Brain surface area and function alterations are correlated with cognition in patients with end-stage renal disease
Introduction
Chronic kidney disease (CKD) has a profound effect on the overall well-being of global populations, and is a substantial contributor to mortality (1). A considerable proportion of patients with CKD may exhibit a spectrum of cognitive dysfunction, ranging from mild cognitive impairment (MCI) to dementia (2,3). These impairments encompass various cognitive domains, including memory (4), language (5), attention (6), visuospatial skills, and executive functions (7). End-stage renal disease (ESRD), which is defined as a glomerular filtration rate <15 mL/min/1.73 m2, is the final stage of CKD progression. In this stage, patients’ renal function is insufficient to support their long-term survival without kidney transplantation or dialysis (8). However, once dialysis is initiated, patients are at an increased risk of a variety of adverse outcomes, including stroke, depression, and high mortality in the first year of dialysis (9,10). Moreover, hemodynamic disturbances caused by hemodialysis may accelerate the progression of cognitive impairment in ESRD (11). Therefore, imaging and biomarkers related to cognitive impairment should be screened, and the potential neuropathological mechanisms of cognitive decline in ESRD patients should be evaluated.
Vascular factors associated with ESRD and neurotoxic substances resulting from uremia may have an effect on cognitive dysfunction (12,13). Kidney dysfunction in ESRD often leads to the retention of various molecular mediators associated with brain damage, which can subsequently affect the brain through direct or indirect pathways (14). Due to the reduced function of the blood-brain barrier (BBB) in advanced CKD (15), certain toxins associated with uremia can readily penetrate the BBB, leading to detrimental effects on endothelial cells and blood vessels.
Dialysis, including hemodialysis and peritoneal dialysis, is a common treatment for ESRD, Dialysis is an efficient method for removing water-soluble toxins to prevent uremic encephalopathy; however, its efficacy in eliminating protein-bound or medium-sized toxins is limited (16), and these toxins are the main cause of MCI. During hemodialysis, patients are exposed to continuous and repeated fluid volume changes, which may lead to acute and chronic hemodynamic stress (17). Additionally, the accumulation of sodium and fluid during the interdialytic period may result in hypertension and cerebrovascular complications (18). Additionally, the dialysis itself may further exacerbate cognitive decline.
Multimodal magnetic resonance imaging (MRI) has been widely used to assess brain structural and functional changes, and plays a key role in the early diagnosis of cognitive impairment in ESRD patients. Structural MRI studies have shown that ESRD patients have decreased cortical gray-matter volume (GMV), which is closely related to cognitive impairment (19,20). In addition, resting-state functional MRI (rs-fMRI) revealed that ESRD patients, with or without dialysis, showed reduced whole-brain spontaneous activity dominated by the default mode network (DMN), which was positively correlated with the Montreal Cognitive Assessment (MoCA) score, providing deeper insights into the neural mechanisms of cognitive impairment in CKD (21,22). However, most studies have focused on GMV and whole-brain functional changes in ESRD with hemodialysis (ESRD-HD) patients; thus, knowledge about the cortical structural and functional changes of ESRD patients is limited. Therefore, there is an urgent need to understand the cortical structural and functional changes of non-dialysis and maintenance dialysis ESRD patients, and their relationship with cognitive decline. Clarifying the imaging features of abnormal brain regions associated with cognitive impairment in early ESRD patients will aid in the early identification of cognitive impairment and the development of intervention strategies.
Therefore, this study aimed to examine the altered structure and function in ESRD-HD and ESRD without hemodialysis (ESRD-ND) patients based on a brain cortical structural and functional approach, and to assess the following hypotheses: (I) patients with ESRD experience structural and functional alterations; and (II) alterations of the brain surface area and function in patients with ESRD are correlated with the MoCA score. We present this article in accordance with the STROBE reporting checklist (available at https://qims.amegroups.com/article/view/10.21037/qims-24-1265/rc).
Methods
Data acquisition
In total, 88 ESRD patients at the Department of Nephrology, Beijing Friendship Hospital, Capital Medical University were included, 45 of whom did not receive dialysis treatment, and 52 local healthy controls (HCs) were included in this study. All the study participants were right-handed, and there was no documented history of renal disease among any of the HCs. Patients were excluded from the study if they met any of the following exclusion criteria: (I) had a history of a psychiatric disorder; (II) had a neurological disorder, including stroke, brain tumor, and brain trauma; (III) had another systemic disease; (IV) had contraindications to MRI; and/or (V) had significant head movement during MRI examination. In addition, eight ESRD patients and six HCs were excluded due to claustrophobia (n=4), chronic infarction (n=1), and excessive head movement (≥3 mm/3°) (n=9). The details of the study population are illustrated in Figure 1.
