Hemodynamic relationship with angioarchitecture of cerebral arteriovenous malformation and changes after embolization: a pilot study by 4D flow MR
Introduction
A cerebral arteriovenous malformation (cAVM) is a complex tangle of abnormal channels with pathological vascular connections between the arterial and venous system, and is an important factor in intracranial hemorrhage, especially in young adults (1). The heterogenous hemodynamics in cAVM is considered to play an important role in its pathophysiology but is difficult to assess by conventional morphology-based imaging or the widely used surgical grading such as the Spetzler-Martin grade (2,3). Previous techniques that measure the hemodynamics of cAVM have certain shortcomings and limitations. Computational fluid dynamics can be simulated from conventional angiography, but the obtained flow field uses geometric and hemodynamic boundary conditions that are less clearly defined (4). 4-dimentional (4D) contrast-enhanced magnetic resonance (MR) angiography can generate time of arrival maps and assist the display of cAVM but does not accurately quantify blood flow (5). Transcranial Doppler is readily used but has limited coverage of the intracranial vasculature, and is highly dependent on operator experience (6). Quantitative digital subtraction angiography (DSA) has high temporal and spatial resolution and calculates an index derived from the time-density curves of selected regions of interest, but is invasive and does not provide actual flow rates (7). 4D flow MR has enabled the noninvasive measurement of real flow in vivo. It was originally applied to the hemodynamics analysis of cardiac valve function and aortic diseases, demonstrating excellent ability in blood flow visualization and quantification (8,9). It has been increasingly used intracranially, showing great potential in the hemodynamic assessment of cAVM (10,11).
Several studies have attempted to reveal the association of hemodynamics with clinical presentation of cAVM, but the results were mixed due to heterogenous populations and varied modalities (12,13). Angioarchitecture such as arterial ectasia, intranidal aneurysm, venous ectasia, and venous stenosis, closely relates to the clinical presentation, hemorrhagic risk, and even complications of embolization of cAVM (14-16). However, the relationship between lesion angioarchitecture and hemodynamics has not been fully explored.
The treatment of cAVMs includes the combination of resection, embolization and/or radiotherapy. Surgical evacuation of the hematoma would be necessary in the setting of a ruptured cAVM with life-threatening mass effect. Embolization is especially considered for deep and diffused lesions, but the quantitative hemodynamic benefits remain difficult to predict. Changes in lesion size following gamma knife treatment may take years to be visible, but hemodynamic changes may occur sooner and could potentially be captured by 4D flow MR (17).
In summary, the aim of this study is to: (I) investigate the association between the angioarchitecture and flow-derived parameters by 4D flow MR imaging in cAVM; and (II) quantify the flow changes during follow-up after embolization and explore the potential of flow-guided subsequent embolization. We present this article in accordance with the STROBE reporting checklist (available at https://qims.amegroups.com/article/view/10.21037/qims-24-1150/rc).
Methods
Ethical statement
This prospective study was approved by the Institutional Review Board of Sichuan Provincial People’s Hospital (No. 2023515) and conformed to the provisions of the Declaration of Helsinki (as revised in 2013). Written informed consent was obtained from each participating patient.
Patient population
Consecutive patients with DSA-diagnosed cAVM were prospectively enrolled from Sichuan Provincial People’s Hospital from April 2022 to January 2024 for a cohort study. The inclusion criteria were: (I) unruptured lesion or ruptured with clinical Hunt-Hess grade I or II; (II) the patient was older than 18 years old; (III) lesion was untreated before. The exclusion criteria were: (I) the patient was experiencing acute intracranial hemorrhage due to reasons other than cAVM; (II) had other coexisting vasogenic abnormalities that might confound this study, including Moyamoya disease, traumatic or bacterial arterial dissection; (III) had an identified malignant intracranial tumor; (IV) had a contra-indication of magnetic resonance imaging (MRI), including with metal implants or claustrophobia; (V) had severe mental diseases that hampered necessary communication.
