Hematoma surface irregularity predicts postoperative rebleeding and poor drainage in patients with spontaneous intracerebral hemorrhage following minimally invasive surgery: a retrospective cohort study at a high-volume stroke center
Introduction
Intracerebral hemorrhage (ICH) accounts for about 10% of all strokes worldwide (1), and has a 1-month mortality rate of up to 40% (2). Despite the acknowledged pathophysiologic benefits of hemostasis and clot removal in the treatment of ICH, effective medical and surgical treatments for ICH are lacking (3,4). Minimally invasive surgery (MIS) for hematoma evacuation has shown promising results in recent years (5,6). However, in the Minimally Invasive Surgery with Thrombolysis in Intracerebral Hemorrhage Evacuation (MISTIE III) study (7), MIS failed to achieve a significant functional improvement in comparison with the standard medical treatment. Among the risk factors that may influence the outcome of MIS (8), early intracerebral postoperative rebleeding is a great challenge for subsequent management as well as a devastating neurologic complication with subsequent higher morbidity and mortality than those non-rebleeding patients (9). Previous studies have reported that irregularly shaped hematoma might be an independent predictor of postoperative rebleeding (10,11). However, the interpretation of irregular hematoma is subjective, and stringent morphological criteria are lacking. Thus quantitative and objective methods need to be established to measure hematoma irregularity. The three-dimensional (3D) Slicer (version 4.10.1, www.slicer.org, Harvard University, USA) is widely used in ICH research on hematoma volume modeling (12) and MIS puncture trace selection (13). It can also be used to describe hematoma irregularity.
Surgical performance also plays a key role in determining the postoperative functional outcomes of patients (14,15). Research suggests that irregularly shaped hematoma are associated with poor drainage (which is often defined as a residual volume >15 mL) (15). Using a mathematically defined surface regularity metric, this study specifically evaluated the association between surface regularity, and postoperative rebleeding and poor drainage in ICH patients following MIS. We present this article in accordance with the STROCSS reporting checklist (available at https://qims.amegroups.com/article/view/10.21037/qims-24-1105/rc).
Methods
Study population
A retrospective analysis of the data of patients with ICH following MIS from January 2021 to September 2022 at the Beijing Tiantan Hospital was conducted. To be eligible for inclusion in this study, the patients had to meet the following inclusion criteria: (I) be aged 18 to 80 years; (II) have first supratentorial ICH confirmed by non-contrast computed tomography (NCCT); (III) have a symptom onset within 24 hours and a hematoma volume ≥20 mL (3); (IV) have a Glasgow Coma Scale (GCS) score of 14 or less, or a National Institutes of Health Stroke Scale (NIHSS) score of 6 or higher, or a modified Rankin Scale (mRS) score of 0 or 1 before the bleed. Patients were excluded from the study if they met any of the following exclusion criteria: (I) had infratentorial ICH; (II) had ICH caused by trauma, cerebrovascular malformation, Moyamoya disease, neoplastic lesion, or aneurysm-related ICH; (III) have anticoagulant-associated ICH or a disturbance in blood coagulation, such as thrombocytopenia and hepatitis; (IV) have a primary interventricular hemorrhage; (V) require other surgical interventions, such as craniotomy; and/or (VI) have missing outcome data or medical records.
The study was conducted in accordance with the Declaration of Helsinki (as revised in 2013). The study was approved by the Ethics Board of Beijing Tiantan Hospital (No. KY2021-025-01), and informed consent was obtained from all the patients or their relatives.
Clinical variables
The baseline demographic characteristics of the patients were collected, including age, gender, and medical history (hypertension, diabetes mellitus, antiplatelet, and anticoagulant therapy before ICH onset). The GCS and NIHSS scores were evaluated at admission. Hematologic tests (glucose level, leukocyte and platelet count, brain natriuretic peptide, and D-dimer), radiologic characteristics [onset to baseline CT time, hematoma location, and presence of intraventricular hemorrhage (IVH)], and MIS procedure variables (onset to surgery time, duct indwelling depth, aspiration volume and presence of duct misplaced) were recorded.