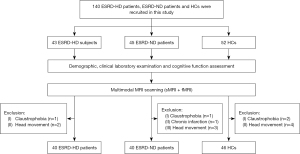
The study was conducted in accordance with the Declaration of Helsinki (as revised in 2013). The study was approved by the Ethics Committee of Beijing Friendship Hospital, Capital Medical University (No. BFHHZS20220112). Informed consent for this study was obtained from all study participants or the legally authorized representatives of the illiterate participants.
Prior to undergoing scanning, all patients diagnosed with ESRD underwent cognitive assessments and biochemical blood examinations.
The images were acquired using an 8-channel phased array coil on a 3.0-Tesla magnetic resonance scanner (Discovery MR750w, GE HealthCare, Anaheim, USA). Throughout the scanning procedure, the participants were instructed to maintain a supine position, keep their heads immobilized, close their eyes, and remain awake while trying to avoid any thinking activity. The patients were also provided earplugs for noise reduction, and offered foam pads to enhance their comfort and minimize their head movement. The acquisition of high-resolution T1-weighted images (T1WI) was performed using a three-dimensional (3D) brain volume imaging (BRAVO) sequence, employing the following parameters: slices, 192; slice thickness, 1 mm (no gap); repetition time (TR), 8.8 ms; echo time (TE), 3.5 ms; inversion time (TI), 450 ms; flip angle (FA), 15°; field of view (FOV), 240 mm × 240 mm; matrix, 256 × 256; and acquisition time, 276 s. The parameters employed for the acquisition of the resting-state functional data were as follows: time points, 200; slices, 28; slice thickness, 5 mm (1-mm gap); TR, 2,000 ms; TE, 35 ms; FOV, 240 mm × 240 mm; FA, 90°; matrix, 64×64; and acquisition time, 400 s.
Anatomical data preprocessing
All cortical parameters were calculated by analyzing the T1WI and rs-fMRI data using DPABISurf (version 1.8) software (http://rfmri.org/DPABISurf) (23). The DPABISurf pre-processed the structural and functional MRI data using the fMRIPrep pipeline [integrating FreeSurfer, advanced normalization tools (ANTs), FMRIB software library (FSL), and analysis of functional neuroimages (AFNI)] (24). The cerebral cortex was separated by the Human Connectome Project (HCP) template built into the DPABISurf software. The whole process was automated, and comprised the following steps: (I) the T1WI were corrected for intensity inhomogeneity using N4BiasFieldCorrection (25), distributed through ANTs (version 2.2.0) (26), and used as the T1WI references throughout the entire process; (II) the T1WI references were skull-stripped using antsBrainExtraction.sh (ANTs version 2.2.0) with OASIS30ANTs as the template for target; (III) Recon-All l (FreeSurfer 6.0.) was used to reconstruct the brain surface (27). A custom variant of the method was used to refine the previously estimated brain mask to coordinate ANTs-derived and Freesurfer-derived cortical gray matter (GM) segmentation in Mindboggle (28); (IV) based on the brain-extracted T1WI volumes and templates, a nonlinear regression analysis with antsRegistration (ANTS version 2.2.0) was conducted to realize the spatial normalization of the international consortium for brain mapping (ICBM) 152 nonlinear asymmetric template (version 2009C); and (V) brain tissue segmentation of white matter (WM), cerebrospinal fluid (CSF), and GM was performed on the brain-extracted T1WI by fast (FSL version 5.0.9) (29).
The functional data were pre-processed as follows: (I) a custom fMRIPrep method was used to generate the reference volume and its skull-stripped version; (II) bbregister (FreeSurfer) was used to co-register blood oxygen level dependent imaging (BOLD) references to the T1WI references, implementing boundary-based registration (30); (III) slice time correction was performed for the BOLD run using 3dTshift (31), and the BOLD time series was processed into FreeSurfer fsaverage5 surface space; (IV) DPABISurf was used to perform harmful covariate regression to eliminate signals of head movement, WM, and CSF; and (V) the data were filtered and smoothed as follows: normalized functional images were processed with a band-pass time filter (0.01 to 0.1 Hz) and spatial smoothing (full-width at half-maximum of 6 mm).
After the above processing steps, the cortical-based structural parameters (i.e., cortical thickness and cortical volume) and functional parameters [i.e., amplitude of low frequency fluctuation (ALFF), fractional ALFF (fALFF), and regional homogeneity (ReHo)] were obtained. ALFF represents the average of ALFFs (0.01 to 0.1 Hz) based on the fast Fourier transform of each voxel over time (32). fALFF is a normalized ALFF, and is determined by dividing the total power in the low frequency range (0.01–0.1 Hz) by the total power in the full frequency range of the same element (33). ReHo represents the homogeneity of the time process of a given voxel relative to the time process of the nearest 26 neighboring voxels, and is calculated based on Kendall’s coefficient of consistency (34).
