Quantitative assessment of pulmonary blood flow redistribution in the classification of group 1 and group 4 pulmonary hypertension
Introduction
Pulmonary hypertension (PH) is a complication of cardiopulmonary disease that affects approximately 1% of the global population (1). Persistently elevated pulmonary pressure and increased right heart load can lead to irreversible remodeling of pulmonary vessels in at least 50% of patients with right heart failure (2). PH has been classified into five groups by the World Health Organization. Of them, pulmonary arterial hypertension (PAH) and chronic thromboembolic pulmonary hypertension (CTEPH) are both characterized by pre-capillary PH, with a low incidence of 5–20 cases per 100,000 (3). However, a significantly higher alveolar dead space fraction has been reported in patients with CTEPH than in those with PAH (4,5). Additionally, the first-line treatment of CTEPH is pulmonary endarterectomy or balloon pulmonary angioplasty, while targeted treatments (phosphodiesterase type 5 inhibitors, soluble guanylate cyclase stimulators, endothelin receptor antagonists, prostacyclin analogs, and prostacyclin receptor agonists) are licensed for patients with PAH (1). Thus, in the algorithm for diagnosis of PH, once group-2 or -3 PH (the two most commonly seen PH) have been excluded, patients with persistent or hemodynamically severe PH should be referred to PH centers for further classification between CTEPH and PAH (1,6).
Right heart catheterization (RHC) serves as the gold standard for measuring and classifying PH. However, given its invasive nature and potential complications, performing RHC requires expertise and meticulous methodology (7), rendering it impractical for clinical studies involving large cohorts of subjects. Although echocardiography offers comprehensive information on heart morphology for the PH evaluation, the study reported that the estimated systolic pulmonary arterial pressure (SPAP) on echocardiography lacks relevance in sub-classification and therapeutic decision-making (8).
Computed tomography pulmonary angiography (CTPA) is essential for subgrouping PH, as CTPA offers vital information regarding underlying lung diseases. Recent advances in image analysis have facilitated volumetric assessments of the intrapulmonary vasculature with different calibers, including pulmonary vessels <5 mm2 (BV5), between 5 and 10 mm2 (BV5–10), and pulmonary vessels >10 mm2 (BV10) in cross-sectional area (9), as well as the volumetric assessments of pulmonary arteries or pulmonary veins (10). These measurements enable the evaluation of pulmonary vasculopathy and serve as essential biomarkers for evaluating major lung diseases.
In patients with chronic obstructive pulmonary disease (COPD), the ratio of BV5 to total blood volume (BV5/TBV) was lower (32.2% vs. 37.7%, P=0.003), and BV10/TBV was higher (50.2% vs. 43.5%, P=0.001) in those with PH-COPD compared to non-PH-COPD subjects (11). Additionally, the BV5/TBV ratio is associated with the severity, exacerbation, and lung function of patients with asthma (12). Poletti et al. (13) demonstrated a significant decrease in BV5/TBV and a significant increase in BV10/TBV in patients with coronavirus disease 2019 (COVID-19) and influenza pneumonia. Salerno et al. (14) found that an increase in BV5–10 and BV10 was associated with a lower diffusion capacity of carbon monoxide in patients with COVID-19. These findings suggest that measuring pulmonary vessels may be a noninvasive indicator of pulmonary vascular pathology and may help in evaluating pulmonary blood flow redistribution.
Utilizing the automatic segmentation algorithm of pulmonary vessels, this study aimed to reveal the characteristics of pulmonary blood flow redistribution in two typical pre-capillary PH conditions, PAH and CTEPH. The evaluation of pulmonary blood circulation encompassed the bilateral ventricles of the heart, central pulmonary arteries, pulmonary vessels with different calibers (BV5, BV5–10, and BV10), and pulmonary arteries and veins. We present this article in accordance with the STROBE reporting checklist (available at https://qims.amegroups.com/article/view/10.21037/qims-24-644/rc).
Methods
Study cohort and design
This retrospective study was conducted in accordance with the Declaration of Helsinki (as revised in 2013). Approval was granted by the ethics committee of The First Affiliated Hospital of Xi’an Jiaotong University (No. 2013-114-1), and the requirement for written consent for this retrospective analysis was waived.
Overall, 69 patients who underwent both RHC and CTPA examination for suspected PH between November 2013 and April 2021 were retrospectively enrolled. The inclusion criteria were as follows: (I) patients who underwent an RHC examination, (II) patients who underwent a CTPA examination before the RHC test, and (III) CTPA images stored in the digital imaging and communications in medicine (DICOM) format. The exclusion criteria are as follows: (I) missing vital clinical information (n=2); (II) incomplete CTPA scan scope or poor image quality (n=4); (III) a time interval between RHC and CTPA exceeds 2 weeks (n=3); and (IV) age <18 years (n=1).
A mean pulmonary arterial pressure (mPAP) of >20 mmHg in the RHC test was used as a threshold for defining PH. This aligns with established criteria outlined in the guidelines of the European Society of Cardiology and the European Respiratory Society, with patients categorized into five sub-groups based on these guidelines (1). Specifically, individuals who fall under group 2, 3, and 5 PH were excluded from the analysis (n=13). Finally, the study focused on three groups: non-PH (18 cases), PAH (12 cases), and CTEPH (16 cases), as illustrated in Figure 1.
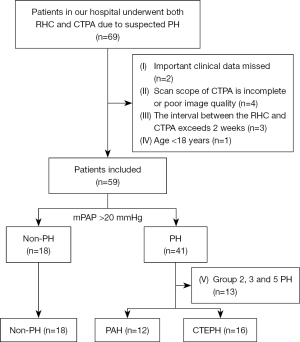
RHC
One interventional physician with more than 5 years of experience performed the procedure. After applying local anesthesia, RHC was performed using an 8 French introducer sheath placed in the right internal jugular vein under ultrasound guidance. A 7 French catheter (Swan-Ganz; Edwards Life Sciences, Santa Ana, California, USA) was placed in the main pulmonary artery (MPA) to measure mPAP, SPAP, and diastolic pulmonary arterial pressure (DPAP).