Image acquisition and interpretation
The baseline, preoperative, and postoperative NCCT examinations were completed on a 16-slice CT scanner (Brilliance iCT; Philips Health-care, Cleveland, OH, USA) using the following helical scan parameters: 120 kVp; 310 mAs; 1 mm thickness; and a 512×512 image size. The follow-up CT examinations were performed 6 hours or more after the baseline CT to access the clot volume. A hematoma expansion (HE) was defined as an increase in the hematoma volume of 6 ml or >33% in the follow-up CT result compared with the baseline CT result (16). If HE was confirmed, an additional CT examination was completed afterwards to rule out active bleeding (which was usually defined as an expanded clot <5 mL compared with that of the adjacent previous CT result) preoperatively. The preoperative CT examination was performed within 1 hour of the MIS for surgical puncture positioning. The hematoma was segmented and reconstructed using original Digital Imaging and Communication in Medicine (DICOM) data in 3D Slicer software (version 4.10.1, www.slicer.org, Harvard University, USA). The hematoma volume and surface area were calculated by 3D modeling without smoothing processing. The SRI was calculated by the formula in the preoperative navigation CT (17). The score ranges from 0 (which indicates a “fractal” hematoma with very irregular surfaces) and 1 (which indicates a spherical hematoma). The SRI was calculated using the following formula:
Surgical procedures
In our study, the procedure of MIS was similar to that used in the MISTIE III study. A diagram of the body surface projection of the hematoma area on the scalp was confirmed according to the preoperative CT scan, and the puncture point on the patient’s scalp and the ideal depth of the duct was then determined. Following the administration of propofol through an intravenous infusion to induce the anesthetic state, and the stabilization of systolic blood pressure (to <140 mmHg), a 3-mm-diameter needle (Type 12F, Godsend Inc., Shandong, China) was used to puncture the skull with the driving force of an electrical drill. Subsequently, a plastic drill was employed to penetrate the dura mater. Next, the drill was extracted and replaced by a tip-blunt plastic duct with a metallic needle core. The puncture duct set was inserted slightly into the hematoma. Removing the metallic needle core, the liquid part of the hematoma was aspirated with a 20-mL syringe, and aspiration was stopped when the first resistance was encountered (usually at the removal of 30–50% of the total amount of the hematoma). The duct guard was connected to a drainage bag through a plastic duct for drainage. Next, 10,000 units of urokinase (diluted in 2 mL of normal saline) was slowly injected every 8 hours into the residual hematoma to dissolve the solid component of the hematoma. Postoperative follow-up CT scans were performed on the second and third days after surgery. Duct misplacement was defined as the absence of the endpoint of the indwelling duct in the central one-third portion of the 3D model of the hematoma. Any misplaced ducts were replaced following the MISTIE procedures (15) and reevaluated in the following CT scans. The duct removal standard consisted of the following criteria: a decrease in clot size of <15 mL; the administration of nine doses of urokinase; and other circumstances determined by a senior physician. The residual hematoma volume was calculated based on a CT scan performed within 1 hour of the duct eradication.
Outcomes
Postoperative rebleeding was defined as the reappearance of the hematoma or hyper-density in the hematoma region on any follow-up CT scan postoperatively. Rebleeding was defined as an increase in the hematoma volume of 6 mL or >33% compared to any previous postoperative CT result. As per the MISTIE III definition, poor drainage was defined as a residual hematoma volume of >15 mL in the last CT scan before the duct eradication (7).
Statistical analysis
SAS software was used for the statistical analysis (version 9.4; SAS Institute, Cary, NC, USA). All patients were divided into groups according to the rebleeding and poor drainage definitions (Table 1). The continuous variables are expressed as the median [interquartile range (IQR)], or mean ± standard deviation (SD), and were evaluated by the Shapiro-Wilk test, and compared using the Mann-Whitney or t-test, respectively. The chi-square test was used to compare the categorical variables, which are expressed as the number (proportion). Variables with P values <0.05 from the comparison of the baseline characteristics and other selected variables were included in the multivariate logistics regression. Odds ratios (ORs) and 95% confidence intervals (CIs) were calculated for each group. A receiver operating characteristic (ROC) curve analysis was performed to assess the value of the SRI in predicting rebleeding and poor drainage. Youden’s index was used to identify the optimal SRI cut-off value to predict postoperative rebleeding and poor drainage. Results with P values <0.05 (two sided) were considered statistically significant.
