Exploring the use of biphasic quantitative thoracic computed tomography to evaluate halo-pelvic traction in managing severe adolescent idiopathic scoliosis
Introduction
Severe adolescent idiopathic scoliosis (AIS) is a complex 3-dimensional (3D) spinal deformity that often presents with pronounced impairment of cardiopulmonary function (1,2). The current method for treating spinal deformities involves osteotomy and internal fixation. However, managing severe rigid scoliosis is challenging due to the intricate nature of the required complex osteotomy and extensive correction. These procedures substantially elevate the risk of neurologic, vascular, and pulmonary complications (3,4). Preoperative halo-pelvic traction (HPT), as an effective and safe solution before surgery, increases the safety and correction rate in the surgical treatment of severe spinal deformities (5-9).
Computed tomography (CT) is a valuable tool that can not only assess morphology but also evaluate respiratory function (10). Previous studies (5,8,9) have examined the effects of HPT on pulmonary function tests (PFTs) and radiographic parameters. However, there is a scarcity of published research utilizing CT to investigate alterations in lung volume following HPT.
This study aimed to utilize biphasic quantitative CT scans to evaluate the alterations in lung volume and anatomical parameters in patients with severe AIS before and after HPT. Additionally, the study sought to determine whether biphasic thoracic CT offers advantages over monophasic CT in the treatment of severe AIS. The hypothesis was that the lung tidal volume, as determined by CT scans at end inspiration and expiration, would exhibit changes in conjunction with PFTs. We present this article in accordance with the STROBE reporting checklist (available at https://qims.amegroups.com/article/view/10.21037/qims-24-568/rc).
Methods
Patients
The cohort for this study consisted of 5 male and 19 female patients, with an average age of 15.8±2.2 years before HPT. A priori sample size calculations indicated that a minimum of 15 patients were required. The medical records of patients diagnosed with AIS who underwent HPT and surgical correction were reviewed. All patients underwent staged treatment, initially receiving HPT until sufficient improvement in pulmonary function was achieved, followed by spinal corrective surgery. The inclusion criteria were as follows: (I) diagnosis of AIS and undergoing HPT before surgery; (II) presence of a major thoracic curve ≥80 degrees; (III) availability of pre- and post-traction radiographs, CT scans, and PFTs; and (IV) CT scans obtained using the same scanner model. The exclusion criteria were as follows: (I) prior spinal surgery; and (II) inability to obtain CT lung volumes through reconstruction. Figure 1 illustrates the patient enrollment flowchart. Clinical data, including apical vertebrae, HPT duration, and demographic information [sex, age, height, arm span, and body mass index (BMI)], were gathered for each patient. The study was conducted in accordance with the Declaration of Helsinki (as revised in 2013). Approval for the study was obtained from the Ethics Committee of the Third People’s Hospital of Chengdu (No. 2024-S-105). The requirement for individual consent for this retrospective analysis was waived.
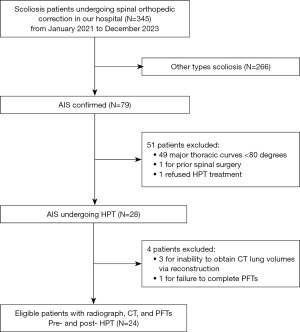
HPT
The HPT device comprised halo and pelvic rings, which were affixed to the cranial and iliac bones, respectively. It featured 4 correcting rods that aligned the rings for spinal distraction. The fitting procedure required general anesthesia. The halo frame was positioned above the right skull equator and secured with 10 pins tightened to a torque of 6–8 inches. Then, 2 pelvic ring pins were inserted at the iliac crest and the posterior superior iliac spine. After the patient had adapted to the device and was confident in routine activities, the halo-pelvic apparatus was fitted. Traction commenced with the bars being distracted by approximately 4–5 mm weekly. Traction was temporarily halted if traction-related symptoms, such as neck pain or numbness, occurred. Respiratory exercises, including deep breathing, abdominal breathing, lip-constriction breathing, and balloon exercises, were performed during traction. Additionally, physical exercise was conducted to improve cardiopulmonary fitness. If pulmonary function had plateaued and the patient could tolerate complex surgery, surgery might have been considered. Figure 2 illustrates the process and appearance of HPT.
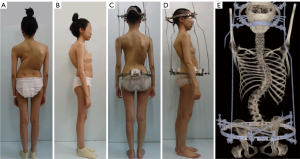
Radiographic parameters
Full-length standing anteroposterior and lateral radiographs were obtained before and after HPT. Full spine radiographs were obtained using 2 distinct X-ray systems: a GE Definium 6000 (GE Healthcare, Chicago, IL, USA) for conventional radiography and a Shimadzu Sonialvsion C200 (Shimadzu, Kyoto, Japan) for slot radiography. Patients were positioned upright with minimal movement, ensuring imaging coverage from the foramen magnum to the femoral head. The imaging technique required precise patient positioning and alignment of the central ray with the spinal axis. The machine’s current and exposure time were automatically controlled. The focal distance was set at 150 cm. The major thoracic Cobb angle and thoracic height (T1–T12) were measured on anteroposterior radiographs, whereas thoracic kyphosis (T5–T12) was measured on lateral radiographs. The thoracic height was defined as the vertical distance between the T1 and T12 vertebral bodies, as illustrated in Figure 3A,3B.
