and monitoring measurements information. The aim of this study was to demonstrate the feasibility of achieving optimized enhancement and contrast homogeneity with personalized PTD in aortic CTA by comparing image quality between the fixed PTD group and the personalized PTD group. We present this article in accordance with the TREND reporting checklist (available at https://qims.amegroups.com/article/view/10.21037/qims-24-624/rc).
Methods
Ethics approval
The study was conducted in accordance with the Declaration of Helsinki (as revised in 2013). This prospective study was approved by the Scientific Research and Clinical Trial Ethics Committee of the First Affiliated Hospital of Zhengzhou University (No. 2021-KY-0976), and written informed consent was provided by all the patients.
Study population
This prospective, single-center study, conducted from May 2023 to February 2024, included 148 participants aged 18 years or older who required aortic CTA in our department. Participants with the following characteristics were excluded: prior allergic reactions to iodinated contrast media (n=1), chronic kidney disease (estimated glomerular filtration rate less than 30 mL/min/1.73 m2; n=2), pregnancy (n=1), occlusion of the infrarenal aorta (aortoiliac occlusive disease; n=1), less than 4 CT numbers during the monitoring scan (n=2), and bolus tracking failure (n=1). Finally, 140 consecutive study participants underwent the aortic CTA (Figure 1).
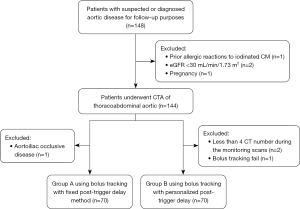
The first 70 participants were assigned to the control group [Group A, 13 females, mean ± standard deviation (SD) age 58±11 years] using a fixed PTD, whereas the remaining 70 participants were assigned to the experimental group (Group B, 18 females, mean ± SD age 59±12 years) with a personalized PTD. Table 1 outlines patient details and indications in both groups.
Table 1
Variables | Group A (n=70) | Group B (n=70) | P value |
---|---|---|---|
Age (years) | 58±11 | 59±12 | 0.645 |
Sex | 0.309 | ||
Female | 13 [19] | 18 [26] | |
Male | 57 [81] | 52 [74] | |
Height (cm) | 169.69±7.01 | 168.30±6.92 | 0.241 |
Weight (kg) | 72.69±13.82 | 70.82±11.40 | 0.383 |
BMI (kg/m2) | 25.18±4.27 | 24.92±3.28 | 0.679 |
Comorbidities | |||
Diabetes | 10 [14] | 11 [16] | 0.813 |
Hypertension | 41 [59] | 38 [54] | 0.609 |
Dyslipidemia | 26 [37] | 30 [43] | 0.534 |
Indications for CTA | 0.985 | ||
Untreated aortic dissection | 5 [7] | 4 [6] | |
Untreated aneurysm | 12 [17] | 11 [16] | |
Follow-up after endovascular repair of thoracic aorta | 9 [13] | 12 [17] | |
Follow-up after endovascular repair of abdominal aorta and iliac arteries | 8 [11] | 8 [11] | |
Pain of uncertain etiology | 10 [14] | 12 [17] | |
Aortic ulcer | 8 [11] | 5 [7] | |
Aortic regurgitation | 5 [7] | 5 [7] | |
Coronary artery disease | 13 [19] | 13 [19] | |
Delay time (s) | 6 | 11.2±1.5 | <0.001 |
Tube voltage (kV) | 90 | 90 | – |
Tube current (mAs) | 205.37±67.74 | 207.61±48.79 | 0.822 |
CTDIvol (mGy) | 5.79±1.68 | 5.89±1.24 | 0.698 |
DLP (mGy∙cm) | 433.55±139.82 | 425.09±98.89 | 0.680 |
ED (mSv) | 6.29±2.03 | 6.16±1.43 | 0.680 |
Data are presented as mean ± SD, n [%], or mean. Group A: the control group using the fixed PTD; Group B: the experimental group using the personalized PTD. BMI, body mass index; CTA, computed tomography angiography; CTDIvol, volumetric CT dose index; CT, computed tomography; DLP, dose length product; ED, effective dose; SD, standard deviation; PTD, post-trigger delay.