Echocardiography
An expert cardiologist evaluated the echocardiography using a high-resolution device (Philips, iE-33, color Doppler Ultrasound Machine, San Jose, California, USA) with a 5-MHz linear array transducer. Doppler echocardiography was performed following the recommendations of the American Society of Echocardiography (15). The diameters of the left ventricle (LVd) and right ventricle (RVd) were measured in a four-chamber view at the end of the dilated phase.
CTPA imaging
All CTPA images were obtained using a 256-detector computed tomography (CT) scanner (Brilliance iCT 256; Philips Health Systems, Amsterdam, The Netherlands) with patients in a supine position and instructed to hold their breath. The scanning scope was positioned from the apex to the base of the lung. The scan parameters were as follows: tube voltage, 120 kVp; automatic tube current (59–189 mA, based on the patient’s weight); rotation time, 0.28 s; pitch, 0.992:1; and reconstruction matrix, 512×512.
A contrast agent (Iopromide, Bayer, Guangzhou, China; 370 mg iodine/mL, 50 mL) was administered intravenously at an injection rate of 5 mL/s with a 40-mL saline chaser bolus. The scan was automatically triggered when the CT value of the right atrium (close to the entrance of the inferior vena cava) exceeded 120 Hounsfield units (HU). All data were reconstructed using a complete iterative reconstruction technique.
Three-dimensional (3D) volumetry of the central pulmonary arteries
An experienced radiologist (H.H.) imported the CTPA images into Materialize Mimics (version 19.0, Materialise, Leuven, Belgium) to construct a 3D model of the central pulmonary arteries. The initial masking was performed using the density threshold ranging from −200 to 500 HU. Subsequently, non-relevant structures, such as bones and tissues outside the pulmonary artery, were cut by the freehand. The dynamic region-growing method was then applied, with a seed placed within the MPA to remove scattered branches (Figure 2A). Manual correction (by another 10-year experienced radiologist, W.Z.) was allowed when segmentation was not satisfied. This process resulted in the generation of the central pulmonary arteries (Figure 2B). The centerline of the segmented 3D object was then fitted (Figure 2C), and the vessel volumes of the MPA (VMPA), right pulmonary artery (VRPA), and left pulmonary artery (VLPA) were calculated and recorded in cubic centimeters (cm3).
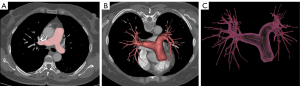
Quantitative assessment of the intrapulmonary vessels
Quantitative assessment of the pulmonary vessels was performed using a Lung Workstation (Dexin, Xi’an, China, http://www.dexhin.com/). A 3D approach was employed to segment the region of the bilateral lungs (Figure 3A) and intrapulmonary vessels (Figure 3B), as previously described (16,17). Lobar fissures were identified using the method previously described (18). Total blood volume (TBV) was quantified for the whole lung (WL), right lung (RL), left lung (LL), and five lobes, including the right upper lobe (RUL), right middle lobe (RML), right lower lobe (RLL), left upper lobe (LUL), and left lower lobe (LLL).
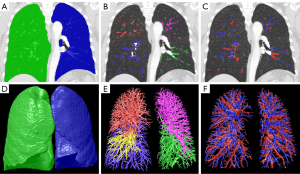
The TBVs were then categorized based on their diameters into BV5, BV5–10, and BV10 (11,13). Pulmonary vessel volumes (TBV, BV5, BV5–10, BV10) were normalized to the volume of the corresponding lung or lobe. This normalization process aimed to eliminate the influence of individual variations in lung or lobe size on vessel volume measurements, ensuring comparability across subjects.
Normalized vessel volume (‰) = blood vessel volume (mL)/corresponding lung or lobe volume (mL) × 1,000.
The ratios of BV5, BV5–10, and BV10 to TBV were calculated and expressed as BV5/TBV, BV5–10/TBV, and BV10/TBV, respectively.
As previously reported, the pulmonary arteries and veins were automatically differentiated (Figure 3C) using a convolutional neural network model (19). The arterial total blood volume (aTBV) and the venous total blood volume (vTBV) were also calculated and normalized.
The bilateral lungs (Figure 3D), intrapulmonary vessel (Figure 3E), and pulmonary arteries and veins (Figure 3F) were also shown in a 3D way.
Statistical analysis
Statistical analyses were performed using Statistical Package for the Social Sciences (SPSS) software (version 25.0; IBM Corp., Armonk, NY, USA). Descriptive statistics were expressed as mean ± standard deviation (SD) or median with interquartile range for continuous variables and as frequency and percentages for categorical variables. The normality of parameter distribution was assessed using the Shapiro-Wilk test. One-way analysis of variance (ANOVA) was used to determine differences in continuous values with a normal distribution. Multiple comparisons between the two groups were conducted using the least significant difference (LSD) for equal variance or Tamhane’s T2 test for unequal variance. Wilcoxon’s signed-rank test and the Kruskal-Wallis test were applied for continuous values with a non-normal distribution.
Linear regression models were constructed, incorporating all statistically significant parameters, to identify the most discriminative variables between non-PH and PAH, non-PH and CTEPH, as well as PAH and CTEPH groups. A significance level of P<0.05 was adopted for all statistical analyses.