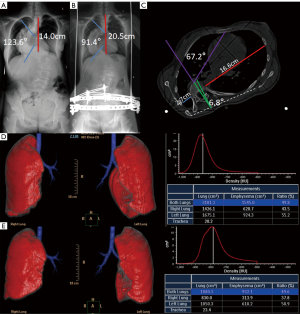
CT scanning and quantitative image analysis
All patients enrolled in the study underwent unenhanced and enhanced scanning using a Philips 256-slice Brilliance iCT scanner (Philips, Amsterdam, The Netherlands) both before and after HPT. To maintain consistent lung volume, patients held their breath at end inspiration during the enhanced scanning and at end expiration during the unenhanced scanning. Low-dose CT scans were acquired with a collimation of 0.625 mm and autoexposure control, adjusting the exposure based on the patient’s size. The hemithoracic symmetry ratio, thoracic rotation, and spinal rotation at the apical vertebral level were calculated using the method described by Campbell et al. (11), as follows (Figure 3C).
- Hemithoracic symmetry ratio: the posterior aspect of the thorax was used as a reference point, specifically the anterior tip of the rib heads where they connected with the spine. The distance from the spine at each rib head to the inner border of the hemithorax was measured. This measurement was then used to calculate the ratio by dividing the larger distance by the smaller distance. In a normal thorax, this ratio is equal to 1. However, as a rib hump develops, the ratio increases, indicating asymmetry in the thorax.
- Thoracic rotation: the angle between a line dividing the thorax and the sagittal plane of the vertebra was measured. An increase in rotation suggested progressive asymmetry in the thorax.
- Spinal rotation: the angle between the sagittal plane of the apical vertebra, defined as a line perpendicular to a line drawn between the rib heads, and a line perpendicular to the inferior edge of the ribs, was measured.
CT lung volumes
3D volumetric reconstructions of the lung parenchyma were created using Lung Density Analysis software on the Philips Extended Brilliance Workspace (R4.5; Philips). Figure 3D,3E illustrate a representative volumetric reconstruction of the lungs and trachea. The trachea and main bronchi were excluded from the 3D reconstruction to generate separate volumes for the right and left lungs. A total of 9 volumetric parameters were computed: the left lung volume at end inspiration, right lung volume at end inspiration, total lung volume (TLV) at end inspiration, convex-to-concave lung volume ratio at end inspiration, left lung volume at end expiration, right lung volume at end expiration, TLV at end expiration, convex-to-concave lung volume ratio at end expiration, and total lung tidal volume. The calculation for total lung tidal volume involved subtracting TLV at end expiration from TLV at end inspiration.
PFTs
Pre- and post-HPT PFTs were conducted on all patients in the study. The collected pulmonary function parameters included forced vital capacity (FVC), percent predicted forced vital capacity (FVC%), forced expiratory volume in the first second (FEV1), percent predicted forced expiratory volume in the first second (FEV1%), FEV1/FVC ratio, total lung capacity (TLC), and percent predicted total lung capacity (TLC%). The percent-predicted volumes were calculated based on normative values adjusted for age and arm span. Pulmonary impairment severity was categorized according to the American Thoracic Society guidelines: ‘no’ impairment for FVC% >80% of predicted, ‘mild’ for FVC% ≤80% but >65%, ‘moderate’ for FVC% ≤65% but >50%, and ’severe’ for FVC% ≤50% (12). Obstructive lung disease was defined as an FEV1/FVC ratio below the 5th percentile (13).
Statistical analysis
Statistical analysis was performed using SPSS software version 27.0 (IBM Corp., Armonk, NY, USA). The data were presented as the mean ± standard deviation. The differences between variables before and after HPT were assessed using matched-pair t-tests. The correlation coefficients were calculated to evaluate the associations between traction duration, alterations in lung volume, thoracic anatomical parameters, and changes in PFTs. Pearson’s correlation coefficient was used if the data conformed to a normal distribution, whereas Spearman’s correlation coefficient was used for the data that failed to conform to a normal distribution. A P value <0.05 indicated a statistically significant difference.
Results
In the study, all 24 patients demonstrated right thoracic curves, with the apex between the T7 and T9 vertebrae. The HPT duration varied from 41 to 182 days, with a mean duration of 91.7±37.1 days. Following HPT, there were statistically significant increases in the patient’s arm span, height, and body weight, accompanied by a decrease in BMI. Detailed patient information is presented in Table 1.