CT scanning and contrast media injection protocol
Scans were performed using the third-generation 192-section dual-source CT scanner (SOMATOM Force; Siemens Healthineers, Erlangen, Germany) in the single-source mode. Image acquisition parameters remained the same in the two groups: tube voltage of 90 kVp; pitch of 1.2; collimation of 192 mm × 0.6 mm; gantry rotation time of 500 ms; and automated attenuation-based tube current (CareDose4D; Siemens Healthineers). All images were reconstructed with a slice thickness of 1.0 mm and an increment of 1.0 mm, utilizing a universally adapted field of view (FOV) size. The reconstruction was performed using advanced modeled iterative reconstruction (ADMIRE) with a strength level of 3 and a medium-smooth soft tissue kernel (Bv40).
The contrast media injection protocol was consistent in both groups: 18 mL of 0.9% saline solution was injected through the anterior cubital vein at a flow rate of 5 mL/s to ensure smooth contrast flow. Subsequently, 60 mL of preheated non-ionic iodinated contrast media (Iohexol, 350 mgI/mL) and 50 mL of saline were sequentially injected at a flow rate of 3 mL/s, because total iodine of 21 g and an injection flow rate of 3 mL/s produces excellent angiography at 90 kVp (16).
Fixed and personalized PTD in bolus tracking
The bolus tracking technique was used to initiate the scan. A circular ROI was positioned at the level of the left atrium on the descending aorta to monitor contrast enhancement. The attenuation of the trigger threshold was 100 Hounsfield units (HU) at 100 kVp. In the control group, image acquisition began with a fixed 6-second delay after reaching the threshold. In the experimental group, personalized PTD was determined by the new bolus tracking algorithm (Custom Pack, Somaris 7.VA50 SP2, version 2; Siemens Healthineers). This algorithm predicts the local contrast over time by extrapolating measured contrast enhancement values. For prediction, contrast dynamics are modeled by convoluting the applied contrast media injection protocol (in gI/s) and the patient-specific arterial impulse response function (in HU). IP depends on the iodine concentration, injection rate and injection duration, whereas arterial input function (AIF) is derived by performing least-squares online fitting using a minimum of 4 CT values obtained during the monitoring scans to match the population-averaged parameterized arterial circulation curve in the enhancement curve database. The mid-scan time was placed at the peak of enhancement. The time between the last bolus tracking enhancement and the start of scan serves as the ideal PTD (17,18) (Figure 2).
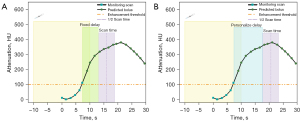
Image analysis
Objective image quality
An experienced cardiovascular radiologist with 11 years of expertise (P.L.) evaluated the objective image quality using the workstation (Syngo.via, VB40; Siemens Healthineers). The radiologist, blinded to patient allocation, scan protocol, and contrast media protocol, manually positioned circular ROIs on the axis-corrected axial reformations based on automatically defined vessel centerlines. ROIs (19) were placed at various anatomical levels to measure the CT attenuation of the vessel (HUvessel). These levels included the pulmonary artery, ascending and descending aorta at the level of the pulmonary trunk, abdominal aorta, right common iliac artery, and the branches of the abdominal aorta (the celiac trunk, superior and inferior mesenteric arteries, left and right renal arteries). Additionally, the attenuation of the psoas muscle at the lower pole of the right kidney was measured as the background signal (HUmuscle), and the SD of the attenuation was defined as the image noise (N).
ROIs were maximized based on the internal diameter of the vessels, excluding the vessel wall, aortic stents, calcified plaque, thrombus, and streak artifacts (20). ROIs in the psoas muscle were carefully drawn to avoid fatty streaks and vessels within the muscle. Contrast-to-noise ratio (CNR) was calculated as follows:
Enhancement level and contrast homogeneity
Contrast enhancement level was defined as low (at least 1 CT attenuation lower than 350 HU in the descending aorta, abdominal aorta, and right common iliac artery), optimal (all three aortic CT attenuations between 350 and 600 HU), and high (at least one CT attenuation greater than 600 HU in the above three ROIs) (16,21).
Contrast enhancement homogeneity is classified as poor when the difference in CT attenuation between the ascending aorta and the right common iliac artery is >80 HU, and optimal when the difference is ≤80 HU (17,21).