Table 1
Variables | All (N=151) | Rebleeding (N=28) | Non-rebleeding (N=123) | P value | Poor drainage (N=75) | Good drainage (N=76) | P value |
---|---|---|---|---|---|---|---|
Male | 112 (74.2) | 22 (78.6) | 90 (73.2) | 0.556 | 57 (76.0) | 55 (72.4) | 0.610 |
Age (years) | 56±14 | 57±13 | 56±15 | 0.568 | 59±14 | 53±14 | 0.007 |
Previous hypertension | 111 (73.5) | 23 (82.1) | 88 (71.5) | 0.251 | 57 (76.0) | 54 (71.1) | 0.491 |
Previous diabetes | 21 (13.9) | 6 (21.4) | 15 (12.2) | 0.203 | 12 (16.0) | 9 (11.8) | 0.460 |
Previous CHD | 9 (6.0) | 1 (3.6) | 8 (6.5) | 0.554 | 5 (6.7) | 4 (5.3) | 0.716 |
Antiplatelet | 4 (2.7) | 1 (3.6) | 3 (2.4) | 0.736 | 3 (4.0) | 1 (1.3) | 0.304 |
GCS | 10 [7, 13] | 12 [9, 12] | 10 [7, 13] | 0.331 | 10 [7, 12] | 12 [7, 15] | 0.263 |
NIHSS | 18 [14, 28] | 18 [14, 25] | 18 [14, 28] | 0.652 | 18 [14, 28] | 18 [14, 26] | 0.612 |
Baseline CT HV (mL) | 36.4 [26.9, 53.6] | 35.5 [26.1, 62.0] | 36.4 [27.0, 50.6] | 0.712 | 49.1 [30.8, 66.4] | 31.5 [25.3, 43.0] | <0.001 |
Onset to baseline CT (hours) | 8.0 [4.5, 16.0] | 7.5 [4.0, 9.0] | 8.0 [4.5, 17.0] | 0.225 | 7.2 [4.4, 12.0] | 9.0 [4.5, 17.0] | 0.170 |
CTA spot sign | 24/132 (18.2%) | 3/22 (13.6%) | 21/110 (19.1%) | 0.545 | 12/65 (18.5%) | 10/67 (15.0%) | 0.586 |
Onset to CTA (hours) | 9.1 [4.5, 22.2] | 8.4 [5.3, 16.8] | 10.7 [3.9, 22.6] | 0.948 | 8.5 [4.6, 17.2] | 11.5 [3.9, 24.3] | 0.533 |
HE | 33 (21.9) | 6 (21.4) | 27 (22.0) | 0.952 | 14 (18.7) | 19 (25.0) | 0.346 |
Lobar hematoma | 28 (18.5) | 5 (17.9) | 23 (18.7) | 0.918 | 19 (25.3) | 9 (11.8) | 0.033 |
IVH | 55 (36.4) | 15 (53.6) | 40 (32.5) | 0.037 | 40 (53.3) | 15 (19.7) | <0.001 |
Na (mmol/L) | 137.7 [136.2, 139.5] |
138.0 [135.2, 139.1] |
137.6 [136.2, 139.6] |
0.937 | 137.5 [135.4, 139.1] |
138.1 [136.4, 140.0] |
0.107 |
K (mmol/L) | 3.8 [3.4, 4.1] | 3.6 [3.2, 4.1] | 3.8 [3.5, 4.1] | 0.376 | 3.8 [3.4, 4.1] | 3.8 [3.4, 4.1] | 0.574 |
Glucose (mmol/L) | 7.6 [6.7, 9.4] | 7.8 [7.0, 10.1] | 7.6 [6.5, 9.2] | 0.248 | 8.0 [6.9, 9.6] | 7.5 [6.4, 9.0] | 0.174 |
eGFR (mL/min∙1.73 m2) | 105.3±22.9 | 109.5±21.7 | 104.4±23.2 | 0.286 | 104.7±22.3 | 105.9±23.6 | 0.757 |
Creatinine (mg/dL) | 67.6 [57.5, 80.1] | 68.0 [53.7, 73.0] | 67.3 [57.6, 80.6] | 0.462 | 66.3 [59.0, 76.2] | 68.1 [55.6, 80.6] | 0.957 |
ALT (U/L) | 22.3 [14.0, 36.9] | 23.7 [14.3, 43.9] | 22.0 [14.0, 33.9] | 0.448 | 25.0 [13.6, 41.0] | 20.9 [14.7, 33.7] | 0.789 |
AST (U/L) | 22.8 [19.0, 29.0] | 25.0 [19.5, 33.7] | 22.1 [19.0, 29.0] | 0.212 | 23.2 [18.9, 29.9] | 22.3 [19.0, 28.4] | 0.575 |
Total protein (g/L) | 73.3±5.5 | 72.7±4.3 | 72.2±5.7 | 0.640 | 71.8±5.7 | 72.8 ±5.2 | 0.238 |
D-dimer (mg/L) | 0.5 [0.3, 0.8] | 0.5 [0.3, 1.1] | 0.5 [0.3, 0.7] | 0.839 | 0.5 [0.3, 0.9] | 0.5 [0.4, 0.7] | 0.540 |
BNP (ng/L) | 47.7 [22.0, 103.3] | 34.3 [22.1, 79.0] | 47.9 [22.0, 110.3] | 0.266 | 47.7 [22.6, 113.0] | 47.3 [21.3, 99.3] | 0.479 |
Leukocyte count (109/L) | 11.43±3.55 | 11.16±4.33 | 11.48±3.36 | 0.666 | 11.77±4.03 | 11.09±2.99 | 0.243 |
Erythrocyte count (109/L) | 4.72±0.62 | 4.90±0.76 | 4.68±0.57 | 0.085 | 4.74±0.65 | 4.70±0.58 | 0.662 |
Platelet count (109/L) | 219.34±61.75 | 220.64±62.62 | 219.04±61.81 | 0.902 | 219.31±65.35 | 219.37±58.42 | 0.995 |
Hemoglobin (g/L) | 147.07±15.68 | 150.18±18.02 | 146.36±15.09 | 0.246 | 148.04±16.47 | 146.11±14.91 | 0.450 |