Table 1
Variables | Values |
---|---|
Sex | |
Female | 19 |
Male | 5 |
Major curve coronal apex level | |
T7 | 4 |
T8 | 11 |
T9 | 9 |
Duration of HPT (days) | 91.7±37.1 |
Age (years) | |
Pre-traction | 15.8±2.2 |
Post-traction | 16.1±2.2* |
Arm span (cm) | |
Pre-traction | 153.5±13.8 |
Post-traction | 155.7±15.1* |
Height (cm) | |
Pre-traction | 142.2±15.2 |
Post-traction | 154.8±14.8* |
Body weight (kg) | |
Pre-traction | 36.6±9.6 |
Post-traction | 39.9±8.4* |
BMI (kg/m2) | |
Pre-traction | 17.8±1.7 |
Post-traction | 16.6±2.0* |
The data are presented as n and mean ± standard deviation. Repeated measures were analyzed using matched-pair t-tests. A P value <0.05 indicated a statistically significant difference. The asterisk (*) denotes statistical differences. HPT, halo-pelvic traction; BMI, body mass index.
The primary thoracic Cobb angle, kyphosis angle, thoracic height, hemithoracic symmetry ratio, thoracic rotation, and spinal rotation data for pre- and post-HPT are presented in Table 2. Following traction, reductions in the thoracic Cobb angle, thoracic kyphosis, and hemithoracic symmetry ratio were observed, with average decreases of 32.9%, 33.0%, and 18.8%, respectively. Additionally, thoracic height showed an increase of 36.1% after HPT. However, there were no significant changes noted in thoracic rotation and spinal rotation.
Table 2
Thoracic anatomical parameters | Pre-traction (n=24) | Post-traction (n=24) | Average change (% change) | P value | Cohen’s d |
---|---|---|---|---|---|
Major thoracic Cobb angle (°) | 104.0±17.5 | 69.8±18.9 | −34.2 (32.9) | <0.001 | −2.48 |
Thoracic kyphosis (°) | 83.6±37.8 | 55.9±35.4 | −27.6 (33.0) | <0.001 | −1.54 |
Thoracic height (cm) | 18.0±5.3 | 24.5±4.6 | 6.5 (36.1) | <0.001 | 2.37 |
Hemithoracic symmetry ratio | 20.7±18.9 | 16.8±16.3 | −3.9 (18.8) | 0.002 | −0.70 |
Thoracic rotation (°) | 84.0±19.3 | 84.1±19.0 | 0.1 (0.1) | 0.943 | – |
Spinal rotation (°) | 27.2±17.0 | 23.1±12.0 | −4.1 (15.1) | 0.080 | – |
The data are expressed as mean ± standard deviation. All changes were calculated by subtracting pre-traction values from post-traction values. Repeated measures were analyzed using matched-pair t-tests. A P value <0.05 indicated a statistically significant difference.
Following HPT, the mean values of total, left and right lung volumes at end inspiration, total and left lung volumes at end expiration showed significant increases ranging from 18.1% to 25.7% (refer to Table 3). Although right lung volume at end expiration and total lung tidal volume increased, the differences were not statistically significant. Changes in TLV at end inspiration and expiration, and total lung tidal volume before and after HPT are depicted in Figure 4. Furthermore, we employed the convex-to-concave (right to left) lung volume ratio to evaluate lung volume asymmetry. Our findings revealed no significant alterations in either the convex-to-concave lung volume ratios at end inspiration or expiration.
Table 3
Lung volume and its ratio | Pre-traction (n=24) | Post-traction (n=24) | Average change (% change) | P value | Cohen’s d |
---|---|---|---|---|---|
RLVEI (mL) | 1,137.0±487.1 | 1,342.3±490.3 | 205.4 (18.1) | 0.001 | 0.76 |
LLVEI (mL) | 960.3±415.6 | 1,189.2±496.5 | 228.9 (23.8) | 0.002 | 0.70 |
CCLVREI | 1.25±0.39 | 1.30±0.62 | 0.04 | 0.736 | – |
TLVEI (mL) | 2,097.3±867.6 | 2,531.6±857.7 | 434.3 (20.7) | <0.001 | 1.22 |
RLVEE (mL) | 809.9±325.4 | 908.7±339.4 | 98.9 (12.2) | 0.167 | – |
LLVEE (mL) | 658.7±291.6 | 827.9±347.2 | 169.2 (25.7) | 0.008 | 0.59 |
CCLVREE | 1.34±0.48 | 1.20±0.52 | −0.14 | 0.264 | – |
TLVEE (mL) | 1,468.6±589.1 | 1,736.6±611.0 | 268.1 (18.2) | 0.020 | 0.51 |
TLTV (mL) | 628.7±373.2 | 794.9±652.9 | 166.2 (26.4) | 0.115 | – |
The data are expressed as mean ± standard deviation. All changes were calculated by subtracting pre-traction values from post-traction values. Repeated measures were analyzed using matched-pair t-tests. A P value <0.05 indicated a statistically significant difference. HPT, halo-pelvic traction; RLVEI, right lung volume at end inspiration; LLVEI, left lung volume at end inspiration; CCLVREI, convex-to-concave lung volume ratio at end inspiration; TLVEI, total lung volume at end inspiration; RLVEE, right lung volume at end expiration; LLVEE, left lung volume at end expiration; CCLVREE, convex-to-concave lung volume ratio at end expiration; TLVEE, total lung volume at end expiration; TLTV, total lung tidal volume.