Baseline demographic and clinical data were collected from a formal medical record system. The information regarding baseline angioarchitecture was obtained from the DSA results and routinely evaluated by experienced neuroradiologists. The angioarchitecture analysis included six features: number of feeding arteries, deep vein drainage, intra-nidal aneurysm, dilated feeders (1.5 times greater in diameter than contralateral side), venous ectasia (2-fold caliber change in any draining venous channel), and stenosis of the main drainage vein (abrupt decreased venous diameter less than 50% of upstream venous diameter), with reference to the terminology provided by a joint committee led by the American Society of Interventional and Therapeutic Neuroradiology and a previous study (14,18). If there were multiple feeding arteries, the feeding artery measured with the highest flow by MR was recorded as the “main feeding artery”. Spetzler-Martin grade was recorded for each case.
MR image acquisition
Routine sequences including T1 and T2 weighted imaging and FLAIR were acquired. Time-of-flight magnetic resonance angiography (TOF-MRA) was performed to assess the anatomic structure of the lesion and for further segmentation. 4D flow MR, an radiofrequency (RF)-spoiled gradient echo sequence with short repetition time (TR) encoded on three spatial directions and temporal encoding through the cardiac cycle, was performed to assess the lesion hemodynamics. Technical details and protocols were as follows: a 3T scanner (MAGNETOM VIDA, Siemens Healthineers Ltd., Erlangen, Germany) equipped with a 20-channel head and neck coil was used. 4D flow with 3D volumetric coverage of the arteriovenous malformation (AVM) vasculature was applied with: velocity encoding sensitivity (VENC) =120 cm/s (19); TR/echo time (TR/TE) =65/5.12; flip angle =15°; voxel size =1.0 mm isotropic; field of view =176×164; phase =10–12; temporal resolution =65 ms. The patients were trained to maintain a steady breath before the scan. Prospective electrocardiogram (ECG) gating was used to perform scanning under free breathing. The technique of parallel imaging reconstruction of GeneRalized Autocalibrating Partially Parallel Acquisitions (GRAPPA) was employed with an acceleration factor of two. The acquisition time was 15–20 minutes depending on the heart rate of the patient during the scan.
4D flow data processing and flow quantification
The MR data processing was performed with blinding to the clinical information and DSA results of the patient. A certified radiologist with 12 years of experience (Yuting Wang) identified the target anatomy of the lesion and a doctoral scientist with 3 years of experience (H.W.) processed the flow data. The workflow reported by Fu et al. was used to obtain quantitative values, in which the interobserver reliability was validated (20). The workflow is shown in Figure 1 and an illustrative result format is shown in Figure 2. The 3D model of cAVM was generated by threshold segmentation, connected domain selection and surface smoothing from registered TOF imaging. The 3D velocity field calculated from 4D flow MRI was used for extracting hemodynamic parameters. Hemodynamic parameters included the flow and velocity of the feeding artery and draining vein were calculated by volume of interest (VOI) measurement box. Mean flow over the cardiac cycle was measured. According to the consensus of 4D flow, conventional flow parameters can be obtained at any location in the data volume where the employed parameter settings provide sufficient accuracy (21). As shown in Figure 2, the VOI measurement box contains a red flow measurement plane. When adjusting the measurement box, the measurement plane was perpendicular to the vascular centerline, where the 3D vessel model was relatively complete and close to the nidus. The red plane cut the vessel model and calculated the flow through the vessel section. The workflow program calculated the velocity in the VOI, which contained about 5 mm of blood vessels. We avoided setting the measurement box in regions with significant noise. For advanced hemodynamic parameters, we calculated wall shear stress (WSS) of the feeding artery adjacent to the nidus. The time averaged WSS was calculated using the average of obtained value at all timepoints during a cardiac cycle. Pulsatility index (PI) and resistive index (RI) were calculated using the following equations: PI = (peak systolic velocity − minimal diastolic velocity)/(mean velocity), RI = (peak systolic velocity − minimal diastolic velocity)/(peak velocity) (22,23). Inflow ratio was defined as the flow of the feeding artery divided by the nidus volume. Outflow ratio was defined as the flow of the draining vein divided by the nidus volume. Inflow-to-outflow ratio was defined as the flow of all feeding arteries divided by the flow of the draining vein (24). After cutting out the interferential vessels, the nidus was selected by the VOI measurement box and the volume of the vessels within the box were calculated.