Results
Basic clinical characteristics
Overall, 46 patients (33 females and 13 males) were included, with 18 cases (representing 39.1%) in the non-PH group, 12 (26.1%) in the PAH group, and 16 (34.8%) in the CTEPH group. Patients in the PAH group were younger than those in the non-PH and CTEPH groups (P<0.05). Of the 12 patients with PAH, 5 were idiopathic, 4 were associated with congenital heart disease (2 cases of ventricular septal defect, 1 case of atrial septal defect, and 1 case of patent ductus arteriosus), and 3 were associated with connective tissue disease (1 case of Behcet disease, 1 case of systemic lupus erythematosus, and 1 case of Sjogren’s syndrome). The prevalence of congenital heart disease was higher in both the non-PH group and PAH group than in the CTEPH group (P<0.05) (Table 1).
Table 1
Items | Non-PH (n=18) | PAH (n=12) | CTEPH (n=16) | F/χ2 | P |
---|---|---|---|---|---|
Age (years) | 50.11±17.37 | 34.33±11.23† | 54.88±10.09‡ | 8.213 | 0.001 |
Sex (male/female) | 7/11 | 1/11 | 5/11 | ¶ | 0.192 |
Signs | |||||
Aortic systolic blood pressure (mmHg) | 126.50±21.22 | 113.9±22.08 | 116.13±11.51 | 2.010 | 0.147 |
Aortic diastolic blood pressure (mmHg) | 75.89±11.20 | 74.00±16.06 | 77.69±8.32 | 0.344 | 0.716 |
Respiratory rate (times/min) | 18 [18–20] | 20 [20–20]† | 19.5 [18–20] | 4.247 | 0.021 |
Pulse (times/min) | 77.33±8.00 | 87.50±9.73 | 84.31±15.41 | 3.148 | 0.053 |
Symptoms | |||||
Dyspnea | 5 (27.8) | 6 (50.0) | 10 (62.5) | 4.240§ | 0.120 |
Fever | 1 (5.6) | 0 (0.0) | 0 (0.0) | ¶ | 1.000 |
Edema of lower limbs | 1 (5.6) | 2 (16.7) | 2 (12.5) | ¶ | 0.607 |
Chest pain | 1 (5.6) | 1 (8.3) | 1 (6.3) | ¶ | 1.000 |
Palpitation | 0 (0.0) | 1 (8.3) | 2 (12.5) | ¶ | 0.348 |
Syncope | 1 (5.6) | 2 (16.7) | 0 (0.0) | ¶ | 0.253 |
Past medical history | |||||
Congenital heart disease | 10 (55.6) | 4 (33.3) | 0 (0.0) | 12.431§ | 0.002 |
Pulmonary embolism | 2 (11.1) | 0 (0.0) | 1 (6.3) | ¶ | 0.340 |
Coronary heart disease | 1 (5.6) | 0 (0.0) | 1 (6.3) | ¶ | 0.541 |
Hypertension | 1 (5.6) | 1 (8.3) | 3 (18.8) | 1.630§ | 0.443 |
Diabetes | 1 (5.6) | 0 (0.0) | 0 (0.0) | ¶ | 0.392 |
RHC | |||||
SPAP (mmHg) | 27 [24–32] | 75.58±21.06† | 79.93±19.25† | 33.176 | <0.001 |
mPAP (mmHg) | 18 [16–19] | 48.67±14.54† | 46.27±13.30† | 28.459 | <0.001 |
DPAP (mmHg) | 10 [8.5–11.25] | 32.41±12.80† | 26.60±10.96† | 19.733 | <0.001 |
Data are presented as mean ± standard deviation, n, median [interquartile range] or n (%). †, compared with non-PH; ‡, compared with PAH; §, χ2 test; ¶, Fisher exact probability test. PH, pulmonary hypertension; PAH, pulmonary arterial hypertension; CTEPH, chronic thromboembolic PH; RHC, right heart catheterization; SPAP, systolic pulmonary arterial pressure; mPAP, mean pulmonary arterial pressure; DPAP, diastolic pulmonary arterial pressure.
All the RHC parameters (SPAP, mPAP, and DPAP) were significantly higher in the PAH group and CTEPH group than those in the non-PH group (P<0.05; Table 1).
Comparisons of four chambers and central pulmonary arteries in three groups
The RVd was significantly higher in the PAH and CTEPH groups than in the non-PH group (P<0.05). The LVd was not significantly different among the three groups (P>0.05). In the volumetric analysis of the central pulmonary arteries, the VMPA and VRPA were significantly higher in the PAH group and CTEPH group than those in the non-PH group (P<0.05) (Table 2).
Table 2
Items | Non-PH (n=18) | PAH (n=12) | CTEPH (n=16) | F | P |
---|---|---|---|---|---|
RVd (mm) | 25.92±3.26 | 33.50±6.88† | 35.00±3.72† | 14.204 | <0.001 |
LVd (mm) | 35.75±7.40 | 30 (26.0–46.50) | 31.43±2.82 | 0.917 | 0.409 |
VMPA (cm3) | 24.12 (20.56–32.14) | 58.49±24.27† | 51.13 (41.48–74.63)† | 8.172 | 0.001 |
VRPA (cm3) | 21.70 (13.11–31.50) | 51.14±13.43† | 49.27 (39.56–79.15)† | 7.783 | 0.001 |
VLPA (cm3) | 21.60 (13.41–29.22) | 36.49±12.71 | 42.39 (25.81–66.62)† | 4.675 | 0.015 |
Data are presented as mean ± standard deviation or median (interquartile range). †, compared with non-PH. PH, pulmonary hypertension; PAH, pulmonary arterial hypertension; CTEPH, chronic thromboembolic PH; RVd, diameter of the right ventricle; LVd, diameter of left ventricle; VMPA, volume of main pulmonary artery; VRPA, volume of right pulmonary artery; VLPA, volume of left pulmonary artery.