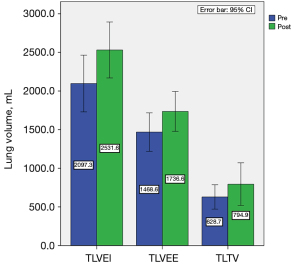
In terms of the results of PFTs, all pulmonary function values, including FVC, FVC%, FEV1, FEV1%, FEV1/FVC, TLC, and TLC% showed significant increases ranging from 6.3% to 24.4% after HPT (refer to Table 4 and Figure 5). Among the patients, 91.7% (22/24) exhibited pulmonary impairment, with 16.7% (4/24) classified as having moderate impairment and 62.5% (15/24) as having severe impairment. Additionally, 6 patients had concomitant obstructive pulmonary abnormalities, whereas 22 patients presented with segmental or lobar bronchial stenosis and atelectasis.
Table 4
Pulmonary function tests | Pre-traction (n=24) | Post-traction (n=24) | Average change (% change) | P value | Cohen’s d |
---|---|---|---|---|---|
FVC (L) | 1.51±0.84 | 1.75±0.66 | 0.24 (15.9) | 0.001 | 0.76 |
FVC% | 45.98±20.15 | 51.91±17.30 | 5.93 (12.9) | 0.013 | 0.55 |
FEV1 (L) | 1.27±0.68 | 1.58±0.56 | 0.31 (24.4) | <0.001 | 1.11 |
FEV1% | 47.92±22.25 | 55.46±17.15 | 7.54 (15.7) | 0.018 | 0.52 |
FEV1/FVC (%) | 85.67±7.83 | 91.11±6.74 | 5.44 (6.3) | 0.002 | 0.73 |
TLC (L) | 2.62±0.95 | 3.02±0.89 | 0.40 (15.3) | 0.001 | 0.83 |
TLC% | 61.12±14.83 | 66.22±12.43 | 5.10 (8.3) | 0.035 | 0.46 |
The data are expressed as mean ± standard deviation. All changes were calculated by subtracting pre-traction values from post-traction values. Repeated measures were analyzed using matched-pair t-tests. A P value <0.05 indicated a statistically significant difference. FVC, forced vital capacity; FVC%, percent predicted forced vital capacity; FEV1, forced expiratory volume in the first second; FEV1%, percent predicted forced expiratory volume in the first second; TLC, total lung capacity; TLC%, percent predicted total lung capacity.
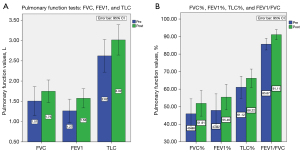
The relationships between the alterations in PFTs and CT, as well as radiographic parameters, were examined. The findings revealed negative correlations between the change in TLC% and hemithoracic symmetry ratio (r=−0.410, P=0.047). Furthermore, a positive correlation was observed between the change in FEV1 and TLV at end expiration (r=0.423, P=0.039). However, no statistically significant associations were found between the changes in PFTs and other CT and radiographic parameters. This study further explored the relationship between traction duration and alterations in radiographic and CT parameters, CT lung volumes, and PFTs. The findings revealed a negative correlation between traction duration and Cobb angle changes (r=−0.688, P<0.001), as well as positive correlations between traction duration and changes in left lung volumes at end inspiration (r=0.518, P=0.010) and expiration (r=0.452, P=0.027) (refer to Figure 6). No correlations were identified for other parameters. Furthermore, the study investigated the association between the primary Cobb angle and changes in PFTs, revealing a positive correlation between the primary Cobb angle and the increase in TLC (r=0.413, P=0.045), with no association observed with other pulmonary function parameters.
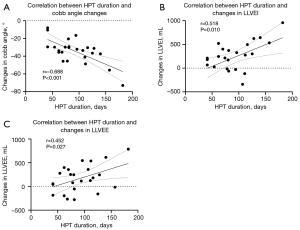
Discussion
Severe AIS often presents with respiratory function impairment, which is associated with thoracic Cobb angles (14,15). Studies (15,16) have shown that the prevalence of moderate to severe pulmonary impairment in preoperative AIS cases ranges from 14% to 28%, significantly increasing to 74% in cases with thoracic curves exceeding 80 degrees. Our study findings align closely with existing literature, revealing that 91.7% (22/24) of patients exhibit pulmonary impairment, with 79.2% (19/24) experiencing moderate to severe pulmonary dysfunction.