Statistical analysis
The demographic and clinical characteristics are presented as the percentage; the continuous variables are expressed as the mean ± standard deviation for those that conformed to normal distribution, and the median value and interquartile spacing for those that did not. The ESRD-ND, ESRD-HD, and HC groups were compared using the Chi-squared test for the categorical variables, and the two-sample t-test or Mann-Whitney U test for the continuous variables. In relation to the cortical parameters, a P value <0.05 (a P value <0.025 for each hemisphere) was considered statistically significant in the cortical analysis [family-wise error (FWE) corrected]. The significance level was set at P<0.05 to determine statistical significance in accordance with established conventions. DPABISurf was employed as a tool for visualizing the results relating to the cortical parameters. A partial correlation analysis with sex, age, and education level as covariates was conducted to examine the relationship between the cortical parameters (i.e., cortical thickness, cortical volume, ALFF, fALFF, and ReHo) and the patient/disease-related rating scale score (i.e., the MoCA score). The test results were adjusted using the Bonferroni method (P<0.05/4=0.0125) in multiple comparisons.
Results
Demographics and clinical measures of participants
In total, 40 ESRD-ND patients, 40 ESRD-HD patients, and 46 HCs were included in this study. The characteristics of the participants, including their demographics and clinical profiles, are provided in Table 1. There were no statistically significant differences among the three groups in terms of age (P=0.393), sex (P=0.067), or education level (P=0.448). However, in terms of the laboratory test findings, significant differences were observed in the blood concentrations of urea, creatinine, hemoglobin, and calcium (Ca2+) between the ESRD-ND and ESRD-HD groups (P<0.05). The results obtained from the neuropsychological assessments revealed a significant disparity in the MoCA scores between the ESRD-ND and ESRD-HD groups (P<0.001).
Table 1
Variables | ESRD-ND (n=40) | ESRD-HD (n=40) | HCs (n=46) | P value |
---|---|---|---|---|
Age (years) | 50.0 (39.5, 59.75) | 55.5 (45.5, 60.75) | 53.5 (42.75, 59.0) | 0.393† |
Sex, male/female | 29/11 | 24/16 | 22/24 | 0.067‡ |
Education level (years) | 12.0 (12.0, 15.0) | 12.0 (12.0, 14.0) | 14.0 (9.0, 16.0) | 0.488† |
Urea (mmol/L)† | 30.0±8.3 | 18.6±8.4 | NA | <0.001§ |
Creatinine (µmol/L)† | 777.0±251.4 | 983.3±208.5 | NA | <0.001§ |
UA (µmol/L) | 408.4±128.4 | 370.4±78.1 | NA | 0.303§ |
Calcium (mmol/L) | 2.1 (1.9, 2.3) | 2.3 (2.2, 2.4) | NA | <0.001¶ |
Phosphate (mmol/L) | 2.0±0.5 | 2.0±0.4 | NA | 0.459§ |
eGFR (mL/min/1.73 m2) | 5.9 (4.25, 8.24) | 2.5 (2.20, 3.2) | NA | <0.001¶ |
Hemoglobin (g/L) | 98 (85.5, 105.0) | 116 (109.0, 122.8) | NA | <0.001¶ |
Parathyroid hormone (pg/mL) | 207.9 (115.0, 380.2) | 206.4 (87.0, 333.0) | NA | 0.580¶ |
MoCA score | 24.5 (22.3, 27.0) | 21 (18.0, 25.0) | NA | <0.001§ |
Data are presented as the median (25th percentile, 75th percentile) or mean ± standard deviation. †, Kruskal-Wallis test; ‡, Chi-squared test; §, two-sample t-test; ¶, Mann-Whitney U test. ESRD, end-stage renal disease; HCs, healthy controls; ESRD-ND, end-stage renal disease without dialysis; ESRD-HD, end-stage renal disease with hemodialysis; NA, not applicable; UA, uric acid; eGFR, estimated glomerular filtration rate; MoCA, Montreal Cognitive Assessment.
Analysis of the cortical structure
The thickness and volume of the left medial temporal cortex were smaller in the ESRD-ND group than in the HC group. Both the thickness and volume of the bilateral medial temporal cortex were reduced in the ESRD-HD group compared to the ESRD-ND group. The cortical thickness of the bilateral temporo-parieto-occipital junction was smaller in the ESRD-ND group than in the HC group. The cortical volume of paracentral lobular and mid cingulate cortex was smaller in the ESRD-ND and ESRD-HD groups than the HC group, while that of the ESRD-HD group was smaller than that of the ESRD-ND group. The cortical volume of the insular and frontal opercular cortex and the anterior cingulate and medial prefrontal cortex (AntCing_MedPFC) was smaller in the ESRD-HD group than in the ESRD-ND and HC group, but no difference was found between the ESRD-ND and HC groups (Figure 2 and Table 2).