Evaluation of the abdominal aortic branches: to further evaluate the new algorithm’s potential in patients with untreated aortic dissection or aneurysm, the enhancement level of the abdominal aortic branches was assessed (22). In Group A, there were five patients with untreated aortic dissection, 12 patients with untreated aneurysms, and seven patients with residual disease after endovascular repair, for a total of 120 branch vessels measured. In Group B, there were four patients with untreated aortic dissection, 11 patients with untreated aneurysms, and seven patients with residual disease after endovascular repair, with a total of 110 branch vessels measured.
Subjective image quality
The subjective image quality for each patient was evaluated by two cardiovascular CT imaging observers (P.L. and J.L.), with 11 and 23 years of experience, respectively. They were blinded to the clinical indication, experimental allocation, and imaging results. The maximum intensity projection (MIP) images of multiplanar reformation (MPR) and curved planar reformation (CPR) were evaluated. The study utilized a 5-point Likert scale to assess subjective image quality scores (17,23): 5, excellent (aorta and iliac arteries with excellent enhancement and homogeneous contrast media distribution, clear delineation of the vessel walls and no artifact); 4, good; 3, moderate; 2, poor; 1, non-diagnostic (aorta and iliac arteries with unacceptable enhancement, and no proximal or distal contrast enhancement caused significant inhomogeneity, poor delineation of vessel walls, and prominent artifact). Data from five patients outside of this study were randomly selected for practice testing based on the above criteria.
Radiation dose estimates
The volumetric CT dose index (CTDIvol) and the dose length product (DLP) were recorded. The effective dose (ED) was estimated by multiplying the DLP with a conversion factor of 0.0145 mSv/mGy·cm, which is the average of the thoracic (0.014 mSv/mGy·cm) and abdominal (0.015 mSv/mGy·cm) coefficients (16).
Statistical analysis
The normality of continuous data was tested using the Shapiro-Wilk test. Quantitative data that were normally distributed were presented as mean ± SD, whereas data with non-normal distributions were presented as medians and interquartile ranges (IQRs). Categorical variables were presented as percentages and frequencies. The Student’s t-test or Mann-Whitney U test was used to determine quantitative data, whereas the Chi-square test was used to compare categorical variables between the two groups. For image quality assessment, the Student’s t-test was employed to analyze the mean attenuation, the Mann-Whitney U test was used to assess CNR, and the Chi-square test was utilized to evaluate enhancement level, contrast homogeneity, and subjective scores in both groups. The agreement level between the two assessments of subjective image quality was evaluated using Cohen’s kappa.
All analyses were performed with the software SPSS 26.0 (IBM Corp., Armonk, NY, USA). A P value <0.05 indicated a statistically significant difference.
Results
Study participants
Characteristics and radiological parameters of the study participants in both groups are shown in Table 1. No significant differences were observed between the two groups in terms of age (P=0.645), sex (P=0.309), height (P=0.241), weight (P=0.383), body mass index (BMI; P=0.679), CTDIvol (P=0.698), DLP (P=0.680), or ED (P=0.680).
Determination of PTD using bolus tracking
In Group A, the PTD was fixed at 6 seconds, whereas for Group B, the algorithm automatically calculated personalized PTD (mean ± SD, 11.2±1.5 seconds) ranging from 7.8 to 14.1 seconds.
Objective image quality
The mean attenuation and CNR of the aortoiliac artery were higher in Group B compared with Group A (417.55±71.55 vs. 345.71±60.41 HU, 16 vs. 13, both P<0.001, respectively), and the largest difference between groups was observed in the common iliac artery [110.97 HU; 95% confidence interval (CI): 87.60–134.34 HU] (Table 2). The mean attenuation of the artery was significantly higher in group B than in group A, regardless of the branch and location of the measurements. Also, Group B demonstrated improved CNRs in most arteries (Figure 3).