In the majority of AIS cases, the primary respiratory dysfunction is restrictive, characterized by equally reduced FEV1 and FVC, while maintaining a normal FEV1/FVC ratio (17). This restriction is primarily linked to chest wall deformity, decreased chest wall compliance, and respiratory muscle weakness (18-20). However, among thoracic AIS patients undergoing surgery, the prevalence of obstructive lung disease ranges from 21% to 39% (21-23). Our study identified that 25% (6/24) of patients exhibited combined obstructive pulmonary abnormalities. Furthermore, 22 patients were diagnosed with segmental or lobar bronchial stenosis and atelectasis. These findings support that ventilatory dysfunction in thoracic AIS is not solely restrictive but often includes an obstructive component, consistent with previous research (24). Moreover, 27.3% (6/22) of patients exhibited improvements in segmental or lobar bronchial stenosis and atelectasis as observed in CT scans (Figure 7). Following HPT, there was an increase in FEV1/FVC ratio. These observed improvements are likely associated with the correction of deformities, particularly the reduction of the hemithoracic symmetry ratio and the primary Cobb angle as shown in the results, leading to the enlargement of the right hemithorax.
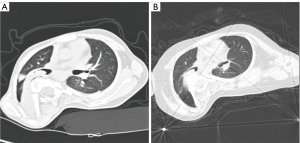
Preoperative HPT has been shown to significantly improve both spinal deformity and pulmonary function, with minor and manageable complications (5,8,9), which aligns with the results of our study. Following traction, there was a reduction in the thoracic Cobb angle, thoracic kyphosis, and hemithoracic symmetry ratio. Additionally, an average increase of 6.5 cm in thoracic height was observed. These outcomes suggest that this intervention effectively addresses deformities in the coronal, sagittal, and axial planes, reduces scoliotic curvature, and elongates the spine. The PFT results demonstrated significant improvements in all parameters, including FVC, FVC%, FEV1, FEV1%, TLC, and TLC%, with enhancements ranging from 8.3% to 24.4% post-HPT. These results are consistent with previous research (8,9). Notably, the observed increase in the FEV1/FVC ratio has not been reported in prior studies.
This study is the first to utilize CT for evaluating changes in lung volume following HPT. Accurate measurement of lung volume is essential for assessing restrictive pulmonary dysfunction (25). CT-based lung volume measurement provides more precise results than conventional PFTs (25,26). The results demonstrated an improvement in CT TLV following traction. These findings were further supported by PFTs, which showed an increase in TLC and TLC% after HPT. A previous study (27) showed that patients diagnosed with AIS exhibit lower right-to-left lung volume ratios compared to age-matched individuals without AIS. Therefore, we assessed lung volume asymmetry by analyzing the convex-to-concave lung volume ratio. Our results indicated no significant changes in the convex-to-concave lung volume ratios at both end inspiration and expiration. Previous studies (28-30) indicated that the ratio of convex-to-concave lung volume remained relatively stable following corrective surgery, consistent with our results, despite the utilization of diverse intervention approaches.
Correlation analysis between changes in PFTs, lung volumes, and thoracic anatomical parameters revealed that only alterations in TLV at end expiration and hemithoracic symmetry ratio were significantly correlated with PFTs. Contrary to previous findings (31), although TLVs increased, these changes showed no correlation with changes in TLC and TLC%. Moreover, variations in thoracic Cobb angle, thoracic kyphosis, and thoracic height did not exhibit direct correlations with changes in PFTs. Additionally, we found no correlation between the primary Cobb angle and the improvement in most PFT parameters. These results demonstrate that despite increases in TLV and spinal deformity, most parameters do not directly impact pulmonary function. We propose that this outcome may be partially attributed to the respiratory training and physical exercises, which enhanced chest wall compliance and respiratory muscle strength. Therefore, the enhancement in PFTs may not solely be influenced by alterations in lung volume and spinal deformity but also by these interventions.
Our study further investigated the influence of traction duration on radiographic and CT parameters, CT lung volumes, and PFTs. The results indicated that extended traction duration was associated with a more substantial improvement in the Cobb angle and an increase in left lung volume. In contrast, no significant association was observed between TLV, right lung volume, and traction duration. These findings imply that as traction duration is prolonged, the correction of scoliotic deformity becomes more pronounced, with a more direct effect on the enhancement of concave (left) lung volume rather than convex (right) lung volume.
Biphasic quantitative thoracic CT has been extensively employed in chronic obstructive pulmonary disease and small airway disease, showcasing its capability to assess pulmonary function (32,33). In this study, lung tidal volume was computed using CT scans at both end inspiration and expiration, assuming it mirrors respiratory movement akin to FVC. Patients were instructed to maintain a consistent lung volume by breath-holding during the scans, and pre-scan breath-holding training was conducted to enhance the precision of CT lung volume measurements. Although there was a rise in lung tidal volume, this difference was not statistically significant, in contrast to the significant increases seen in FVC and FVC%. This finding suggests that lung tidal volume cannot be considered a reliable predictor of pulmonary function, as originally hypothesized.