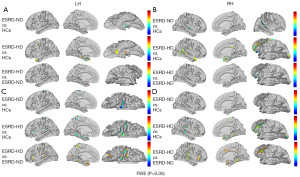
Table 2
Anatomical region | HCP | MNI coordinate | Cluster size (mm) | Peak T value |
||
---|---|---|---|---|---|---|
X | Y | Z | ||||
Thickness | ||||||
Left brain | ||||||
ESRD-ND < HC | ||||||
Temporo-parieto-occipital junction | 25 | –48 | –43 | 20 | 447 | –4.64 |
Medial temporal cortex | 126 | –27 | –27 | –24 | 233 | –4.02 |
ESRD-HD < HC | ||||||
Left lateral temporal cortex | 118 | –23 | –15 | –27 | 1,629 | –8.43 |
Insular and frontal opercular cortex | 167 | –41 | –26 | –1 | 277 | –4.38 |
HD < ESRD-ND | ||||||
Medial temporal cortex | 120 | –24 | –14 | –29 | 600 | –21.76 |
Right brain | ||||||
ESRD-ND < HC | ||||||
Temporo-parieto-occipital junction | 25 | 46 | –36 | 14 | 549 | –5.45 |
ESRD-HD < HC | ||||||
Medial temporal cortex | 118 | 25 | –14 | –27 | 1,369 | –7.23 |
Temporo-parieto-occipital junction | 139 | 44 | –50 | 20 | 785 | –4.91 |
Paracentral lobular and mid cingulate cortex | 55 | 14 | –19 | 71 | 246 | –4.20 |
ESRD-HD < ESRD-ND | ||||||
Medial temporal cortex | 120 | 24 | –12 | –29 | 458 | –18.08 |
Volume | ||||||
Left brain | ||||||
ESRD-ND < HC | ||||||
Paracentral lobular and mid cingulate cortex | 55 | –7 | –27 | 73 | 828 | –5.42 |
ESRD-HD < HC | ||||||
Medial temporal cortex | 155 | –22 | –17 | –26 | 1,433 | –4.79 |
Paracentral lobular and mid cingulate cortex | 55 | –18 | –7 | –56 | 945 | –4.74 |
Insular and frontal opercular cortex | 178 | –37 | –27 | 0.1 | 939 | –6.19 |
Anterior cingulate and medial prefrontal cortex | 166 | –10 | 22 | –17 | 242 | –4.81 |
ESRD-HD < ESRD-ND | ||||||
Insular and frontal opercular cortex | 178 | –34 | –27 | 3 | 1,976 | –8.47 |
Medial temporal cortex | 126 | –34 | –40 | –11 | 708 | –9.68 |
Anterior cingulate and medial prefrontal cortex | 166 | –14 | 20 | –14 | 223 | –6.15 |
Right brain | ||||||
ESRD-HD < HC | ||||||
Insular and frontal opercular cortex | 178 | 38 | –25 | 0 | 2,021 | –6.01 |
Temporo-parieto-occipital junction | 139 | 48 | –36 | 5 | 1,232 | –7.26 |
Medial temporal cortex | 155 | 30 | –41 | –10 | 1,042 | –6.31 |
Paracentral lobular and mid cingulate cortex | 55 | 9 | –16 | 69 | 581 | –5.83 |
Anterior cingulate and medial prefrontal cortex | 166 | 13 | 20 | –14 | 355 | –5.68 |
ESRD-HD < ESRD-ND | ||||||
Insular and frontal opercular cortex | 178 | 41 | –37 | 18 | 3,078 | –8.42 |
Temporo-parieto-occipital junction | 139 | 47 | –21 | –13 | 1,432 | –8.82 |
Medial temporal cortex | 155 | 37 | –31 | –17 | 841 | –8.76 |
Inferior parietal cortex | 146 | 28 | –64 | 27 | 454 | –5.91 |
Anterior cingulate and medial prefrontal cortex | 60 | 14 | 20 | 33 | 230 | –4.75 |
ESRD-ND, end-stage renal disease without dialysis; HC, healthy control; HD, hemodialysis; ESRD-HD, end-stage renal disease with hemodialysis; HCP, Human Connectome Project; MNI, Montreal Neurological Institute.
Analysis of cortical function
The fALFF of the bilateral inferior parietal cortex was found to be comparatively reduced in the ESRD-ND group compared to the HC group, and the fALFF of the bilateral medial temporal cortex was higher in the ESRD-ND group than in the HC group. The ReHo of the double medial temporal cortex was higher in the ESRD-HD group than in the ESRD-ND group, while that of the ESRD-ND group was higher than that of the HC group. The ReHo of the AntCing_MedPFC was higher in the ESRD-HD and ESRD-ND groups than in the HC group (Figure 3 and Table 3).