Comparisons of the quantitative pulmonary vessel volumes among three groups
The TBVs were larger in the PAH group than those in the non-PH group, and significant differences were found in the RL, RML, and LUL (P<0.05). The BV10s were larger in the PAH group than those in the non-PH group, and significant differences were found in the WL, RL, LL, RUL, RML, RLL, and LUL (P<0.05). The BV5–10s of the PAH group increased compared with those of the non-PH group, with statistical significance found only in the RML (P<0.05). The BV5s of PAH showed a slight decrease in the PAH group, but all differences were not significant (P>0.05) (Table 3).
Table 3
Items | Non-PH (n=18) | PAH (n=12) | CTEPH (n=16) | F | P |
---|---|---|---|---|---|
TBVWL (‰) | 80.33±16.72 | 93.85±31.86 | 68.81±14.22‡ | 4.639 | 0.015 |
BV5WL (‰) | 18.31±4.86 | 16.83±6.11 | 16.57±3.78 | 0.581 | 0.564 |
BV5–10WL (‰) | 38.87±8.13 | 44.32±16.83 | 33.52±7.14‡ | 3.266 | 0.048 |
BV10WL (‰) | 23.59±10.61 | 32.69±13.53† | 18.73±6.10‡ | 6.234 | 0.004 |
TBVRL (‰) | 77.98±17.50 | 96.79±34.63† | 61.27±16.61†‡ | 7.863 | 0.001 |
BV5RL (‰) | 19.40±5.20 | 19.32±7.62 | 17.66±5.39 | 0.382 | 0.685 |
BV5–10RL (‰) | 40.52±9.45 | 48.42±19.45 | 33.62±9.15‡ | 4.497 | 0.017 |
BV10RL (‰) | 18.05±9.60 | 29.06±15.01† | 9.99±4.26†‡ | 10.819 | <0.001 |
TBVLL (‰) | 80.56±17.28 | 96.32±36.13 | 73.54±15.20‡ | 3.196 | 0.052 |
BV5LL (‰) | 18.90±5.67 | 17.24±7.64 | 17.53±4.19 | 0.239 | 0.788 |
BV5–10LL (‰) | 40.49±9.27 | 46.68±20.29 | 37.69±8.99 | 1.602 | 0.214 |
BV10LL (‰) | 21.16±9.70 | 32.41±15.83† | 18.32±6.43‡ | 4.322 | 0.020 |
TBVRUL (‰) | 71.30±18.99 | 86.89±34.04 | 55.15±13.64†‡ | 6.659 | 0.003 |
BV5RUL (‰) | 19.70±4.66 | 19.85±7.97 | 17.12±4.94 | 1.082 | 0.348 |
BV5–10RUL (‰) | 38.00±11.00 | 44.78±19.34 | 30.14±7.41‡ | 4.449 | 0.018 |
BV10RUL (‰) | 13.61±8.20 | 22.26±12.07† | 7.90±2.92‡ | 8.991 | 0.001 |
TBVRML (‰) | 61.82±16.45 | 81.74±28.25† | 50.49±19.68‡ | 7.275 | 0.002 |
BV5RML (‰) | 16.94±6.40 | 19.10±7.90 | 15.19±4.92 | 1.270 | 0.291 |
BV5–10RML (‰) | 32.33±8.50 | 41.91±17.17† | 27.76±10.71‡ | 4.724 | 0.014 |
BV10RML (‰) | 12.55±9.97 | 20.72±9.95† | 7.54±5.32‡ | 7.117 | 0.002 |
TBVRLL (‰) | 79.74±21.48 | 92.63±36.64 | 66.42±19.19‡ | 3.462 | 0.041 |
BV5RLL (‰) | 17.12±7.70 | 15.56±8.25 | 17.28±6.61 | 0.196 | 0.823 |
BV5–10RLL (‰) | 40.55±11.66 | 45.52±20.44 | 36.74±10.82 | 1.276 | 0.290 |
BV10RLL (‰) | 22.07±10.52 | 31.55±18.22† | 12.40±5.99†‡ | 8.098 | 0.001 |
TBVLUL (‰) | 69.88±18.9 | 88.10±32.38† | 65.99±16.12‡ | 3.436 | 0.042 |
BV5LUL (‰) | 18.46±5.74 | 17.81±8.60 | 17.31±4.40 | 0.101 | 0.904 |
BV5–10LUL (‰) | 35.50±9.85 | 42.05±18.08 | 33.72±9.42 | 1.558 | 0.223 |
BV10LUL (‰) | 15.92±8.58 | 28.23±14.82† | 14.95±5.24‡ | 5.630 | 0.007 |
TBVLLL (‰) | 81.65±19.12 | 89.04±37.31 | 73.18±14.71 | 1.428 | 0.252 |
BV5LLL (‰) | 16.62±7.74 | 13.82±6.73 | 15.47±4.31 | 0.449 | 0.641 |
BV5–10LLL (‰) | 40.62±11.61 | 44.19±21.49 | 37.68±8.26 | 0.712 | 0.497 |
BV10LLL (‰) | 24.41±10.15 | 31.04±14.85 | 20.04±8.58‡ | 2.477 | 0.096 |
All the values of pulmonary vessel volume (TBV, BV5, BV5–10, BV10) were normalized. Data are presented as mean ± standard deviation. †, compared with non-PH; ‡, compared with PAH. PH, pulmonary hypertension; PAH, pulmonary arterial hypertension; CTEPH, chronic thromboembolic PH; TBV, total blood volume; WL, whole lung; BV5, blood volume of vessels with cross-sectional area <5 mm2; BV5–10, blood volume of vessels with cross-sectional area between 5 and 10 mm2; BV10, blood volume of vessels with cross-sectional area >10 mm2; RL, right lung; LL, left lung; RUL, right upper lobe; RML, right middle lobe; RLL, right lower lobe; LUL, left upper lobe; LLL, left lower lobe.