This study has some limitations. First, biphasic CT scans were used to assess lung volume at both end inspiration and expiration for calculating lung tidal volume. Radiation exposure is a critical concern that must be addressed. In this research, both low-dose unenhanced and enhanced CT scans were employed to evaluate spine and vessel conditions, ensuring precision and safety in surgical procedures rather than for the primary study objective. To minimize radiation exposure, lung volume at end inspiration was measured from enhanced scans, whereas lung volume at end expiration was measured from unenhanced scans. In addition, we utilized low voltage (80–100 keV), automated exposure control, and iterative reconstruction algorithms (iDose4) to minimize patient radiation exposure. Table S1 provides details of the radiation doses for both unenhanced and enhanced CT scans. The total dose is within the recommended American College of Radiology 2022 guidelines for only the unenhanced chest CT (34). However, the routine use of CT scans for lung function assessment is not recommended. Another limitation of this study is that CT lung volume results may be influenced by breathing. Despite conducting pre-scan breath-holding training, lung volume measurements were still susceptible to factors such as breathing patterns and patient compliance. The lack of correlation between the alteration in CT lung tidal volume and PFTs may be partly attributed to this. Quantitative dynamic thoracic magnetic resonance imaging (MRI) could serve as a potential alternative for evaluating dynamic thoracic function and lung volume, particularly in pediatric and adolescent patients. One notable advantage of this method is its capability to capture MRI scans while the patient breathes naturally, without radiation exposure (35,36).
Conclusions
Preoperative HPT significantly improved spinal deformity, TLV, and pulmonary functions. CT lung tidal volume, as the key parameter in biphasic CT, was found to be an unreliable predictor of pulmonary function. The use of biphasic thoracic CT did not demonstrate substantial benefits over monophasic CT and is not recommended for severe AIS.
Acknowledgments
We thank Xiaodi Zhang for editing the manuscript and for editorial assistance.
Funding: This work was supported by
Footnote
Reporting Checklist: The authors have completed the STROBE reporting checklist. Available at https://qims.amegroups.com/article/view/10.21037/qims-24-568/rc
Conflicts of Interest: All authors have completed the ICMJE uniform disclosure form (available at https://qims.amegroups.com/article/view/10.21037/qims-24-568/coif). All authors report that this work was supported by the Research Project of Chengdu Medical Association (grant No. 2021117). The authors have no other conflicts of interest to declare.
Ethical Statement: The authors are accountable for all aspects of the work in ensuring that questions related to the accuracy or integrity of any part of the work are appropriately investigated and resolved. The study was conducted in accordance with the Declaration of Helsinki (as revised in 2013). The study was approved by the Ethics Committee of the Third People’s Hospital of Chengdu (No. 2024-S-105) and the requirement for individual consent for this retrospective analysis was waived.
Open Access Statement: This is an Open Access article distributed in accordance with the Creative Commons Attribution-NonCommercial-NoDerivs 4.0 International License (CC BY-NC-ND 4.0), which permits the non-commercial replication and distribution of the article with the strict proviso that no changes or edits are made and the original work is properly cited (including links to both the formal publication through the relevant DOI and the license). See: https://creativecommons.org/licenses/by-nc-nd/4.0/.
References
- Deng M, Chen Q, Deng Q, Shi L, Cheng CCN, Yeung KH, Zhang R, Yu WPF, Lam TP, Cheng JCY, Chu WCW. Statistical changes of lung morphology in patients with adolescent idiopathic scoliosis after spinal fusion surgery-a prospective nonrandomized study based on low-dose biplanar X-ray imaging. Quant Imaging Med Surg 2022;12:3325-39. [Crossref] [PubMed]
- Xu Y, Liu C, Li D, Cai Y, Hu Z, Zhu Z, Qiu Y, Liu Z. Cosmetic evaluation in type II congenital scoliosis with long-spanned curve: a case-matched comparison with adolescent idiopathic scoliosis. Quant Imaging Med Surg 2023;13:6164-75. [Crossref] [PubMed]
- Xia L, Li P, Wang D, Bao D, Xu J. Spinal osteotomy techniques in management of severe pediatric spinal deformity and analysis of postoperative complications. Spine (Phila Pa 1976) 2015;40:E286-92. [Crossref] [PubMed]
- Teixeira da Silva LE, de Barros AG, de Azevedo GB. Management of severe and rigid idiopathic scoliosis. Eur J Orthop Surg Traumatol 2015;25:S7-12. [Crossref] [PubMed]
- O'Brien JP, Yau AC, Hodgson AR. Halo pelvic traction: a technic for severe spinal deformities. Clin Orthop Relat Res 1973;179-90. [Crossref] [PubMed]
- O'Brien JP, Yau AC, Smith TK, Hodgson AR. Halo pelvic traction. A preliminary report on a method of external skeletal fixation for correcting deformities and maintaining fixation of the spine. J Bone Joint Surg Br 1971;53:217-29.