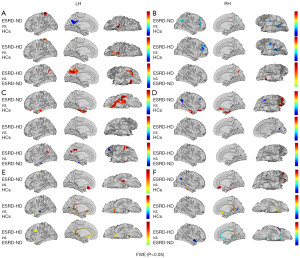
Table 3
Anatomical region | HCP | MNI coordinate | Cluster | Cluster size (mm) | Peak T value |
|||
---|---|---|---|---|---|---|---|---|
X | Y | Z | size | |||||
fALFF | ||||||||
Left brain | ||||||||
ESRD-ND < HC | ||||||||
Inferior parietal cortex | 144 | –39 | –54 | 35 | 47 | 141.457 | –4.484 | |
Right brain | ||||||||
ESRD-ND < HC | ||||||||
Inferior parietal cortex | 150 | 48 | –60 | 31 | 61 | 223.925 | –5.13 | |
ReHo | ||||||||
Left brain | ||||||||
ESRD-ND > HC | ||||||||
Anterior cingulate and medial prefrontal cortex | 164 | –7 | 25 | –14 | 41 | 212.097 | 4.23 | |
Medial temporal cortex | 122 | –29 | –30 | –19 | 33 | 117.989 | 3.90 | |
ESRD-HD > HC | ||||||||
Medial temporal cortex | 119 | –5 | –45 | 17 | 122 | 270.02 | 7.89 | |
Anterior cingulate and medial prefrontal cortex | 166 | –5 | 22 | –7 | 73 | 263.497 | 8.2 | |
ESRD-HD > ESRD-ND | ||||||||
Medial temporal cortex | 119 | –5 | –45 | 17 | 221 | 583.403 | 19.16 | |
Right brain | ||||||||
ESRD-ND > HC | ||||||||
Medial temporal cortex | 135 | 36 | –12 | –32 | 36 | 149.863 | 4.15 | |
Anterior cingulate and medial prefrontal cortex | 88 | 7 | 37 | –18 | 33 | 190.497 | 4.95 | |
ESRD-HD > HC | ||||||||
Anterior cingulate and medial prefrontal cortex | 166 | 6 | –45 | 15 | 206 | 586.346 | 10.48 | |
ESRD-HD > ESRD-ND | ||||||||
Medial temporal cortex | 119 | 4 | –36 | 24 | 219 | 580.38 | 20.05 |
ALFF, amplitude of low frequency fluctuation; fALFF, fractional ALFF; ESRD-ND, end-stage renal disease without dialysis; HC, healthy control; ReHo, regional homogeneity; ESRD-HD, end-stage renal disease with hemodialysis; HCP, Human Connectome Project; MNI, Montreal Neurological Institute.
Correlation analysis
In ESRD-HD, the cortical thickness (r=0.359, P=0.023; corrected r=0.360, P=0.026) and volume (r=0.433, P=0.005; corrected r=0.440, P=0.006) of the left medial temporal cortex were positively correlated with the MoCA score. The fALFF value (r=–0.325, P=0.041; corrected r=0.028, P=0.869) and ReHo value (r=–0.447, P=0.004; corrected r=–0.206, P=0.214) of the left medial temporal cortex were negatively correlated with the MoCA score. The ReHo value (r=–0.350, P=0.027; corrected r=–0.165, P=0.332) of the left AntCing_MedPFC was negatively correlated with the MoCA score. Conversely, in ESRD-ND, the cortical thickness (r=0.604, P<0.001; corrected r=0.529, P=0.001) and volume (r=0.435, P=0.005; corrected r=0.571, P<0.001) of the right posterior cingulate cortex were positively correlated with the MoCA score, and the cortical thickness (r=0.378, P=0.016; corrected r=0.243, P=0.148) of the temporo-parieto-occipital junction was positively correlated with the MoCA score (Figure 4).
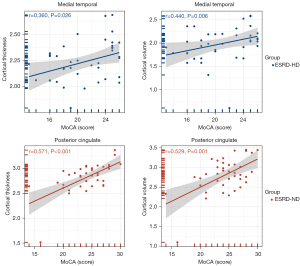
Discussion
This study used multimodal MRI to evaluate brain function and cortical changes in ESRD-ND and ESRD-HD patients. The patients in the ESRD-HD and ESRD-ND groups showed alterations in brain function and cortical structural features compared to the HCs. Additionally, the ESRD-HD group displayed unique changes in brain function and cortical structural features compared to both the HC and ESRD-ND groups. This study sought to explore functional and structural changes to elucidate the underlying mechanisms that contribute to cognitive impairment in patients with ESRD with and without dialysis. Our findings may help to identifying early diagnostic indicators for cognitive dysfunction in ESRD patients with and without dialysis.
We used surface-based morphometry to investigate cortical thickness and volume alterations in ESRD-ND and ESRD-HD patients. The left medial temporal cortex was thinner and had a smaller volume in the ESRD-ND group than in the HC group. The double medial temporal cortex was also thinner and had a smaller volume in the ESRD-HD group than in the ESRD-ND group. The hippocampus, perirhinal cortex, para-hippocampal cortex, and entorhinal cortex are crucial components of the medial temporal lobe memory system due to their vital role in the processing of long-term memories (35). The visual-mnemonic is typically backed by the presentation of the medial temporal lobe’s involvement in visual processing (36). The medial temporal lobe plays a crucial role in supporting memory recall (the recall effect) (37). If the medial temporal lobe is damaged, it can lead to abnormalities in the memory and recall of known things. The process of ESRD and dialysis can aggravate the atrophy of the medial temporal lobe, leading to a decline in cognitive function.