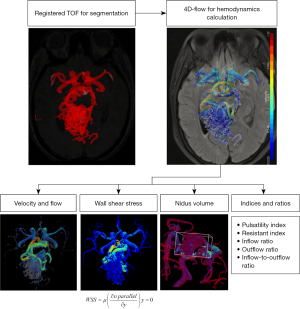
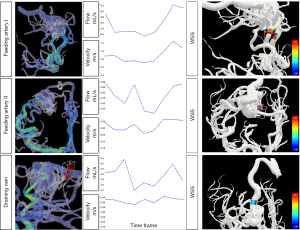
Patient management
Multi-discipline evaluation (including neurosurgeons, neurointerventional radiologists, and neuroradiologists) was conducted to decide whether treatment should be craniotomy and resection, interventional embolization, Gamma Knife radiosurgery or conservative strategies based on the Spetzler-Martin grade of the lesion, the estimated difficulty of the interventional approach according to pre-operative imaging, and the clinical severity of the patient, with consideration of the multi-discipline expert consensus published in the Asian Journal of Neurosurgery (25).
Considering the possibility of staged embolization, patients who underwent embolization were referred for a follow-up MRI scan 6–12 months after the procedure, or if any obvious symptom arose before that timepoint (17,19). Neurointerventional radiologists decided whether to proceed to a second embolization based on the follow-up imaging data.
Statistical analysis
Continuous variables were summarized as means ± standard deviation (SD) if normally distributed, or median (range or interquartile range) if otherwise. Categorical variables were presented as percentages. Data were assessed for normality by using the Shapiro-Wilk test. For continuous variables, comparisons between groups were conducted using t-test or analysis of variance if normally distributed, and Mann-Whitney U test or Kruskal-Wallis test if not normally distributed. Comparisons between groups were conducted using Chi-squared test or Fisher’s exact test for categorical variables. Correlations between flow and multiple flow-derived advanced parameters were assessed by Pearson correlations. Statistical analyses were performed using SPSS version 26.0 (IBM Corp., Chicago, IL, USA). A P<0.05 (two-sided) was considered statistically significant.
Results
Demographics and angioarchitecture
A total of 28 patients were initially included for MRI studies. Five cases were excluded as no significant nidus remained after a prior embolization, 2 were excluded for having diagnosis other than cAVM (one for arteriovenous fistula, one for cavernous hemangioma). Finally, this cohort included 21 patients with confirmed cAVM (age range 19–61 years with a median age of 37 years old, 9 females). Demographics and clinical characteristics of the patients with cAVM are summarized in Table 1. Feeding by multiple arteries was strongly associated with unruptured status (1/7 in ruptured group and 14/14 in unruptured group, P<0.001). Intra-nidal aneurysm presence was significantly associated with lesion rupture (7/7 in ruptured group and 5/14 in unruptured group, P=0.007). The other features of angioarchitecture were not associated with rupture status, and the rupture status was not related to the Spetzler-Martin grade (P=0.362). Among the angioarchitectural features, feeding by a single artery was significantly associated with presentation of an intra nidal aneurysm (P=0.017).
Table 1
Characteristics | Unruptured (N=14) | Ruptured (N=7) | P value |
---|---|---|---|
Female | 4 | 5 | 0.062 |
Age, years | 37 [19–61] | 53 [29–58] | 0.605 |
Deep vein drainage | 6 (42.9) | 5 (71.4) | 0.374 |
Multiple feeding arteries | 14 (100.0) | 1 (14.3) | <0.001 |
Intra nidal aneurysm | 5 (35.7) | 7 (100.0) | 0.007 |
Dilated feeders | 14 (100.0) | 5 (71.4) | 0.111 |
Venous ectasia | 14 (100.0) | 6 (85.7) | 0.350 |
Stenosis of the main drainage vein | 1 (7.1) | 3 (42.8) | 0.101 |
Spetzler-Martin grade | 0.362 | ||
I | 0 | 1 | |
II | 5 | 4 | |
III | 5 | 1 | |
IV | 4 | 1 |
Data are presented as n, n (%), or median [range].