SBP (mmHg) | 171 [157, 193] | 176 [157, 193] | 170 [157, 195] | 0.723 | 172 [156, 188] | 171 [157, 202] | 0.231 |
Onset to surgery (days) | 2.07 [1.57, 2.73] | 2.05 [1.69, 2.68] | 2.08 [1.55, 2.72] | 0.862 | 2.02 [1.64, 2.60] | 2.11 [1.51, 2.74] | 0.713 |
Preoperative HV (mL) | 40.1 [30.8, 55.0] | 41.5 [32.8, 62.0] | 40.0 [30.8, 53.6] | 0.372 | 49.1 [33.6, 66.4] | 33.4 [27.8, 45.6] | <0.001 |
Preoperative hematoma surface area (cm2) | 96.5 [69.7, 132.9] | 131.5 [87.5, 169.9] | 88.5 [66.6, 127.1] | 0.003 | 125.6 [89.0, 161.1] | 73.9 [60.8, 100.6] | <0.001 |
Preoperative hematoma SRI | 49.22±16.57 | 37.37±11.48 | 51.91±16.40 | <0.001 | 40.63±12.86 | 57.68±15.48 | <0.001 |
Indwelling time (days) | 3.1 [3.0, 3.5] | 3.1 [3.0, 3.9] | 3.1 [3.0, 3.5] | 0.845 | 3.2 [3.0,4.0] | 3.1 [3.0, 3.5] | 0.198 |
Indwelling depth (cm) | 6.5 [6.0, 6.5] | 6.5 [6.0, 6.5] | 6.5 [6.0, 6.5] | 0.856 | 6.2 [5.8, 6.5] | 6.5 [6.0, 6.5] | 0.172 |
Indwelling volume (mL) | 20.0 [10.0, 25.0] | 20.0 [11.3, 25.0] | 18.0 [10.0, 25.0] | 0.570 | 20.0 [15.0, 30.0] | 15.0 [10.0, 20.0] | <0.001 |
Misplaced | 26 (17.22) | 3 (10.71) | 23 (18.70) | 0.312 | 14 (18.67) | 12 (15.79) | 0.640 |
Values are reported as mean ± SD, median [IQR], or n (%). CHD, coronary heart disease; GCS, Glasgow Coma Scale; NIHSS, National Institutes of Health Stroke Scale; CT, computed tomography; HV, hematoma volume; CTA, computed tomography angiography; HE, hematoma expansion; IVH, intraventricular hemorrhage; eGFR, estimated glomerular filtration rate; ALT, alanine aminotransferase; AST, aspartate aminotransferase; BNP, brain natriuretic peptide; SBP, systolic blood pressure; SRI, surface regularity index; SD, standard deviation; IQR, interquartile range.
Results
Participants
From January 2021 to September 2022, 151 patients who met the study’s inclusion criteria were identified (Figure 1). The mean age of the patients was 56 years (56±14 years). Among the 151 patients, 112 were male. The median of the preoperative CT hematoma volume was 40.1 mL (IQR, 30.8 to 55.0 mL), the median surface area was 96.5 cm2 (IQR, 69.7 to 132.9 cm2), and the mean SRI was 49.22 (49.22±16.57). The median time from onset to surgery was 2.07 days (IQR, 1.57 to 2.73 days). Demographic and clinical information for the study patients are shown in Table 1.
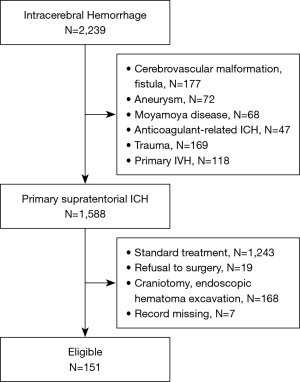
Preoperative CT surface regularity and outcomes
Based on our definition, 28 patients (18.5%) experienced postoperative rebleeding. The patients with rebleeding had a larger preoperative hematoma surface area (131.47 versus 88.53 cm2, P=0.003), a higher prevalence of IVH (53.6% versus 32.5%, P=0.037), and a greater degree of irregularity in the hematoma (hematoma SRI: 37.3 versus 51.9, P<0.001) (Figure 2). Thus, the SRI was able to independently predict rebleeding (OR: 0.926, 95% CI: 0.891–0.963) after adjusting for the following variables: age, sex, hematoma position, time from onset to surgery, the preoperative hematoma volume, indwelling duct lasting time and depth, aspiration volume, the percentage of IVH, and misplaced ducts. The SRI predicted the postoperative rebleeding with a satisfactory discrimination; the area under the curve (AUC) of the ROC curve was 0.765 (95% CI: 0.671–0.858, P<0.001), and the cut-off point, sensitivity, and specificity were 44.980, 66.7%, 82.1%, respectively (Figure 3).