Table 2
Parameters | Group A (n=70) | Group B (n=70) | Difference† | 95% CI† | P value |
---|---|---|---|---|---|
Aortoiliac attenuation (HU) | 345.71±60.41 | 417.55±71.55 | 71.84 | 49.70, 93.97 | <0.001 |
Ascending aorta (HU) | 381.77±73.17 | 415.66±78.71 | 33.89 | 8.49, 59.28 | 0.009 |
Descending aorta (HU) | 350.40±69.47 | 421.81±70.81 | 71.41 | 47.97, 94.86 | <0.001 |
Abdominal aorta (HU) | 341.06±71.54 | 412.13±72.08 | 71.07 | 47.07, 95.07 | <0.001 |
Right common iliac artery (HU) | 309.63±64.44 | 420.60±75.00 | 110.97 | 87.60, 134.34 | <0.001 |
Psoas muscle (HU) | 50.60±10.30 | 51.70±18.55 | 1.10 | −3.91, 6.11 | 0.665 |
CNR‡ | 13 [12, 15] | 16 [14, 20] | – | – | <0.001 |
Data are presented as mean ± SD or median [IQR], unless otherwise specified. †, indicates differences in mean attenuation values (Group B − Group A) and CIs of differences at each location; ‡, CNR of aortoiliac artery was calculated as the difference of mean aortoiliac attenuation minus right psoas muscle attenuation divided by SD of attenuation measured in the right psoas muscle. Group A: the control group using the fixed PTD; Group B: the experimental group using the personalized PTD. CI, confidence interval; HU, Hounsfield units; CNR, contrast-to-noise ratio; SD, standard deviation; IQR, interquartile range; PTD, post-trigger delay.
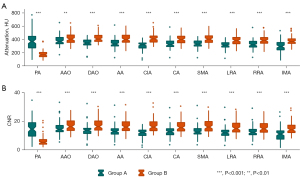
Enhancement levels and contrast homogeneity
Group B exhibited a significantly higher percentage of optimal enhancement levels and contrast homogeneity compared to Group A (78.6% vs. 37.1%, 94.3% vs. 64.3%, both P<0.001, respectively; see Table 3).
Table 3
Category | All participates | Vessels branches† | |||||
---|---|---|---|---|---|---|---|
Group A (n=70) | Group B (n=70) | P value | Group A (n=120) | Group B (n=110) | P value | ||
Enhancement level | <0.001 | <0.001 | |||||
Low | 43 (61.4) | 13 (18.6) | 92 (76.7) | 28 (25.5) | |||
Optimal | 26 (37.1) | 55 (78.6) | 28 (23.3) | 82 (74.5) | |||
High | 1 (1.4) | 2 (2.9) | |||||
Homogeneity | <0.001 | ||||||
Fair | 25 (35.7) | 4 (5.7) | |||||
Excellent | 45 (64.3) | 66 (94.3) |
Data are presented as n (%). †, vessels branch of patients with untreated aortic disease or residual disease. Group A: the control group using the fixed PTD; Group B: the experimental group using the personalized PTD. PTD, post-trigger delay.
With personalized PTD, 75.7% (53/70) of participants showed optimal enhancement levels and contrast homogeneity, whereas using fixed delay, only 20.0% (14/70) achieved both. Specifically, 17.1% (12/70) in Group A had optimal enhancement levels but poor contrast homogeneity, whereas 44.3% (31/70) had optimal contrast homogeneity but low enhancement levels (Tables S1,S2).
Evaluation of abdominal aortic branches: a total of 230 vessel branches were evaluated (Table 3). Group B exhibited a significantly higher percentage of holding an attenuation of over 350 HU in branch vessels of the abdominal aorta compared to Group A (74.5% vs. 23.3%, P<0.001).
Subjective image quality
Figure 4 displays the scores of subjective image quality in the two groups. Both observers concurred that Group B had superior overall image quality (both P<0.001), which they both rated excellent or good in 50 of 70 (71.4%) and 8 of 70 participants (11.4%) (Table S3). Good coherence was found between the two observers, with subjective quality scores reaching a Cohen’s kappa of 0.868 (95 % CI: 0.806–0.930). Hence, the rating of observer 1 was used for the final outcomes (20). The percentage of scores greater than or equal to 4 was 91.4% for Group B and 68.6% for Group A. Image examples of the two groups are shown in Figure 5.