The fALFF in the bilateral inferior parietal cortex was lower in the ND group than in the HC group. The inferior parietal lobule is an important node in the DMN and plays a key role in many cognitive functions (38). The decrease of parietal spontaneous activity may be a potential cause of cognitive decline in ESRD-ND patients. The value of ReHo in the double medial temporal cortex was greater in the ESRD-HD group than in the ESRD-ND group, while it was greater in the ESRD-ND group than in the HC group. Further, the AntCing_MedPFC exhibited significantly elevated ReHo values in both the ESRD-HD and ESRD-ND groups compared to those observed in the HC group. We speculate that this may be a generational change, and that ESRD patients may reduce the negative effects of rib cortical structural changes and decreased spontaneous activity by increasing ReHo.
In this study, we found that the cortical thickness and volume of the medial temporal lobe were reduced in both the ESRD-ND and ESRD-HD groups compared with the HC group, and those of the ESRD-HD group were smaller than those of ESRD-ND group. Previous studies have shown that the cortical thickness of the medial temporal lobe is associated with cognitive impairment (39,40). Similarly, this study showed that the cortical thickness and volume of the medial temporal lobe were positively correlated with the MoCA score in the ESRD-HD patients. Thus, medial temporal lobe involvement may be an important cause of cognitive impairment in ESRD-HD patients. The thickness and volume of the cingulate cortex in the ESRD-ND group were proportional to the MoCA score. Somewhat surprisingly, the original negative correlation between the ReHo values of the AntCing_MedPFC and the MoCA scores became non-correlated after adjusting for covariates of age, sex and education. This may mean that changes in cortical structure may be a more reliable neuroimaging marker than changes in function.
This study had a number of limitations. First, the sample size of the study was small. Thus, future studies should be conducted with larger sample sizes to increase the reliability of the results. Second, this study adopted a cross-sectional design. Thus, future longitudinal studies need to be conducted.
Conclusions
Our findings suggest ESRD patients undergo cognitively related cortical structure and function changes. Dialysis can aggravate or cause changes in new brain regions. The atrophy of posterior cingulate cortex is an important cause of cognitive decline in ESRD patients. Dialysis can aggravate the damage of the middle temporal lobe and lead to further cognitive impairment.
Acknowledgments
Funding: This work was supported by
Footnote
Reporting Checklist: The authors have completed the STROBE reporting checklist. Available at https://qims.amegroups.com/article/view/10.21037/qims-24-1265/rc
Conflicts of Interest: All authors have completed the ICMJE uniform disclosure form (available at https://qims.amegroups.com/article/view/10.21037/qims-24-1265/coif). B.X. is an employee of MR Research, GE Healthcare, Beijing, China. The other authors have no conflicts of interest to declare.
Ethical Statement: The authors are accountable for all aspects of the work in ensuring that questions related to the accuracy or integrity of any part of the work are appropriately investigated and resolved. The study was approved by the Ethics Committee of Beijing Friendship Hospital, Capital Medical University (No. BFHHZS20220112). The study was conducted in accordance with the Declaration of Helsinki (as revised in 2013). Informed consent for this study was obtained from all study participants or the legally authorized representatives of the illiterate participants.
Open Access Statement: This is an Open Access article distributed in accordance with the Creative Commons Attribution-NonCommercial-NoDerivs 4.0 International License (CC BY-NC-ND 4.0), which permits the non-commercial replication and distribution of the article with the strict proviso that no changes or edits are made and the original work is properly cited (including links to both the formal publication through the relevant DOI and the license). See: https://creativecommons.org/licenses/by-nc-nd/4.0/.