The TBVs were significantly decreased in the CTEPH group than those in the non-PH group, and a significant decrease was found in the RL and RUL (P<0.05). The BV10 of the RL and RLL was significantly lower in the CTEPH group than those in the non-PH group (P<0.05). The BV5s and BV5–10s of the CTEPH group were slightly decreased compared with those of the non-PH group, but none of the differences were significant (P>0.05) (Table 3).
The BV10s of CTEPH were significantly lower than those of the PAH group for the WL, RL, LL, RUL, RML, RLL and LUL (P<0.05). The BV5–10 of the CTEPH group showed lower values than those of the PAH group for the WL, RL, RUL, and RML significantly (P<0.05) (Table 3).
The ratio of smaller-caliber vessels (BV5/TBV) was significantly lower in the PAH group than in the non-PH and CTEPH groups for the WL, RL, LL, RUL, and LUL (P<0.05). The ratio of larger-caliber vessels (BV10/TBV) was significantly higher in the PAH group than in the non-PH and CTEPH groups for the RL, LL, RUL, and LUL (P<0.05) (Figure 4).
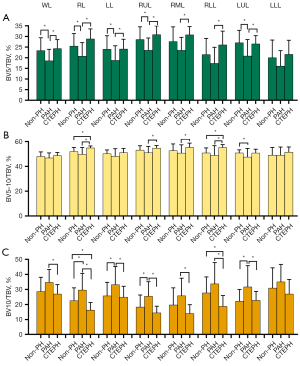
The aTBV and arterial BV10 values were significantly higher in the PAH group than in the non-PH and CTEPH groups (P<0.05). However, the aTBV of CTEPH decreased compared with that of non-PH, although it was not statistically significant (P>0.05) (Table 4).
Table 4
Items | Non-PH (n=18) | PAH (n=12) | CTEPH (n=16) | F/χ2 | P |
---|---|---|---|---|---|
aTBV (‰) | 32.91±9.52 | 42.18±13.67† | 27.76±7.96‡ | 6.544 | 0.003 |
aBV5 (‰) | 10.23±3.15 | 9.11±3.40 | 9.15±2.67 | 0.671 | 0.516 |
aBV5–10 (‰) | 20.76±5.30 | 23.58±7.88 | 18.10±4.85‡ | 2.819 | 0.071 |
aBV10 (‰) | 11.81±6.32 | 18.19±7.29† | 9.36±3.83‡ | 7.758 | 0.001 |
vTBV (‰) | 29.73±7.20 | 35.58±16.35 | 25.01±4.79‡ | 3.727 | 0.033 |
vBV5 (‰) | 8.05±2.02 | 7.72±3.28 | 7.42±1.26 | 0.347 | 0.709 |
vBV5–10 (‰) | 17.65±3.69 | 20.74±9.91 | 15.42±2.69‡ | 2.773 | 0.074 |
vBV10 (‰) | 11.78±5.30 | 14.50±7.09 | 9.36±2.76‡ | 3.676 | 0.034 |
All the values of pulmonary vessel volume were normalized. Data are presented as mean ± standard deviation. †, compared with non-PH; ‡, compared with PAH. PH, pulmonary hypertension; PAH, pulmonary arterial hypertension; CTEPH, chronic thromboembolic PH; aTBV, arterial total blood volume; aBV5, arterial blood volume of vessels with cross-sectional area <5 mm2; aBV5–10, arterial blood volume of vessels with cross-sectional area between 5 and 10 mm2; aBV10, arterial blood volume of vessels with cross-sectional area >10 mm2; vTBV, venous total blood volume; vBV5, venous blood volume of vessels with cross-sectional area <5 mm2; vBV5–10, venous blood volume of vessels with cross-sectional area between 5 and 10 mm2; vBV10, venous blood volume of vessels with cross-sectional area >10 mm2.
Regarding venous blood volumes, vTBV, venous BV10, and venous BV5–10 of PAH increased without significance compared with those of non-PH (P>0.05). Conversely, vTBV and venous BV10 of CTEPH decreased significantly compared to those of PAH group (P<0.05) (Table 4).
Figure 5 showed illustrations of the 3D volumetry of the central pulmonary arteries, intrapulmonary vessels, pulmonary arteries, and veins in the three groups.
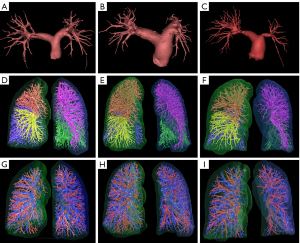
Linear regression
The RVd, VMPA, and BV5–10RML values were identified as the most distinguishing parameters between the non-PH group and PAH group, while RVd, VMPA, and BV10RLL were found to be the most distinguishing parameters between the non-PH group and CTEPH group. Age and BV10RUL were the most distinguishing parameters between the PAH group and CTEPH group (Table 5). In the receiver operating characteristic (ROC) analysis, the area under the curve (AUC) was calculated to be 0.950 in the differentiation between PAH and CTEPH (Figure 6).