Angioarchitecture and hemodynamics
The lesions fed by multiple arteries showed higher total flow of feeding arteries (P=0.017), higher mean flow of the main feeding artery (P=0.017), and higher time averaged WSS (P=0.007). The lesions with dilated feeders showed higher PI and RI of the feeding artery (P=0.042, 0.032, respectively), and higher mean flow (P=0.044). The hemodynamic parameters showed no significant differences between lesions distinguished by the other different angioarchitectural features (all P>0.05), as shown in Table 2 (in three cases, segmentation of the draining veins from the 4D flow study could not be performed because of low MR signal strength, thus their vein-related index could not be utilized).
Table 2
Variables | Group | Dilated feeders | Multiple feeding arteries | Intra nidus aneurysm | Deep vein drainage | Whole group |
---|---|---|---|---|---|---|
Total flow, mL/s | Yes | 5.15±3.89 | 5.93±3.63* | 4.59±5.03 | 4.20±4.62 | 4.62±3.87 |
No | 0.27±0.19 | 1.71±3.27 | 4.78±1.75 | 5.23±3.19 | ||
Mean flow of FAm, mL/s | Yes | 3.23 (4.24)* | 3.46 (3.78)* | 1.37 (7.55) | 3.04 (3.64) | 3.46±3.08 |
No | 0.27 (0.26) | 0.27 (2.79) | 3.46 (1.41) | 3.11 (5.37) | ||
Peak velocity of FAm, cm/s | Yes | 52.0±19.8 | 55.9±14.2 | 49.7±23.9 | 51.0±23.4 | 51.6±19.7 |
No | 39.6±23.6 | 38.6±26.9 | 52.4±12.8 | 50.4±15.8 | ||
WSS, Pa | Yes | 1.98±0.77 | 2.21±0.58** | 2.00±1.03 | 2.04±1.08 | 1.98±0.76 |
No | 0.66§ | 0.99±0.73 | 1.97±0.46 | 1.92±0.27 | ||
Pulsatility index | Yes | 0.33±0.12* | 0.32±0.92 | 0.38±0.16* | 0.39±0.17* | 0.35±0.13 |
No | 0.54±0.15 | 0.43±0.20 | 0.32±0.09 | 0.31±0.08 | ||
Resistive index | Yes | 0.28±0.90* | 0.27±0.07 | 0.31±0.12* | 0.32±0.12* | 0.29±0.09 |
No | 0.44±0.11 | 0.35±0.15 | 0.27±0.06 | 0.26±0.07 |
*, P<0.05; **, P<0.01, for comparisons between “Yes” (group with this feature) and “No” (group without this feature). Data are presented as mean ± standard deviation or median (interquartile range). §, only one cases in that group (the other case was excluded because of technical difficulty of measurement due to the noise). cAVM, cerebral arteriovenous malformation; FAm, feeding artery with the maximal flow; WSS, wall shear stress.
As shown in Table 3, the ruptured group exhibited lower mean flow of the main feeding artery and total flow (P=0.019, 0.029, respectively), lower mean and total flow of the draining vein (P=0.012, 0.047, respectively), lower WSS of the main feeding artery (P=0.023), and higher inflow-to-outflow ratio (P=0.041). Meanwhile, there was considerable overlap between the hemodynamics of lesions with different Spetzler-Martin grades. Except the grade III group, which showed greater total flow of draining veins (P=0.003), there were no significant differences in any other flow-derived parameters among grade II to IV.