References
- Webster AC, Nagler EV, Morton RL, Masson P. Chronic Kidney Disease. Lancet 2017;389:1238-52. [Crossref] [PubMed]
- Lizio R, Lopez S, Babiloni C, Del Percio C, Noce G, Losurdo A, et al. Resting state EEG rhythms in different stages of chronic kidney disease with mild cognitive impairment. Neurobiol Aging 2023;130:70-9. [Crossref] [PubMed]
- Lau WL, Fisher M. New insights into cognitive decline in chronic kidney disease. Nat Rev Nephrol 2023;19:214-5. [Crossref] [PubMed]
- Jones DJ, Harris JP, Vaux E, Hadid R, Kean R, Butler LT. The nature of impairments of memory in patients with end-stage renal disease (ESRD). Physiol Behav 2015;147:324-33. [Crossref] [PubMed]
- Berger I, Wu S, Masson P, Kelly PJ, Duthie FA, Whiteley W, Parker D, Gillespie D, Webster AC. Cognition in chronic kidney disease: a systematic review and meta-analysis. BMC Med 2016;14:206. [Crossref] [PubMed]
- Mendley SR, Matheson MB, Shinnar S, Lande MB, Gerson AC, Butler RW, Warady BA, Furth SL, Hooper SR. Duration of chronic kidney disease reduces attention and executive function in pediatric patients. Kidney Int 2015;87:800-6. [Crossref] [PubMed]
- Puy L, Bugnicourt JM, Liabeuf S, Desjardins L, Roussel M, Diouf M, Chillon JM, Choukroun G, Massy ZA, Godefroy O. Cognitive Impairments and Dysexecutive Behavioral Disorders in Chronic Kidney Disease. J Neuropsychiatry Clin Neurosci 2018;30:310-7. [Crossref] [PubMed]
- Wouk N. End-Stage Renal Disease: Medical Management. Am Fam Physician 2021;104:493-9. [PubMed]
- Wolfgram DF. Intradialytic Cerebral Hypoperfusion as Mechanism for Cognitive Impairment in Patients on Hemodialysis. J Am Soc Nephrol 2019;30:2052-8. [Crossref] [PubMed]
- Natale P, Palmer SC, Ruospo M, Saglimbene VM, Rabindranath KS, Strippoli GF. Psychosocial interventions for preventing and treating depression in dialysis patients. Cochrane Database Syst Rev 2019;12:CD004542. [Crossref] [PubMed]
- Bugnicourt JM, Godefroy O, Chillon JM, Choukroun G, Massy ZA. Cognitive disorders and dementia in CKD: the neglected kidney-brain axis. J Am Soc Nephrol 2013;24:353-63. [Crossref] [PubMed]
- Ali S, Dave N, Virani SS, Navaneethan SD. Primary and Secondary Prevention of Cardiovascular Disease in Patients with Chronic Kidney Disease. Curr Atheroscler Rep 2019;21:32. [Crossref] [PubMed]
- Greco F, Quarta LG, Parizel PM, Zobel BB, Quattrocchi CC, Mallio CA. Relationship between chronic kidney disease and cerebral white matter hyperintensities: a systematic review. Quant Imaging Med Surg 2023;13:7596-606. [Crossref] [PubMed]
- Noguchi Y, Nagasawa H, Tachi T, Tsuchiya T, Teramachi H. Signal detection of oral drug-induced dementia in chronic kidney disease patients using association rule mining and Bayesian confidence propagation neural network. Pharmazie 2019;74:570-4. [PubMed]
- Mazumder MK, Paul R, Bhattacharya P, Borah A. Neurological sequel of chronic kidney disease: From diminished Acetylcholinesterase activity to mitochondrial dysfunctions, oxidative stress and inflammation in mice brain. Sci Rep 2019;9:3097. [Crossref] [PubMed]
- Viggiano D, Wagner CA, Martino G, Nedergaard M, Zoccali C, Unwin R, Capasso G. Mechanisms of cognitive dysfunction in CKD. Nat Rev Nephrol 2020;16:452-69. [Crossref] [PubMed]
- Canaud B, Chazot C, Koomans J, Collins A. Fluid and hemodynamic management in hemodialysis patients: challenges and opportunities. J Bras Nefrol 2019;41:550-9. [Crossref] [PubMed]
- Liu HS, Hartung EA, Jawad AF, Ware JB, Laney N, Port AM, Gur RC, Hooper SR, Radcliffe J, Furth SL, Detre JA. Regional Cerebral Blood Flow in Children and Young Adults with Chronic Kidney Disease. Radiology 2018;288:849-58. [Crossref] [PubMed]
- Zhang LJ, Wen J, Ni L, Zhong J, Liang X, Zheng G, Lu GM. Predominant gray matter volume loss in patients with end-stage renal disease: a voxel-based morphometry study. Metab Brain Dis 2013;28:647-54. [Crossref] [PubMed]
- Tsuruya K, Yoshida H, Haruyama N, Fujisaki K, Hirakata H, Kitazono T. Clinical Significance of Fronto-Temporal Gray Matter Atrophy in Executive Dysfunction in Patients with Chronic Kidney Disease: The VCOHP Study. PLoS One 2015;10:e0143706. [Crossref] [PubMed]