Table 5
Groups | Selected factors | Beta | 95% CI of beta | Odds ratio | t | P |
---|---|---|---|---|---|---|
Non-PH vs. PAH | Intercept | −1.603 | −2.388 to −0.817 | – | −4.305 | <0.001 |
RVd | 0.045 | 0.022 to 0.067 | 0.575 | 4.224 | 0.001 | |
VMPA | 0.008 | 0.002 to 0.015 | 0.397 | 2.767 | 0.013 | |
BV5–10RML | 0.011 | 0.001 to 0.021 | 0.312 | 2.217 | 0.041 | |
Non-PH vs. CTEPH | Intercept | −1.651 | −3.103 to −0.198 | – | −2.357 | 0.028 |
RVd | 0.096 | 0.056 to 0.136 | 0.594 | 4.981 | <0.001 | |
BV10RLL | −0.029 | −0.053 to −0.005 | −0.284 | −2.490 | 0.021 | |
VMPA | 0.006 | 0.000 to 0.011 | 0.241 | 2.223 | 0.037 | |
PAH vs. CTEPH | Intercept | 1.457 | – | – | 1.362 | 0.187 |
Age | 0.046 | 0.011 to 0.082 | 0.475 | 2.686 | 0.013 | |
BV10RUL | −0.053 | −0.102 to −0.004 | −0.400 | −2.263 | 0.034 |
PH, pulmonary hypertension; PAH, pulmonary arterial hypertension; CTEPH, chronic thromboembolic PH; CI, confidence interval; RVd, diameter of the right ventricle; VMPA, volume of main pulmonary artery; BV5–10, blood volume of vessels with cross-sectional area between 5 and 10 mm2; RML, right middle lobe; BV10, blood volume of vessels with cross-sectional area >10 mm2; RLL, right lower lobe; RUL, right upper lobe.
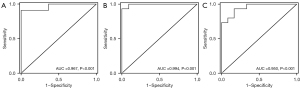
Discussion
In this study, we aimed to investigate the value of the quantitative assessment of pulmonary blood flow redistribution in the classification of two typical pre-capillary PH, PAH and CTEPH. Our evaluation included the diameter of bilateral ventricles, the volume of the central pulmonary arteries, the intrapulmonary vessel volume with different calibers (BV5, BV5–10, and BV10), as well as the blood volume of the pulmonary arteries and veins. Several notable results were obtained. First, patients with PAH were younger and exhibited higher pulmonary arterial pressures compared to the non-PH group. Dilations were observed in RVd, MPA, and RPA, along with significant dilation in intrapulmonary blood vessels of large caliber. Additionally, a decrease in BV5/TBV was noted for bilateral lungs and bilateral upper lobes. Notably, pulmonary vascular dilatation was statistically significant in the pulmonary arteries but not in the pulmonary veins compared to the non-PH group.
Previous study has also reported a higher occurrence of PAH in young females (20). In our PAH group, 33.3% (4/12) of patients had a history of congenital heart disease. The systemic-to-pulmonary shunt in patients with PAH allows for persistently increased pressure and volume overload of the pulmonary circulation (21). And the endothelial proliferation and remodeling of the pulmonary vasculature may explain the increased volume of large- and medium-sized vessels of the idiopathic or with connective tissue disease-associated PAH, especially in the pulmonary arteries.
Second, in the CTEPH group, pulmonary arterial pressure was significantly higher than in the non-PH group. Enlargements were observed in RVd, MPA, RPA, and LPA, while TBVs were significantly reduced, particularly in the RL and RUL. The trend of shrinking pulmonary blood vessels was observed across different calibers, with significant reductions noted in large-caliber vessels for the RL and RLL. Unlike patients with PAH, both pulmonary arteries and venous vessels showed a reduction in CTEPH, though not statistically significant.
Group 4 pulmonary hypertension (CTEPH), caused by persistent unresolved thromboembolic occlusion of the pulmonary arteries, showed enlarged central pulmonary arteries and reduced intrapulmonary vessels. This may explain the more frequent embolism in the RL, particularly in the RLL, due to the gravitational gradient of pulmonary perfusion and the geometry of the pulmonary vascular (22). Organized thromboembolic occlusion initiated in large pulmonary arteries may explain the significant reduction observed in larger pulmonary calibers (23). Both the pulmonary arteries and veins were reduced in the CTEPH group, although the difference was not statistically significant. These results are consistent with those of previous studies. Huang et al. (24) showed that the subpleural small vessels in CTEPH increased after treatment, as indicated by an increase of 11.3% in the BV5/TBV ratio. The increase in the BV5/TBV ratio correlated with the percentage change in mPAP (r=−0.56; P=0.001) and pulmonary vascular resistance (r=−0.64; P<0.001). Kroeger et al. (25) also showed that patients with CTEPH had a higher percentage of malperfused lungs by the quantification of volumetric iodine.
As the clinical management of PAH and CTEPH is different, understanding these patterns of vascular remodeling can inform clinical decision-making and treatment strategies. Despite radiological signs on CTPA images, including ring-like stenoses, webs, slits, eccentric filling defects, or total occlusion (26) aiding CTEPH identification, their sensitivity remains insufficient, ranging from 76% to 80% (27,28). Our data showed that the TBVs and BV10s in the PAH group were significantly higher than those in the CTEPH group. Shahin et al. (29) also proved that PAH has a higher pulmonary blood volume than CTEPH using peeled blood volume.
For instance, the identification of dilated pulmonary vessels in PAH patients may prompt the initiation of targeted therapies aimed at vasodilation and pulmonary vascular remodeling. Conversely, recognizing constricted vessels in CTEPH patients may warrant consideration for surgical interventions, such as pulmonary endarterectomy, to alleviate pulmonary artery obstruction and improve hemodynamic status. Further, the linear regression analysis revealed age and BV10RUL as independent predictors for differentiating CTEPH from PAH. This suggests the potential of measuring larger-caliber pulmonary vessels (BV10RLL) as a biomarker for CTEPH identification, as supported by previous studies.