Table 3
Hemodynamic parameters | Unruptured (N=14) | Ruptured (N=7) | P value |
---|---|---|---|
Mean velocity of FAm, cm/s | 49.3±16.2 | 34.3±21.2 | 0.093 |
Peak velocity of Fam, cm/s | 55.5±14.6 | 41.9±26.0 | 0.146 |
Mean flow of FAm, mL/s | 3.56 (3.96) | 0.40 (2.91) | 0.019 |
Total flow of all FA, mL/s | 6.05±3.76 | 2.09±3.15 | 0.029 |
Pulsatility index | 0.32±0.09 | 0.42±0.19 | 0.112 |
Resistent index | 0.27±0.07 | 0.34±0.14 | 0.103 |
WSS, Pa | 2.10±0.45 | 0.99±0.73 | 0.023 |
Mean velocity of DVm, cm/s | 20.1±5.8 | 16.0±5.2 | 0.236 |
Mean flow of DVm, mL/s | 3.48±1.64 | 0.94±0.93 | 0.012 |
Total flow of all DV, mL/s | 4.14±2.54 | 1.20±1.32 | 0.047 |
Inflow ratio | 1.09±0.51 | 1.43±0.47 | 0.394 |
Outflow ratio | 0.70±0.16 | 0.64±0.40 | 0.706 |
Inflow-to-outflow ratio | 1.46±0.55 | 2.46±0.79 | 0.041 |
Data are presented as mean ± standard deviation or median (interquartile range). cAVM, cerebral arteriovenous malformation; FAm, feeding artery with the maximal flow; FA, feeding artery; WSS, wall shear stress; DVm, draining vein with the maximal flow; DV, draining vein.
Correlation among hemodynamic parameters
The flow indices and velocity indices were highly positively correlated (P<0.001). Time averaged WSS positively correlated with velocity of the feeding artery (P=0.02), and with inflow ratio (P=0.01), but was not correlated with the mean flow of the main feeding artery or the total flow. PI and RI were highly positively correlated (P<0.001).
Hemodynamic changes during follow-up after treatment
Two patients had the lesion resected at craniotomy, 10 patients had successful embolization, one had a failed embolization due to inability of super-selection using a microcatheter. Two other patients received embolization after craniotomy, one patient had Gamma Knife radiosurgery, and five patients with unruptured lesions were offered the option of embolization but refused further intervention and followed with conservative strategies.
Among the patients who had successful embolization, seven patients reached the timepoint of reevaluation and had a follow-up 4D flow MR scan with a median interval of 8.9 months (range, 3.4–11.8 months). The embolic material appeared to be hypointense on MR images, and artifacts induced by embolic material were not observed in this study. The total flow of the lesions decreased by 10–100% after the embolization (details in Table S1). For the lesions fed by multiple arteries, the main feeding artery exhibited reduction of the mean flow (reduction rate: median 86.6%, range, 19.5–100%), and decrease in velocity (reduction rate: median 61.2%, range, 4.5–100% for mean velocity, median 51.8%, range, 1.2–100% for peak velocity, respectively). A demonstrative case is presented in Figure 3, in which the reduction of the arterial size was not as prominent as the flow reduction. Meanwhile, the velocity of the minor feeding artery increased in 3 cases out of 5, and the flow of the minor feeding artery increased in 2 cases out of 5. The nidus volume was reduced significantly (reduction rate: 59.6–100%, P=0.009), and the WSS of the main feeding artery decreased significantly too (reduction rate: 9.3–100%, P=0.037). The PI and RI of the main feeding artery, however, showed a slight tendency to increase (in 5 of 6 cases for which the index could be measured, since the artery was not visible in one case).
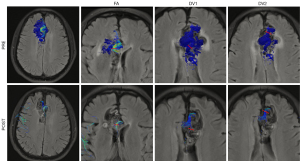
Three patients had a second embolization guided by the follow-up imaging data, including the patient with the least decrease in velocity and flow (4.5% decrease in the mean velocity of the main feeding artery and 10% decrease in the total flow). In that case, although there was significant reduction in nidus volume (75.2%), the main feeding artery was selectively embolized by a second procedure to achieve the maximal hemodynamic benefit. One patient had three timepoints of 4D flow MR data (at admission, 5 months after the first embolization and before a second embolization, and 3 months after the second intervention). The total flow decreased by 40.1% at the second timepoint and by 90.1% at the third timepoint. The nidus volume dropped from 6,449 to 2,605 mm3, and finally became no longer visible (Figure 4).