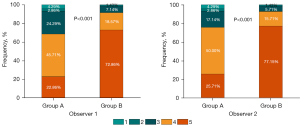
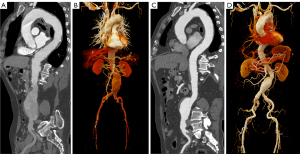
Discussion
In the present study, we compared aortic CTA images obtained using a personalized PTD with those using a fixed delay. Our results showed that the personalized PTD can achieve significantly higher vessel attenuation and improved contrast homogeneity under the same injection and acquisition protocol, compared with the fixed delay.
The development of CT technology has led to a gradual reduction in scanning time, driving the trend towards using less contrast media. With the reduction in the amount of contrast media, the precise determination of scanning timing becomes more critical for achieving sufficient contrast opacification. For the precise determination of scan timing, three key factors need to be considered: (I) injection duration; (II) contrast media arrival time; and (III) scan duration (5). Under the same contrast media injection protocol and other scan parameters, attention should be paid to the contrast media arrival time, which is closely related to the individual patient’s cycle time and is influenced by individual factors such as cardiac output and cardiovascular circulation. In our study, the new bolus tracking algorithm was used to achieve this by precisely synchronizing the scanning duration with the contrast administration. The personalized PTD ranged from 7.8 to 14.1 seconds under the same injection and collection protocol, indicating that the varied time-concentration curves were caused by patient-specific cardiovascular parameters such as blood circulation time and cardiac output; therefore, using the same fixed PTD for all patients was not ideal.
Previous studies have proposed the anticipation of contrast enhancement before diagnostic CTA scans to provide patient-specific scan timing for each patient. For instance, the optical test bolus technique by Eisa et al. (24) provided adequate estimates of the bolus arrival time, but was limited in specialized optical sensors, dye contrast media, and lacked clinical validation. In contrast, this new bolus tracking technique with personalized PTD can be seamlessly integrated into scanning protocols to enhance the intelligence of the CTA imaging process. It adapts real-time patient data to an online database of the population-averaged parameterized arterial circulation curve, generates the patient-specific arterial impulse response, incorporates contrast media injection information, uses the convolution algorithm to build a predictive model, and automatically provides a personalized PTD, minimizing the impact of individual differences. According to Bae et al. (5), a higher enhancement can be achieved with faster multidetector CT for a given iodine dose due to improved contrast medium utilization from optimized scan timing. Several studies (15,17,25,26) investigated the feasibility of improving image quality with the personalized PTD bolus tracking technology in contrast-enhanced CT of abdominal parenchymal organs and CTA. Our study applied the same technique to CTA of the aorta, and validated the benefits of providing homogeneous and enhanced images.
Related to the precise scan timing, homogeneous and high-contrast enhancement are also essential for ensuring proper diagnosis in long z-axis coverage of CTA scans, such as aortic CTA. In addition to determining aneurysm size, angulation, and tortuosity, as well as the extension of aortic dissection and involvement of branch arteries, the presence of distal artery stenoses also needs to be assessed in patients with bifurcation endovascular stents or symptoms such as lower limb pain and absent femoral pulse. Discrepancies in enhancement level may affect the ability to address all clinical questions, leading to artificial defects or perceived narrowing (27). Previously, researchers have typically prolonged the state plateau phase, such as using an exponentially decelerated or multibolus contrast injection protocol (22,28), rather than precisely capturing the scan timing. The personalized PTD optimizes the scan timing by allowing sufficient delay before image acquisition and ensuring that the CT scan is focused on the plateau phase (a broad peak) of the time-enhancement curve. For instance, the turbulent blood flow in the aneurysm changes the hemodynamic situation and reduces distal blood flow and the bolus transit time is expected to increase (29). Thus, the use of a personalized PTD seems reasonable in these patients to provide sufficient contrast enhancement of the aneurysms and distal aortic branches, as a fixed PTD may lead to suboptimal visualization (30). The results of this study show that, using a personalized PTD, Group B showed significantly higher CT values and contrast homogeneity compared to Group A. Furthermore, patients with aortic dissection or aneurysm exhibited enhanced enhancement in abdominal aortic branches. This information was valuable for diagnosing and adequately opacifying the lesion, which is important for predicting late aortic events (31-33).