- Sun Y, Zhang Y, Ma H, Tan M, Zhang Z. Halo-pelvic traction in the treatment of severe scoliosis: a meta-analysis. Eur Spine J 2023;32:874-82. [Crossref] [PubMed]
- Zhao D, Wang F, Hu Z, Zhong R, Liang Y. Pulmonary and clinical outcomes of patients with severe rigid scoliosis and type I respiratory failure treated with halo-pelvic distraction. J Orthop Surg Res 2023;18:710. [Crossref] [PubMed]
- Qi L, Xu B, Li C, Wang Y. Clinical efficacy of short-term pre-operative halo-pelvic traction in the treatment of severe spinal deformities complicated with respiratory dysfunction. BMC Musculoskelet Disord 2020;21:665. [Crossref] [PubMed]
- Iwasawa T, Matsushita S, Hirayama M, Baba T, Ogura T. Quantitative Analysis for Lung Disease on Thin-Section CT. Diagnostics (Basel) 2023;13:2988. [Crossref] [PubMed]
- Campbell RM Jr, Smith MD, Mayes TC, Mangos JA, Willey-Courand DB, Kose N, Pinero RF, Alder ME, Duong HL, Surber JL. The characteristics of thoracic insufficiency syndrome associated with fused ribs and congenital scoliosis. J Bone Joint Surg Am 2003;85:399-408. [Crossref] [PubMed]
- Quanjer PH, Tammeling GJ, Cotes JE, Pedersen OF, Peslin R, Yernault JC. Lung volumes and forced ventilatory flows. Report Working Party Standardization of Lung Function Tests, European Community for Steel and Coal. Official Statement of the European Respiratory Society. Eur Respir J Suppl 1993;16:5-40.
- Quanjer PH, Stanojevic S, Cole TJ, Baur X, Hall GL, Culver BH, Enright PL, Hankinson JL, Ip MS, Zheng J, Stocks JERS Global Lung Function Initiative. Multi-ethnic reference values for spirometry for the 3-95-yr age range: the global lung function 2012 equations. Eur Respir J 2012;40:1324-43. [Crossref] [PubMed]
- Kan MMP, Negrini S, Di Felice F, Cheung JPY, Donzelli S, Zaina F, Samartzis D, Cheung ETC, Wong AYL. Is impaired lung function related to spinal deformities in patients with adolescent idiopathic scoliosis? A systematic review and meta-analysis-SOSORT 2019 award paper. Eur Spine J 2023;32:118-39. [Crossref] [PubMed]
- Newton PO, Faro FD, Gollogly S, Betz RR, Lenke LG, Lowe TG. Results of preoperative pulmonary function testing of adolescents with idiopathic scoliosis. A study of six hundred and thirty-one patients. J Bone Joint Surg Am 2005;87:1937-46. [Crossref] [PubMed]
- Johnston CE, Richards BS, Sucato DJ, Bridwell KH, Lenke LG, Erickson MSpinal Deformity Study Group. Correlation of preoperative deformity magnitude and pulmonary function tests in adolescent idiopathic scoliosis. Spine (Phila Pa 1976) 2011;36:1096-102. [Crossref] [PubMed]
- Lung function testing: selection of reference values and interpretative strategies. American Thoracic Society. Am Rev Respir Dis 1991;144:1202-18. [Crossref] [PubMed]
- Yaszay B, Bastrom TP, Bartley CE, Parent S, Newton PO. The effects of the three-dimensional deformity of adolescent idiopathic scoliosis on pulmonary function. Eur Spine J 2017;26:1658-64. [Crossref] [PubMed]
- Kotani T, Minami S, Takahashi K, Isobe K, Nakata Y, Takaso M, Inoue M, Maruta T, Akazawa T, Ueda T, Moriya H. An analysis of chest wall and diaphragm motions in patients with idiopathic scoliosis using dynamic breathing MRI. Spine (Phila Pa 1976) 2004;29:298-302. [Crossref] [PubMed]
- Martínez-Llorens J, Ramírez M, Colomina MJ, Bagó J, Molina A, Cáceres E, Gea J. Muscle dysfunction and exercise limitation in adolescent idiopathic scoliosis. Eur Respir J 2010;36:393-400. [Crossref] [PubMed]
- Farrell J, Garrido E. Predicting preoperative pulmonary function in patients with thoracic adolescent idiopathic scoliosis from spinal and thoracic radiographic parameters. Eur Spine J 2021;30:634-44. [Crossref] [PubMed]
- McPhail GL, Ehsan Z, Howells SA, Boesch RP, Fenchel MC, Szczesniak R, Jain V, Agabegi S, Sturm P, Wall E, Redding GJ. Obstructive lung disease in children with idiopathic scoliosis. J Pediatr 2015;166:1018-21. [Crossref] [PubMed]