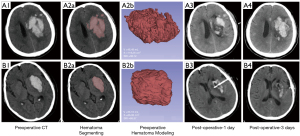
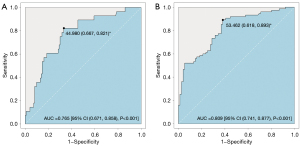
A total of 75 patients (49.7%) experienced poor drainage, which was defined as a residual volume >15 mL. The patients in the poor drainage group were older (59 versus 53 years, P=0.007), had a larger baseline hematoma volume (49.1 versus 31.5 mL, P<0.001) and preoperative hematoma volume (49.1 versus 33.4 mL, P<0.001), a larger preoperative hematoma surface area (125.6 versus 73.9 cm2, P<0.001), a higher degree of irregularity (40.63 versus 57.68, P<0.001), and a higher prevalence of lobar hematoma [19 (25.3%) versus 9 (11.8%), P=0.033] and IVH [40 (53.3) versus 15 (19.7), P<0.001]. The SRI (OR: 0.912, 95% CI: 0.882–0.944, P=0.025) and IVH (OR: 3.729, 95% CI: 1.550–8.972, P<0.001) independently predicted poor drainage after adjusting for the 10 variables mentioned above. The AUC of the SRI for poor drainage was 0.809 (95% CI: 0.741–0.877, P<0.001), and the cut-off point, sensitivity, and specificity were 53.462, 61.8%, 89.3%, respectively (Table 2 and Figure 3).
Table 2
Variables | OR (95% CI) | P value |
---|---|---|
Rebleeding | ||
SRI | 0.926 (0.891, 0.963) | <0.001 |
Poor drainage | ||
SRI | 0.912 (0.882, 0.944) | 0.025 |
IVH | 3.729 (1.550, 8.972) | <0.001 |
Rebleeding and poor drainage all adjusted for 10 variables (age, sex, baseline hematoma volume, hematoma position, time from onset to surgery, indwelling duct lasting time, indwelling duct depth, aspiration volume, and the presence of IVH and misplaced ducts). SRI, surface regularity index; IVH, intraventricular hemorrhage; OR, odds ratio; CI, confidence interval.
Discussion
In this novel study, we explored whether postoperative rebleeding and poor drainage following MIS could be predicted based on the assessment of hematoma shape via 3D Slicer software. We found that the SRI independently predicted postoperative rebleeding and poor drainage in patients following MIS. Thus, the SRI could be used as a precise screening tool for patients who may benefit from MIS.
The precise characterization of hematoma shape in patients with ICH has been highly debated in recent years. Several studies have reported relationships between different classifications of hematoma shape and outcomes. Fujii et al. initially sought to describe hematoma shape using three categories, and classified hematoma as either regular or irregular (18). Barras et al. used a 5-point categorical scale to assess the density and shape of hematoma, classifying them into regular and irregular groups (19). These studies consistently revealed that the irregularity of hematoma was a predictor of HE. Further, the satellite sign (20) and island sign (21), which are characterized of hematoma with very uneven margins, can also serve as predictors of HE with acceptable discriminations (22). However, these signs require subjective and laborious identification that mainly relies on the experience of neurologists, which has led to inevitable interrater deviations and a lack of stringent morphological criteria. Further, the restricted categorizations of irregular shapes fail to adequately illustrate the distinctions among various irregular hematoma.
In a comprehensive study of major ICH surgery trials, the Tada formula (ABC/2) was inadequate in accurately describing irregular, lobar, and big hematoma (23,24). We used 3D Slicer software to quantify the volume and surface area of the hematoma. This software accurately determined the number of pixels that constituted a hematoma without making any assumptions other than those about its shape (25). This precise and objective calculation provided a basis for quantifying the irregularity of the hematoma. Notably, we adopted 1-mm thickness head CT for the segmentation, which differs from the regular approach of 5-mm CT for hematoma modeling adopted in most studies. The model derived from 1-mm CT prevents the artificial enlargement of the hematoma surface area, and the resulting overestimation of hematoma irregularity, and ensures a closer description to its actual state.
Hematoma irregularity was reported to independently predict MIS postoperative rebleeding (10,11). The exact mechanism underlying the association between the observed increase in irregularity and HE (in this study, postoperative rebleeding specifically) remains unclear. Fisher’s domino model proposes that hematoma growth is caused by the shearing of blood vessels around the initial site of hemorrhage, which in turn contributes to secondary bleedings (26). Greenberg et al. mathematically simulated HE, and showed that HE led to hematoma irregularity in macrobleeds (27). Additionally, we also observed that lobar hematoma had greater irregularity than deep hematoma (SRI: lobar 0.41 versus deep 0.51, P=0.004). It is reasonable to assume that the less rigid architecture of the lobes facilitates the expansion of hematoma and the formation of irregular shapes.
Notably, previous studies have shown that the spot sign is an independent predictor of rebleeding following MIS (28) and endoscopic surgery (29). Unfortunately, we did not observe similar results in our study. The predictive performance declined as the time from onset to computed tomography angiography (CTA) increased (30), and our median time window for CTA was 9.1 hours, which may explain this result. Additionally, the low accessibility of CTA may limit the application of the spot sign in predicting rebleeding.