Additionally, reducing the contrast media volume has become an increasingly important concern for avoiding additional risks, such as post-contrast acute kidney injury. The implementation of personalized PTD in Group B maximized the use of contrast media while minimizing redundancy or waste, resulting in higher CT attenuation than the fixed PTD under the same injection protocol. Given that the peak enhancement of the aorta is directly proportional to the contrast media volume, we believe that utilizing the personalized PTD algorithm has the potential to further reduce the volume of contrast media while maintaining image quality, and further work will be conducted in the future.
Conclusions
Some limitations existed in this study. First of all, the sample size was limited. Second, the tube voltage and contrast protocol were fixed and not adjusted for body weight. However, these steps were taken to minimize other potential confounding factors affecting vessel attenuation and contrast homogeneity, to evaluate the effect of PTD more clearly. Finally, this study focused on the feasibility of using the personalized PTD algorithm to optimize image quality in the broad area of the thoracic and abdominal aorta rather than determining the optimal PTD required for a specific disease type. Further research is needed on whether there is a relationship between different aneurysm sizes or extension of aortic dissection and delay time, and whether different scan timing parameters are required for different diseases, such as hepatic hemangioma. In conclusion, the personalized PTD in bolus tracking can provide accurate scan timing, produce consistent, homogeneous vascular enhancement, and achieve enhanced image quality in aortic CTA scans. Comparison with the conventional fixed PTD highlights the superiority of the personalized PTD.
Acknowledgments
Funding: This work was supported by
Footnote
Reporting Checklist: The authors have completed the TREND reporting checklist. Available at https://qims.amegroups.com/article/view/10.21037/qims-24-624/rc
Conflicts of Interest: All authors have completed the ICMJE uniform disclosure form (available at https://qims.amegroups.com/article/view/10.21037/qims-24-624/coif). L.L. is employed by Siemens Healthineers and did not have control over the data at any point during the study. The other authors have no conflicts of interest to declare.
Ethical Statement: The authors are accountable for all aspects of the work in ensuring that questions related to the accuracy or integrity of any part of the work are appropriately investigated and resolved. The study was conducted in accordance with the Declaration of Helsinki (as revised in 2013). The study was approved by Scientific Research and Clinical Trial Ethics Committee of the First Affiliated Hospital of Zhengzhou University (No. 2021-KY-0976) and informed consent was provided by all participants.
Open Access Statement: This is an Open Access article distributed in accordance with the Creative Commons Attribution-NonCommercial-NoDerivs 4.0 International License (CC BY-NC-ND 4.0), which permits the non-commercial replication and distribution of the article with the strict proviso that no changes or edits are made and the original work is properly cited (including links to both the formal publication through the relevant DOI and the license). See: https://creativecommons.org/licenses/by-nc-nd/4.0/.
References
- Bossone E, Eagle KA. Epidemiology and management of aortic disease: aortic aneurysms and acute aortic syndromes. Nat Rev Cardiol 2021;18:331-48. [Crossref] [PubMed]
- Rubin GD, Leipsic J, Joseph Schoepf U, Fleischmann D, Napel S. CT angiography after 20 years: a transformation in cardiovascular disease characterization continues to advance. Radiology 2014;271:633-52. [Crossref] [PubMed]
- Fleischmann D, Mitchell RS, Miller DC. Acute aortic syndromes: new insights from electrocardiographically gated computed tomography. Semin Thorac Cardiovasc Surg 2008;20:340-7. [Crossref] [PubMed]
- Tsai TT, Nienaber CA, Eagle KA. Acute aortic syndromes. Circulation 2005;112:3802-13. [Crossref] [PubMed]
- Bae KT. Intravenous contrast medium administration and scan timing at CT: considerations and approaches. Radiology 2010;256:32-61. [Crossref] [PubMed]
- Sakai S, Yabuuchi H, Chishaki A, Okafuji T, Matsuo Y, Kamitani T, Setoguchi T, Honda H. Effect of cardiac function on aortic peak time and peak enhancement during coronary CT angiography. Eur J Radiol 2010;75:173-7. [Crossref] [PubMed]