- Gu Z, Lu H, Zhou H, Zhang J, Xing W. The relationship between abnormal cortical activity in the anterior cingulate gyrus and cognitive dysfunction in patients with end- stage renal disease: a fMRI study on the amplitude of low- frequency fluctuations. Ann Palliat Med 2020;9:4187-93. [Crossref] [PubMed]
- Guo H, Liu W, Li H, Yang J. Structural and Functional Brain Changes in Hemodialysis Patients with End-Stage Renal Disease: DTI Analysis Results and ALFF Analysis Results. Int J Nephrol Renovasc Dis 2021;14:77-86. [Crossref] [PubMed]
- Yan CG, Wang XD, Lu B. DPABISurf: data processing & analysis for brain imaging on surface. Sci Bull (Beijing) 2021;66:2453-5. [Crossref] [PubMed]
- Esteban O, Markiewicz CJ, Blair RW, Moodie CA, Isik AI, Erramuzpe A, Kent JD, Goncalves M, DuPre E, Snyder M, Oya H, Ghosh SS, Wright J, Durnez J, Poldrack RA, Gorgolewski KJ. fMRIPrep: a robust preprocessing pipeline for functional MRI. Nat Methods 2019;16:111-6. [Crossref] [PubMed]
- Tustison NJ, Avants BB, Cook PA, Zheng Y, Egan A, Yushkevich PA, Gee JC. N4ITK: improved N3 bias correction. IEEE Trans Med Imaging 2010;29:1310-20. [Crossref] [PubMed]
- Avants BB, Epstein CL, Grossman M, Gee JC. Symmetric diffeomorphic image registration with cross-correlation: evaluating automated labeling of elderly and neurodegenerative brain. Med Image Anal 2008;12:26-41. [Crossref] [PubMed]
- Dale AM, Fischl B, Sereno MI. Cortical surface-based analysis. I. Segmentation and surface reconstruction. Neuroimage 1999;9:179-94. [Crossref] [PubMed]
- Klein A, Ghosh SS, Bao FS, Giard J, Häme Y, Stavsky E, Lee N, Rossa B, Reuter M, Chaibub Neto E, Keshavan A. Mindboggling morphometry of human brains. PLoS Comput Biol 2017;13:e1005350. [Crossref] [PubMed]
- Zhang Y, Brady M, Smith S. Segmentation of brain MR images through a hidden Markov random field model and the expectation-maximization algorithm. IEEE Trans Med Imaging 2001;20:45-57. [Crossref] [PubMed]
- Greve DN, Fischl B. Accurate and robust brain image alignment using boundary-based registration. Neuroimage 2009;48:63-72. [Crossref] [PubMed]
- Cox RW, Hyde JS. Software tools for analysis and visualization of fMRI data. NMR Biomed 1997;10:171-8. [Crossref] [PubMed]
- Zang YF, He Y, Zhu CZ, Cao QJ, Sui MQ, Liang M, Tian LX, Jiang TZ, Wang YF. Altered baseline brain activity in children with ADHD revealed by resting-state functional MRI. Brain Dev 2007;29:83-91. [Crossref] [PubMed]
- Zou QH, Zhu CZ, Yang Y, Zuo XN, Long XY, Cao QJ, Wang YF, Zang YF. An improved approach to detection of amplitude of low-frequency fluctuation (ALFF) for resting-state fMRI: fractional ALFF. J Neurosci Methods 2008;172:137-41. [Crossref] [PubMed]
- Zang Y, Jiang T, Lu Y, He Y, Tian L. Regional homogeneity approach to fMRI data analysis. Neuroimage 2004;22:394-400. [Crossref] [PubMed]
- Lech RK, Suchan B. The medial temporal lobe: memory and beyond. Behav Brain Res 2013;254:45-9. [Crossref] [PubMed]
- Lech RK, Suchan B. Involvement of the human medial temporal lobe in a visual discrimination task. Behav Brain Res 2014;268:22-30. [Crossref] [PubMed]
- de Vanssay-Maigne A, Noulhiane M, Devauchelle AD, Rodrigo S, Baudoin-Chial S, Meder JF, Oppenheim C, Chiron C, Chassoux F. Modulation of encoding and retrieval by recollection and familiarity: mapping the medial temporal lobe networks. Neuroimage 2011;58:1131-8. [Crossref] [PubMed]
- Wang J, Xie S, Guo X, Becker B, Fox PT, Eickhoff SB, Jiang T. Correspondent Functional Topography of the Human Left Inferior Parietal Lobule at Rest and Under Task Revealed Using Resting-State fMRI and Coactivation Based Parcellation. Hum Brain Mapp 2017;38:1659-75. [Crossref] [PubMed]
- Kaestner E, Reyes A, Chen A, Rao J, Macari AC, Choi JY, Qiu D, Hewitt K, Wang ZI, Drane DL, Hermann B, Busch RM, Punia V, McDonald CRAlzheimer’s Disease Neuroimaging Initiative. Atrophy and cognitive profiles in older adults with temporal lobe epilepsy are similar to mild cognitive impairment. Brain 2021;144:236-50. [Crossref] [PubMed]
- Soldan A, Pettigrew C, Lu Y, Wang MC, Selnes O, Albert M, Brown T, Ratnanather JT, Younes L, Miller MIBIOCARD Research Team. Relationship of medial temporal lobe atrophy, APOE genotype, and cognitive reserve in preclinical Alzheimer's disease. Hum Brain Mapp 2015;36:2826-41. [Crossref] [PubMed]