In our study, 50.3% (76/151) of the patients achieved the surgery aim (i.e., a residual clot ≤15 mL), which is similar to the rate of 58% reported in the MISTIE III study (7). The residual volume and evacuation rate were associated with the functional outcomes and mortality rates (15,31); ICH removal to ≤15 mL (or ≥70%) has a greater likelihood of achieving a mRS score 0 to 3 in 1 year (14). Irregularly shaped hematoma are also strongly associated with less efficient ICH removal (15). Irregular hematoma expands dispersedly, resulting a less efficient drainage. The higher rates of poor drainage in lobar hematoma (a relatively more irregular group) may explain our assumption that a more irregular hematoma results in poor drainage. The rate of poor drainage was 67.9% (19/28) in lobar ICH compared to 45.5% (56/123) in deep ICH (P=0.033). The easier hematoma dispersion in lobes contributed to the inadequate drainage.
More advanced minimally invasive surgical equipment has been used in recent clinical trials, such as the Early Minimally-invasive Removal of Intracerebral Hemorrhage (ENRICH) (32) and Minimally Invasive Subcortical Para-fascicular Access for Clot Evacuation (MISPLACE) (33) trials, which achieved significant functional improvements. In contrast, our free-hand MIS procedure can be conducted at the bedside or in an emergency department, without general anesthesia and complicated equipment, and thus provides a reassuring approach for hematoma excavation. Target patients, especially those with regularly shaped hematoma, need to be carefully selected to ensure optimal surgical performance. In contrast, for patients with irregularly shaped hematoma, surgical approaches (such as endoscopic hematoma removal, or stereotactic navigation-guided hematoma removal using the BrainPath-Myriad and Artemis systems) should be considered to achieve optimal surgical outcomes.
The limitations of this study should be considered in the interpretation of our results. First, our findings were derived from a single-center retrospective analysis; thus, further prospective confirmation is required. Second, the results could be altered if different definitions of rebleeding or different CT parameters were to be applied.
Conclusions
In conclusion, the hematoma SRI can serve as an independent indicator of postoperative rebleeding and poor drainage in ICH patients following MIS.
Acknowledgments
We would like to thank each and every participant, and Mr. Youxia Ye for his efforts in polishing the manuscript.
Funding: This work was supported by
Footnote
Reporting Checklist: The authors have completed the STROCSS reporting checklist. Available at https://qims.amegroups.com/article/view/10.21037/qims-24-1105/rc
Conflicts of Interest: All authors have completed the ICMJE uniform disclosure form (available at https://qims.amegroups.com/article/view/10.21037/qims-24-1105/coif). X.Z. reports that this work was supported by the National Key R&D Program of China (No. 2022ZD0118005), the Chinese Academy of Medical Sciences Innovation Fund for Medical Sciences (No. 2019-I2M-5-029), and the Beijing Municipal Committee of Science and Technology Fund (No. Z201100005620010). The other authors have no conflicts of interest to declare.
Ethical Statement: The authors are accountable for all aspects of the work in ensuring that questions related to the accuracy or integrity of any part of the work are appropriately investigated and resolved. The study was conducted in accordance with the Declaration of Helsinki (as revised in 2013). The study was approved by the Ethics Board of Beijing Tiantan Hospital (No. KY2021-025-01), and informed consent was obtained from all the patients or their relatives.
Open Access Statement: This is an Open Access article distributed in accordance with the Creative Commons Attribution-NonCommercial-NoDerivs 4.0 International License (CC BY-NC-ND 4.0), which permits the non-commercial replication and distribution of the article with the strict proviso that no changes or edits are made and the original work is properly cited (including links to both the formal publication through the relevant DOI and the license). See: https://creativecommons.org/licenses/by-nc-nd/4.0/.