- Faggioni L, Gabelloni M. Iodine Concentration and Optimization in Computed Tomography Angiography: Current Issues. Invest Radiol 2016;51:816-22. [Crossref] [PubMed]
- Manghat NE, Morgan-Hughes GJ, Shaw SR, Marshall AJ, Roobottom CA. Impaired left ventricular function has a detrimental effect on image quality in multi-detector row CT coronary angiography. Clin Radiol 2008;63:415-23. [Crossref] [PubMed]
- Beeres M, Loch M, Schulz B, Kerl M, Al-Butmeh F, Bodelle B, Herrmann E, Gruber-Rouh T, Lee C, Jacobi V, Vogl TJ, Bauer RW. Bolus timing in high-pitch CT angiography of the aorta. Eur J Radiol 2013;82:1028-33. [Crossref] [PubMed]
- Schneider JG, Wang ZJ, Wang W, Yee J, Fu Y, Yeh BM. Patient-tailored scan delay for multiphase liver CT: improved scan quality and lesion conspicuity with a novel timing bolus method. AJR Am J Roentgenol 2014;202:318-23. [Crossref] [PubMed]
- Camera L, Dell'Aversano-Orabona G, Gambardella M, Riccitiello F, Galatola R, Liuzzi R, Longobardi M, Danzi R, Ponsiglione A, Stanzione A, Maurea S, Brunetti A. Tailored versus fixed scan delay in contrast-enhanced abdominal multi-detector CT: An intra-patient comparison of image quality. Eur J Radiol 2021;143:109914. [Crossref] [PubMed]
- Lehmkuhl L, Andres C, Lücke C, Hoffmann J, Foldyna B, Grothoff M, Nitzsche S, Schmidt A, Ulrich M, Scheinert D, Gutberlet M. Dynamic CT angiography after abdominal aortic endovascular aneurysm repair: influence of enhancement patterns and optimal bolus timing on endoleak detection. Radiology 2013;268:890-9. [Crossref] [PubMed]
- Hoshino T, Ichikawa K, Hara T, Terakawa S, Hosomi K, Nishimura K, Takayama K. Optimization of scan timing for aortic computed tomographic angiography using the test bolus injection technique. Acta Radiol 2016;57:829-36. [Crossref] [PubMed]
- Tomizawa N, Ito S, Nakao T, Arakawa H, Yamamoto K, Inoh S, Nojo T, Nakamura S. Aortic CT angiography using the double region of interest timing bolus technique: feasibility of 80 kVp scanning in lean patients. Int J Cardiovasc Imaging 2019;35:2113-21. [Crossref] [PubMed]
- Yu J, Lin S, Lu H, Wang R, Liu J, Gutjahr R, Gao J. Optimize scan timing in abdominal multiphase CT: Bolus tracking with an individualized post-trigger delay. Eur J Radiol 2022;148:110139. [Crossref] [PubMed]
- Higashigaito K, Schmid T, Puippe G, Morsbach F, Lachat M, Seifert B, Pfammatter T, Alkadhi H, Husarik DB. CT Angiography of the Aorta: Prospective Evaluation of Individualized Low-Volume Contrast Media Protocols. Radiology 2016;280:960-8. [Crossref] [PubMed]
- Hinzpeter R, Eberhard M, Gutjahr R, Reeve K, Pfammatter T, Lachat M, Schmidt B, Flohr TG, Kolb B, Alkadhi H. CT Angiography of the Aorta: Contrast Timing by Using a Fixed versus a Patient-specific Trigger Delay. Radiology 2019;291:531-8. [Crossref] [PubMed]
- Korporaal JG, Bischoff B, Arnoldi E, Sommer WH, Flohr TG, Schmidt B. Evaluation of A New Bolus Tracking-Based Algorithm for Predicting A Patient-Specific Time of Arterial Peak Enhancement in Computed Tomography Angiography. Invest Radiol 2015;50:531-8. [Crossref] [PubMed]
- Komber HMEI, Neumann S, Paull J, Andrade MG, Lyen SM, Manghat NE, Hamilton MCK. A quality-improvement project to enhance systemic arterial contrast opacification in CT for trans-catheter aortic valve implantation. Clin Radiol 2022;77:e697-704. [Crossref] [PubMed]
- Higashigaito K, Mergen V, Eberhard M, Jungblut L, Hebeisen M, Rätzer S, Zanini B, Kobe A, Martini K, Euler A, Alkadhi H. CT Angiography of the Aorta Using Photon-counting Detector CT with Reduced Contrast Media Volume. Radiol Cardiothorac Imaging 2023;5:e220140. [Crossref] [PubMed]
- Chen PA, Huang EP, Chen KT, Chen YC, Huang YL, Chuo CC, Wu FZ, Wu MT. Comparison of four contrast medium delivery protocols in low-iodine and low-radiation dose CT angiography of the aorta. Clin Radiol 2020;75:797.e9-797.e19. [Crossref] [PubMed]
- Obmann MM, Gehweiler J, Schindera ST, Janetzki J, Boll DT, Benz MR. Clinical evaluation of a novel multibolus contrast agent injection protocol for thoraco-abdominal CT angiography: Assessment of homogeneity of arterial contrast enhancement. Eur J Radiol 2020;126:108957. [Crossref] [PubMed]
- Fink MA, Stoll S, Melzig C, Steuwe A, Partovi S, Böckler D, Kauczor HU, Rengier F. Prospective Study of Low-Radiation and Low-Iodine Dose Aortic CT Angiography in Obese and Non-Obese Patients: Image Quality and Impact of Patient Characteristics. Diagnostics (Basel) 2022.