There are some limitations in this study. As PAH is divided into 7 subgroups (idiopathic PAH, heritable PAH, drug- and toxin-induced PAH, PAH associated with various conditions, PAH in long-term responders to calcium channel blockers, PAH with venous/capillary involvement, and persistent PH of the newborn), the sample size of PAH is relatively small. Thus, the study cohort cannot fully represent the pathophysiological changes in PAH. Therefore, further studies with larger sample sizes are warranted to validate the prognostic value of the larger-caliber pulmonary vessels in the identification of PAH from CTEPH. Meantime, the quantitative measurement of pulmonary vessels may be promising in terms of assessing the response to treatment in patients with PAH or CTEPH.
Conclusions
This study contributes valuable insights into the clinical implications of pulmonary blood flow redistribution in two typical pre-capillary PH, PAH and CTEPH patients. The right ventricle enlarged, and the volumes of the MPA and RPA were dilated in both PAH and CTEPH patients. However, the pulmonary vessel volume increased in PAH, especially in the larger-caliber vessels and pulmonary artery, whereas the pulmonary vessel volume decreased in CTEPH, especially in larger-caliber vessels and the RLL. Exploring the underlying mechanisms driving these differences may provide insights into pathophysiology and potential therapeutic targets.
Acknowledgments
Funding: This work was supported by
Footnote
Reporting Checklist: The authors have completed the STROBE reporting checklist. Available at https://qims.amegroups.com/article/view/10.21037/qims-24-644/rc
Conflicts of Interest: All authors have completed the ICMJE uniform disclosure form (available at https://qims.amegroups.com/article/view/10.21037/qims-24-644/coif). The authors have no conflicts of interest to declare.
Ethical Statement: The authors are accountable for all aspects of the work in ensuring that questions related to the accuracy or integrity of any part of the work are appropriately investigated and resolved. The study was conducted in accordance with the Declaration of Helsinki (as revised in 2013). The study was approved by the ethics committee of The First Affiliated Hospital of Xi’an Jiaotong University (No. 2013-114-1), and the requirement for written consent for this retrospective analysis was waived.
Open Access Statement: This is an Open Access article distributed in accordance with the Creative Commons Attribution-NonCommercial-NoDerivs 4.0 International License (CC BY-NC-ND 4.0), which permits the non-commercial replication and distribution of the article with the strict proviso that no changes or edits are made and the original work is properly cited (including links to both the formal publication through the relevant DOI and the license). See: https://creativecommons.org/licenses/by-nc-nd/4.0/.
References
- Humbert M, Kovacs G, Hoeper MM, Badagliacca R, Berger RMF, Brida M, et al. 2022 ESC/ERS Guidelines for the diagnosis and treatment of pulmonary hypertension. Eur Respir J 2023;61:2200879. [Crossref] [PubMed]
- Strange G, Stewart S, Celermajer DS, Prior D, Scalia GM, Marwick TH, Gabbay E, Ilton M, Joseph M, Codde J, Playford D, Contributing Sites NEDA. Threshold of Pulmonary Hypertension Associated With Increased Mortality. J Am Coll Cardiol 2019;73:2660-72. [Crossref] [PubMed]
- Strange G, Playford D, Stewart S, Deague JA, Nelson H, Kent A, Gabbay E. Pulmonary hypertension: prevalence and mortality in the Armadale echocardiography cohort. Heart 2012;98:1805-11. [Crossref] [PubMed]
- Zhai Z, Murphy K, Tighe H, Wang C, Wilkins MR, Gibbs JSR, Howard LS. Differences in ventilatory inefficiency between pulmonary arterial hypertension and chronic thromboembolic pulmonary hypertension. Chest 2011;140:1284-91. [Crossref] [PubMed]
- Rehman MB, Howard LS, Christiaens LP, Gill D, Gibbs JSR, Nihoyannopoulos P. Resting right ventricular function is associated with exercise performance in PAH, but not in CTEPH. Eur Heart J Cardiovasc Imaging 2018;19:185-92. [Crossref] [PubMed]
- Frost A, Badesch D, Gibbs JSR, Gopalan D, Khanna D, Manes A, Oudiz R, Satoh T, Torres F, Torbicki A. Diagnosis of pulmonary hypertension. Eur Respir J 2019;53:1801904. [Crossref] [PubMed]
- Chen Y, Shlofmitz E, Khalid N, Bernardo NL, Ben-Dor I, Weintraub WS, Waksman R. Right Heart Catheterization-Related Complications: A Review of the Literature and Best Practices. Cardiol Rev 2020;28:36-41. [Crossref] [PubMed]
- Nickel N, Golpon H, Greer M, Knudsen L, Olsson K, Westerkamp V, Welte T, Hoeper MM. The prognostic impact of follow-up assessments in patients with idiopathic pulmonary arterial hypertension. Eur Respir J 2012;39:589-96. [Crossref] [PubMed]
- Synn AJ, Margerie-Mellon C, Jeong SY, Rahaghi FN, Jhun I, Washko GR, Estépar RSJ, Bankier AA, Mittleman MA, VanderLaan PA, Rice MB. Vascular remodeling of the small pulmonary arteries and measures of vascular pruning on computed tomography. Pulm Circ 2021;11:20458940211061284. [Crossref] [PubMed]