- Wang J, Zhou B, Yang X, Zhou C, Ling T, Hu B, Song Y, Liu L. Computed tomography-based bronchial tree three-dimensional reconstruction and airway resistance evaluation in adolescent idiopathic scoliosis. Eur Spine J 2020;29:1981-92. [Crossref] [PubMed]
- Farrell J, Garrido E, Vavruch L, Schlösser TPC. Thoracic Morphology and Bronchial Narrowing Are Related to Pulmonary Function in Adolescent Idiopathic Scoliosis. J Bone Joint Surg Am 2021;103:2014-23. [Crossref] [PubMed]
- Pellegrino R, Viegi G, Brusasco V, Crapo RO, Burgos F, Casaburi R, Coates A, van der Grinten CP, Gustafsson P, Hankinson J, Jensen R, Johnson DC, MacIntyre N, McKay R, Miller MR, Navajas D, Pedersen OF, Wanger J. Interpretative strategies for lung function tests. Eur Respir J 2005;26:948-68. [Crossref] [PubMed]
- Aaron SD, Dales RE, Cardinal P. How accurate is spirometry at predicting restrictive pulmonary impairment? Chest 1999;115:869-73. [Crossref] [PubMed]
- Adam CJ, Cargill SC, Askin GN. Computed tomographic-based volumetric reconstruction of the pulmonary system in scoliosis: trends in lung volume and lung volume asymmetry with spinal curve severity. J Pediatr Orthop 2007;27:677-81. [Crossref] [PubMed]
- Yu CG, Grant CA, Izatt MT, Labrom RD, Askin GN, Adam CJ, Little JP. Change in Lung Volume Following Thoracoscopic Anterior Spinal Fusion Surgery: A 3-Dimensional Computed Tomography Investigation. Spine (Phila Pa 1976) 2017;42:909-16. [Crossref] [PubMed]
- Fu J, Liu C, Zhang YG, Zheng GQ, Zhang GY, Song K, Tang XY, Wang Y. Three-dimensional computed tomography for assessing lung morphology in adolescent idiopathic scoliosis following posterior spinal fusion surgery. Orthop Surg 2015;7:43-9. [Crossref] [PubMed]
- Sarwahi V, Sugarman EP, Wollowick AL, Amaral TD, Harmon ED, Thornhill B. Scoliosis surgery in patients with adolescent idiopathic scoliosis does not alter lung volume: a 3-dimensional computed tomography-based study. Spine (Phila Pa 1976) 2014;39:E399-405. [Crossref] [PubMed]
- Ledonio CG, Rosenstein BE, Johnston CE, Regelmann WE, Nuckley DJ, Polly DW Jr. Pulmonary function tests correlated with thoracic volumes in adolescent idiopathic scoliosis. J Orthop Res 2017;35:175-82. [Crossref] [PubMed]
- Kirby M, Yin Y, Tschirren J, Tan WC, Leipsic J, Hague CJ, Bourbeau J, Sin DD, Hogg JC, Coxson HO. CanCOLD Collaborative Research Group and the Canadian Respiratory Research Network. A Novel Method of Estimating Small Airway Disease Using Inspiratory-to-Expiratory Computed Tomography. Respiration 2017;94:336-45. [Crossref] [PubMed]
- Matsuo Y, Ogawa E, Tsunoda Y, Yamazaki A, Kawashima S, Uchida Y, Nakagawa H, Kinose D, Yamaguchi M, Nakano Y. Inspiratory and Expiratory Computed Tomography Imaging Clusters Reflect Functional Characteristics in Chronic Obstructive Pulmonary Disease. Int J Chron Obstruct Pulmon Dis 2023;18:1047-55. [Crossref] [PubMed]
- Kanal KM, Butler PF, Chatfield MB, Wells J, Samei E, Simanowith M, Golden D, Gress DA, Burleson J, Sensakovic WF, Strauss KJ, Frush D U.S.. Diagnostic Reference Levels and Achievable Doses for 10 Pediatric CT Examinations. Radiology 2022;302:E6. [Crossref] [PubMed]
- Tong Y, Udupa JK, McDonough JM, Wileyto EP, Capraro A, Wu C, Ho S, Galagedera N, Talwar D, Mayer OH, Torigian DA, Campbell RM. Quantitative Dynamic Thoracic MRI: Application to Thoracic Insufficiency Syndrome in Pediatric Patients. Radiology 2019;292:206-13. [Crossref] [PubMed]
- Udupa JK, Tong Y, Capraro A, McDonough JM, Mayer OH, Ho S, Wileyto P, Torigian DA, Campbell RM Jr. Understanding Respiratory Restrictions as a Function of the Scoliotic Spinal Curve in Thoracic Insufficiency Syndrome: A 4D Dynamic MR Imaging Study. J Pediatr Orthop 2020;40:183-9. [Crossref] [PubMed]