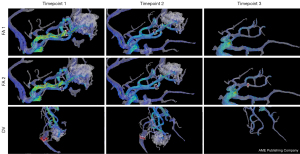
Discussion
The hemodynamics of cAVM is believed to contribute to the pathophysiology and clinical presentation (26). Nevertheless, the hemodynamics is complex and likely varies with lesion angioarchitecture (24). In this pilot study, successful intracranial flow evaluation by 4D flow MR was achieved with image quality sufficient for subsequent post processing, and the complicated feeding/draining pattern of AVM could be visualized. With the current measurements, the inflow-to-outflow ratio and outflow ratio quantified the imbalance between inflow and outflow after cAVM rupture, while the mere status of deep vein drainage did not provide any related information. The follow-up flow evaluation quantified the hemodynamic change on an individual basis.
The role of angioarchitecture
Angioarchitectural features have been widely reported in studies of cAVM (13,27). Unexpectedly, supply by a single feeding artery, has been shown to be strongly associated with the ruptured status of cAVM. Although presence of an intra-nidal aneurysm seems important, it is quite difficult to demonstrate on MRA (in this cohort, only five cases showed the aneurysm on MRA of the 12 cases in which the DSA found the aneurysm). Nevertheless, single artery supply was shown to be strongly associated with an intra-nidal aneurysm, which should raise clinical awareness during the scan reading, even without the acquisition of 4D flow. Only very few features of angioarchitecture showed association with the hemodynamic indexes of cAVM, indicating that lesion hemodynamics could not be easily determined or predicted by simple geometric features (19).
Comparison with prior studies, and interactions between the hemodynamic parameters of cAVM
Two prior studies (Chang et al. with 10 patients and Wu et al. with 17 patients) evaluated cAVM by 4D flow and reported the flow or WSS differences between feeding arteries and contralateral normal arteries (13,28). In this study, more comprehensive flow parameters were explored and compared between groups. Unexpectedly, the flow of ruptured lesions exhibited lower flow than unruptured ones, but a greater imbalance between the inflow and outflow (higher inflow-to-outflow ratio). Previous studies commonly inferred that the imbalanced hemodynamics in AVMs caused venous congestion, resulting in rupture of the AVMs (7,24). A more recent study retrospectively compared more flow parameters (including inflow-to-outflow ratio) between ruptured and unruptured cAVM (24). The significance of intra-nidal aneurysm, inflow-to-outflow ratio and insignificance of deep vein drainage status were all confirmed by this prospective study. In addition, we investigated more flow parameters including WSS, evaluated correlations between them, and explored the hemodynamic changes/remodeling during follow-up for staged embolization.
The correlations and interactions between the hemodynamic parameters could provide insights into the underlying mechanism of cAVM rupture. It is noteworthy that ruptured cases showed lower WSS of the main feeding artery. Multiple studies have reported that in intracranial aneurysms unassociated with cAVM, lower WSS of the aneurysm wall is associated with aneurysm rupture (29,30). There are also variations in distribution of WSS throughout the vasculature (31). Furthermore, it has been reported that higher WSS activates vascular remodeling and angiogenesis in cAVM (2). Although the feeding artery is not likely the rupture point, whether its lowered WSS is the trigger or consequence of cAVM rupture is worthy of further validation. In addition, it is noteworthy that the PI and RI of the feeding artery showed a tendency to increase during follow-up in this cohort. The increase of the relative pulsatile flow in the resistance part would indicate a decreased compliance according to the two-element Windkessel model, which also warrants further investigation (11).