- Rahaghi FN, Nardelli P, Harder E, Singh I, Sánchez-Ferrero GV, Ross JC, San José Estépar R, Ash SY, Hunsaker AR, Maron BA, Leopold JA, Waxman AB, San José Estépar R, Washko GR. Quantification of Arterial and Venous Morphologic Markers in Pulmonary Arterial Hypertension Using CT Imaging. Chest 2021;160:2220-31. [Crossref] [PubMed]
- Cajigas HR, Lavon B, Harmsen W, Muchmore P, Costa J, Mussche C, Pulsipher S, De Backer J. Quantitative CT measures of pulmonary vascular volume distribution in pulmonary hypertension associated with COPD: Association with clinical characteristics and outcomes. Pulm Circ 2023;13:e12321. [Crossref] [PubMed]
- Ash SY, Rahaghi FN, Come CE, Ross JC, Colon AG, Cardet-Guisasola JC, et al. Pruning of the Pulmonary Vasculature in Asthma. The Severe Asthma Research Program (SARP) Cohort. Am J Respir Crit Care Med 2018;198:39-50. [Crossref] [PubMed]
- Poletti J, Bach M, Yang S, Sexauer R, Stieltjes B, Rotzinger DC, Bremerich J, Walter Sauter A, Weikert T. Automated lung vessel segmentation reveals blood vessel volume redistribution in viral pneumonia. Eur J Radiol 2022;150:110259. [Crossref] [PubMed]
- Salerno D, Oriaku I, Darnell M, Lanclus M, De Backer J, Lavon B, Gupta R, Jaffe F, Vega Sanchez ME, Kim V. Temple University Covid-19 Research Group. Association of abnormal pulmonary vasculature on CT scan for COVID-19 infection with decreased diffusion capacity in follow up: A retrospective cohort study. PLoS One 2021;16:e0257892. [Crossref] [PubMed]
- Dokainish H, Nguyen JS, Bobek J, Goswami R, Lakkis NM. Assessment of the American Society of Echocardiography-European Association of Echocardiography guidelines for diastolic function in patients with depressed ejection fraction: an echocardiographic and invasive haemodynamic study. Eur J Echocardiogr 2011;12:857-64. [Crossref] [PubMed]
- Zhao W, Liu H, Leader JK, Wilson D, Meng X, Wang L, Chen LA, Pu J. Computerized identification of the vasculature surrounding a pulmonary nodule. Comput Med Imaging Graph 2019;74:1-9. [Crossref] [PubMed]
- Pu J, Fuhrman C, Good WF, Sciurba FC, Gur D. A differential geometric approach to automated segmentation of human airway tree. IEEE Trans Med Imaging 2011;30:266-78. [Crossref] [PubMed]
- Pu J, Leader JK, Zheng B, Knollmann F, Fuhrman C, Sciurba FC, Gur D. A Computational geometry approach to automated pulmonary fissure segmentation in CT examinations. IEEE Trans Med Imaging 2009;28:710-9. [Crossref] [PubMed]
- Pu J, Leader JK, Sechrist J, Beeche CA, Singh JP, Ocak IK, Risbano MG. Automated identification of pulmonary arteries and veins depicted in non-contrast chest CT scans. Med Image Anal 2022;77:102367. [Crossref] [PubMed]
- Docherty CK, Harvey KY, Mair KM, Griffin S, Denver N, MacLean MR. The Role of Sex in the Pathophysiology of Pulmonary Hypertension. Adv Exp Med Biol 2018;1065:511-28. [Crossref] [PubMed]
- Arshad HB, Duarte VE. Evaluation and Management of Pulmonary Arterial Hypertension in Congenital Heart Disease. Methodist Debakey Cardiovasc J 2021;17:145-51. [Crossref] [PubMed]
- Lee HJ, Wanderley M, Rubin VCDS, Rodrigues ACT, Diniz AR, Parga JR, Amato MBP. Lobar pulmonary perfusion quantification with dual-energy CT angiography: Interlobar variability and relationship with regional clot burden in pulmonary embolism. Eur J Radiol Open 2022;9:100428. [Crossref] [PubMed]
- Teerapuncharoen K, Bag R. Chronic Thromboembolic Pulmonary Hypertension. Lung 2022;200:283-99. [Crossref] [PubMed]
- Huang YS, Chen ZW, Lee WJ, Wu CK, Kuo PH, Hsu HH, Tang SY, Tsai CH, Su MY, Ko CL, Hwang JJ, Lin YH, Chang YC. Treatment Response Evaluation by Computed Tomography Pulmonary Vasculature Analysis in Patients With Chronic Thromboembolic Pulmonary Hypertension. Korean J Radiol 2023;24:349-61. [Crossref] [PubMed]
- Kroeger JR, Zöllner J, Gerhardt F, Rosenkranz S, Gertz RJ, Kerszenblat S, Pahn G, Maintz D, Bunck AC. Detection of patients with chronic thromboembolic pulmonary hypertension by volumetric iodine quantification in the lung-a case control study. Quant Imaging Med Surg 2022;12:1121-9. [Crossref] [PubMed]
- Barco S, Mavromanoli AC, Kreitner KF, Bunck AC, Gertz RJ, Ley S, Valerio L, Klok FA, Gerhardt F, Rosenkranz S, Konstantinides SV. Preexisting Chronic Thromboembolic Pulmonary Hypertension in Acute Pulmonary Embolism. Chest 2023;163:923-32. [Crossref] [PubMed]
- Yilmaz F, Tunçez A, Önner H, Gedik GK, Uysal E, Körez MK. Diagnostic compatibility of V/Q SPECT and CTPA, which are non-invasive diagnostic methods, for the detection of CTEPH, which is a treatable cause of pulmonary hypertension. Hell J Nucl Med 2022;25:168-76. [Crossref] [PubMed]
- Dong C, Zhou M, Liu D, Long X, Guo T, Kong X. Diagnostic accuracy of computed tomography for chronic thromboembolic pulmonary hypertension: a systematic review and meta-analysis. PLoS One 2015;10:e0126985. [Crossref] [PubMed]
- Shahin Y, Alabed S, Alkhanfar D, Tschirren J, Rothman AMK, Condliffe R, Wild JM, Kiely DG, Swift AJ, Quantitative CT. Evaluation of Small Pulmonary Vessels Has Functional and Prognostic Value in Pulmonary Hypertension. Radiology 2022;305:431-40. [Crossref] [PubMed]