References
- Tsao CW, Aday AW, Almarzooq ZI, Alonso A, Beaton AZ, Bittencourt MS, et al. Heart Disease and Stroke Statistics-2022 Update: A Report From the American Heart Association. Circulation 2022;145:e153-639. [Crossref] [PubMed]
- van Asch CJ, Luitse MJ, Rinkel GJ, van der Tweel I, Algra A, Klijn CJ. Incidence, case fatality, and functional outcome of intracerebral haemorrhage over time, according to age, sex, and ethnic origin: a systematic review and meta-analysis. Lancet Neurol 2010;9:167-76. [Crossref] [PubMed]
- Greenberg SM, Ziai WC, Cordonnier C, Dowlatshahi D, Francis B, Goldstein JN, Hemphill JC 3rd, Johnson R, Keigher KM, Mack WJ, Mocco J, Newton EJ, Ruff IM, Sansing LH, Schulman S, Selim MH, Sheth KN, Sprigg N, Sunnerhagen KS. 2022 Guideline for the Management of Patients With Spontaneous Intracerebral Hemorrhage: A Guideline From the American Heart Association/American Stroke Association. Stroke 2022;53:e282-361. [Crossref] [PubMed]
- de Oliveira Manoel AL, Goffi A, Zampieri FG, Turkel-Parrella D, Duggal A, Marotta TR, Macdonald RL, Abrahamson S. The critical care management of spontaneous intracranial hemorrhage: a contemporary review. Crit Care 2016;20:272. [Crossref] [PubMed]
- Scaggiante J, Zhang X, Mocco J, Kellner CP. Minimally Invasive Surgery for Intracerebral Hemorrhage. Stroke 2018;49:2612-20. [Crossref] [PubMed]
- Hou D, Lu Y, Wu D, Tang Y, Dong Q. Minimally Invasive Surgery in Patients With Intracerebral Hemorrhage: A Meta-Analysis of Randomized Controlled Trials. Front Neurol 2021;12:789757. [Crossref] [PubMed]
- Hanley DF, Thompson RE, Rosenblum M, Yenokyan G, Lane K, McBee N, et al. Efficacy and safety of minimally invasive surgery with thrombolysis in intracerebral haemorrhage evacuation (MISTIE III): a randomised, controlled, open-label, blinded endpoint phase 3 trial. Lancet 2019;393:1021-32. [Crossref] [PubMed]
- Shah VA, Thompson RE, Yenokyan G, Acosta JN, Avadhani R, Dlugash R, McBee N, Li Y, Hansen BM, Ullman N, Falcone G, Awad IA, Hanley DF, Ziai WC. One-Year Outcome Trajectories and Factors Associated with Functional Recovery Among Survivors of Intracerebral and Intraventricular Hemorrhage With Initial Severe Disability. JAMA Neurol 2022;79:856-68. [Crossref] [PubMed]
- Seifman MA, Lewis PM, Rosenfeld JV, Hwang PY. Postoperative intracranial haemorrhage: a review. Neurosurg Rev 2011;34:393-407. [Crossref] [PubMed]
- Wang L, Zhang L, Mao Y, Li Y, Wu G, Li Q. Regular-Shaped Hematomas Predict a Favorable Outcome in Patients with Hypertensive Intracerebral Hemorrhage Following Stereotactic Minimally Invasive Surgery. Neurocrit Care 2021;34:259-70. [Crossref] [PubMed]
- Wang L, Luo S, Ren S, Yu H, Shen G, Wu G, Yang Q. Irregular-Shaped Hematoma Predicts Postoperative Rehemorrhage After Stereotactic Minimally Invasive Surgery for Intracerebral Hemorrhage. Front Neurol 2022;13:727702. [Crossref] [PubMed]
- Xu X, Chen X, Zhang J, Zheng Y, Sun G, Yu X, Xu B. Comparison of the Tada formula with software slicer: precise and low-cost method for volume assessment of intracerebral hematoma. Stroke 2014;45:3433-5. [Crossref] [PubMed]
- Zhou L, Wang W, Li Z, Wei H, Cai Q, Chen Q, Liu Z, Ye H, Song P, Cheng L, Lei P, Chen S. Clinical application of 3D-Slicer + 3D printing guide combined with transcranial neuroendoscopic in minimally invasive neurosurgery. Sci Rep 2022;12:20421. [Crossref] [PubMed]
- de Havenon A, Joyce E, Yaghi S, Ansari S, Delic A, Taussky P, Alexander M, Tirschwell D, Grandhi R. End-of-Treatment Intracerebral and Ventricular Hemorrhage Volume Predicts Outcome: A Secondary Analysis of MISTIE III. Stroke 2020;51:652-4. [Crossref] [PubMed]
- Awad IA, Polster SP, Carrión-Penagos J, Thompson RE, Cao Y, Stadnik A, et al. Surgical Performance Determines Functional Outcome Benefit in the Minimally Invasive Surgery Plus Recombinant Tissue Plasminogen Activator for Intracerebral Hemorrhage Evacuation (MISTIE) Procedure. Neurosurgery 2019;84:1157-68. [Crossref] [PubMed]