- Eisa F, Brauweiler R, Peetz A, Hupfer M, Nowak T, Kalender WA. Optical tracking of contrast medium bolus to optimize bolus shape and timing in dynamic computed tomography. Phys Med Biol 2012;57:N173-82.
- Yuan D, Li L, Zhang Y, Qi K, Zhang M, Zhang W, Lyu P, Zhang Y, Gao J, Liu J. Image quality improvement in head and neck CT angiography: Individualized post-trigger delay versus fixed delay. Eur J Radiol 2023;168:111142. [Crossref] [PubMed]
- Yuan D, Wang Y, Lin S, Gutjahr R, Lyu P, Zhang Y, Gao J, Liu J. Patient-specific post-trigger delay in coronary CT angiography: A prospective study comparing with fixed delay. Eur J Radiol 2023;163:110813. [Crossref] [PubMed]
- Liu Y, Hopper KD, Mauger DT, Addis KA. CT angiographic measurement of the carotid artery: optimizing visualization by manipulating window and level settings and contrast material attenuation. Radiology 2000;217:494-500. [Crossref] [PubMed]
- Saade C, Mayat A, El-Merhi F. Exponentially Decelerated Contrast Media Injection Rate Combined With a Novel Patient-Specific Contrast Formula Reduces Contrast Volume Administration and Radiation Dose During Computed Tomography Pulmonary Angiography. J Comput Assist Tomogr 2016;40:370-4. [Crossref] [PubMed]
- Biasetti J, Gasser TC, Auer M, Hedin U, Labruto F. Hemodynamics of the normal aorta compared to fusiform and saccular abdominal aortic aneurysms with emphasis on a potential thrombus formation mechanism. Ann Biomed Eng 2010;38:380-90. [Crossref] [PubMed]
- Werncke T, von Falck C, Wittmann M, Elgeti T, Wacker FK, Meyer BC. Optimal table feed in run-off CT angiography in patients with abdominal aortic aneurysms. Eur Radiol 2013;23:2482-91. [Crossref] [PubMed]
- Hsu HL, Chiu YN, Chen TW, Huang CY, Shih CC, Hsu CP. Flow density of computed tomography aortography for predicting early unfavorable aortic remodeling after TEVAR in type IIIb aortic dissection. Int J Cardiol 2021;332:41-7. [Crossref] [PubMed]
- Nishida H, Nakamura R, Tamaki R, Abe K. Inconsistent False Lumen Enhancement Predicts Late Aortic Events After Hemiarch Replacement in Acute Type A Aortic Dissection. Am J Cardiol 2024;221:77-83. [Crossref] [PubMed]
- Dou BY, La Combe de Villers VG, Boukhatem A, Noel-Lamy M, Belzile F, Bui TB, Gahide G. Use of the Endoleak-to-Aortic Density Ratio to Distinguish Direct Endoleaks from Indirect Endoleaks after Endovascular Aortic Aneurysm Repair. J Vasc Interv Radiol 2023;34:1698-1706.e1. [Crossref] [PubMed]