- Demchuk AM, Dowlatshahi D, Rodriguez-Luna D, Molina CA, Blas YS, Dzialowski I, et al. Prediction of haematoma growth and outcome in patients with intracerebral haemorrhage using the CT-angiography spot sign (PREDICT): a prospective observational study. Lancet Neurol 2012;11:307-14. [Crossref] [PubMed]
- Pérez-Beteta J, Molina-García D, Ortiz-Alhambra JA, Fernández-Romero A, Luque B, Arregui E, et al. Tumor Surface Regularity at MR Imaging Predicts Survival and Response to Surgery in Patients with Glioblastoma. Radiology 2018;288:218-25. [Crossref] [PubMed]
- Fujii Y, Takeuchi S, Sasaki O, Minakawa T, Tanaka R. Multivariate analysis of predictors of hematoma enlargement in spontaneous intracerebral hemorrhage. Stroke 1998;29:1160-6. [Crossref] [PubMed]
- Barras CD, Tress BM, Christensen S, MacGregor L, Collins M, Desmond PM, Skolnick BE, Mayer SA, Broderick JP, Diringer MN, Steiner T, Davis SM. Density and shape as CT predictors of intracerebral hemorrhage growth. Stroke 2009;40:1325-31. [Crossref] [PubMed]
- Shimoda Y, Ohtomo S, Arai H, Okada K, Tominaga T. Satellite Sign: A Poor Outcome Predictor in Intracerebral Hemorrhage. Cerebrovasc Dis 2017;44:105-12. [Crossref] [PubMed]
- Li Q, Liu QJ, Yang WS, Wang XC, Zhao LB, Xiong X, Li R, Cao D, Zhu D, Wei X, Xie P. Island Sign: An Imaging Predictor for Early Hematoma Expansion and Poor Outcome in Patients With Intracerebral Hemorrhage. Stroke 2017;48:3019-25. [Crossref] [PubMed]
- Boulouis G, Morotti A, Charidimou A, Dowlatshahi D, Goldstein JN. Noncontrast Computed Tomography Markers of Intracerebral Hemorrhage Expansion. Stroke 2017;48:1120-5. [Crossref] [PubMed]
- Webb AJ, Ullman NL, Morgan TC, Muschelli J, Kornbluth J, Awad IA, et al. Accuracy of the ABC/2 Score for Intracerebral Hemorrhage: Systematic Review and Analysis of MISTIE, CLEAR-IVH, and CLEAR III. Stroke 2015;46:2470-6. [Crossref] [PubMed]
- Huttner HB, Steiner T, Hartmann M, Köhrmann M, Juettler E, Mueller S, Wikner J, Meyding-Lamade U, Schramm P, Schwab S, Schellinger PD. Comparison of ABC/2 estimation technique to computer-assisted planimetric analysis in warfarin-related intracerebral parenchymal hemorrhage. Stroke 2006;37:404-8. [Crossref] [PubMed]
- Liao R, Liu L, Song B, Wan X, Wang S, Xu J. 3D-Slicer Software-Assisted Neuroendoscopic Surgery in the Treatment of Hypertensive Cerebral Hemorrhage. Comput Math Methods Med 2022;2022:7156598. [Crossref] [PubMed]
- Fisher CM. Pathological observations in hypertensive cerebral hemorrhage. J Neuropathol Exp Neurol 1971;30:536-50. [Crossref] [PubMed]
- Greenberg CH, Frosch MP, Goldstein JN, Rosand J, Greenberg SM. Modeling intracerebral hemorrhage growth and response to anticoagulation. PLoS One 2012;7:e48458. [Crossref] [PubMed]
- Li Y, Wang J, Li Z, Zhang X, Tian B, Yang Y, Li M, Wang B, Xia Y, Ma T, Yang Y, Gao G, Li L. Computed Tomography Angiography Spot Sign as an Indicator for Ultra-Early Stereotactic Aspiration of Intracerebral Hemorrhage. World Neurosurg 2018;109:e136-43. [Crossref] [PubMed]
- Miki K, Yagi K, Nonaka M, Iwaasa M, Abe H, Morishita T, Arima H, Inoue T. Spot sign as a predictor of rebleeding after endoscopic surgery for intracerebral hemorrhage. J Neurosurg 2019;130:1485-90. [Crossref] [PubMed]
- Dowlatshahi D, Brouwers HB, Demchuk AM, Hill MD, Aviv RI, Ufholz LA, et al. Predicting Intracerebral Hemorrhage Growth With the Spot Sign: The Effect of Onset-to-Scan Time. Stroke 2016;47:695-700. [Crossref] [PubMed]
- Polster SP, Carrión-Penagos J, Lyne SB, Gregson BA, Cao Y, Thompson RE, et al. Intracerebral Hemorrhage Volume Reduction and Timing of Intervention Versus Functional Benefit and Survival in the MISTIE III and STICH Trials. Neurosurgery 2021;88:961-70. [Crossref] [PubMed]
- Pradilla G, Ratcliff JJ, Hall AJ, Saville BR, Allen JW, Paulon G, et al. Trial of Early Minimally Invasive Removal of Intracerebral Hemorrhage. N Engl J Med 2024;390:1277-89. [Crossref] [PubMed]
- Labib MA, Shah M, Kassam AB, Young R, Zucker L, Maioriello A, Britz G, Agbi C, Day JD, Gallia G, Kerr R, Pradilla G, Rovin R, Kulwin C, Bailes J. The Safety and Feasibility of Image-Guided BrainPath-Mediated Transsulcul Hematoma Evacuation: A Multicenter Study. Neurosurgery 2017;80:515-24. [Crossref] [PubMed]