The study by Ansari et al. reported heterogeneous flow even with the same Spetzler-Martin grade (19), However, the study by Wu et al. found that the Spetzler-Martin grade correlated with cumulative flow of the feeding artery and draining vein (13). It was verified in this cohort that the diverse and complex hemodynamics of AVM could not be reflected by the surgical Spetzler-Martin grading, and that the individualized hemodynamic analysis provided an irreplaceable role in cAVM evaluation.
Potential of hemodynamic evaluation by 4D flow to guide staged embolization
Although DSA is usually performed in the preoperative evaluation to more accurately map the lesion angioarchitecture, it provides limited quantification of flow dynamics. The embolization-induced changes on hemodynamics of cAVM remain difficult to predict even if feeding arteries can be clearly identified (19). A non-invasive examination is more suitable for repeated evaluations for staged embolization. Quantitative measures in this study showed that hemodynamics stabilized months after the procedure with considerable remodeling of the flow pattern, which would be otherwise difficult to know and might require further surveillance. The flow-guided second embolization revealed the potential of 4D flow MR to maximally utilize the knowledge of lesion hemodynamics in the clinical setting. Case reports have measured flow changes using 4D flow MR during stereotactic radiosurgery (17,32). Another study reported four cases followed by 4D flow MR during staged embolization but did not mention the interval for the stabilization of the hemodynamics or the guiding role of flow data for a second embolization (19).
Limitations
There were several limitations to this study. A major limitation is the relatively small sample size. Although this is a prospective study of a very rare disease with a comparable or greater number of cases compared to retrospective studies in this area, it remains a pilot study and the generalizability of the conclusion remains to be further validated. Second, there were several technical challenges. In three cases it was impossible to extract flow data for the draining veins because of low signal. This is likely because the study used a single setting for the VENC—the flow sensitization parameter. Given a certain VENC, vessels with flow signal below the measurement threshold (typically small veins) would not be captured. A dual VENC technique could be of great potential to evaluate lesions with heterogeneous hemodynamics (both high and low flow) like cAVM, and the measurement of vein-related parameters like inflow-to-outflow ratio would be more reliable (33). However, that technique is not widely available. Another technical challenge for intracranial 4D flow is the limited resolution compared with 4D flow of the heart and aorta. It was difficult to segment or quantify small vessels (e.g., with diameters <3 mm), in which cases the reliability of measurements could be limited. Higher resolution would require longer scan times. This could possibly be addressed with advanced acceleration techniques in future studies. In addition, an improved post contrast MRA would help define the anatomy. It also provides better image quality for post contrast 4D flow. Fourth, noise of the acquired images remains a limitation of the measurement technique. In data processing, we did not use filters and limited minmax values to process speed information to avoid unvalidated manual intervention. Although the obvious noise during the measurement was avoided, there could still be some noise affecting the measurement results. Finally, although the flow analysis indicates a potential role for 4D flow in guiding a second embolization, this potential is merely descriptive, and this study is not a randomized double-armed study to compare strategies with or without the guidance of flow data. Given the rarity of the disease, a future multi-center study would be of great value to improve interventional planning and post-embolization monitoring.
Conclusions
cAVM lesions tend to have heterogeneous hemodynamics even with the same Spetzler-Martin grade or similar angioarchitecture. This could probably be reflected and quantified on an individual basis using 4D flow MR. Furthermore, follow-up scans months after the embolization showed a varied rate of flow reduction of cAVM along with flow remodeling. Flow analysis by MR exhibited the potential to guide a second embolization by providing the quantitative extent of flow reduction and remodeling after the first embolization.
Acknowledgments
The authors thank Prof. David Saloner and Dr. Evan Kao from University of California San Francisco for helping revising the language of the manuscript.
Funding: This work was supported by
Footnote
Reporting Checklist: The authors have completed the STROBE reporting checklist. Available at https://qims.amegroups.com/article/view/10.21037/qims-24-1150/rc
Conflicts of Interest: All authors have completed the ICMJE uniform disclosure form (available at https://qims.amegroups.com/article/view/10.21037/qims-24-1150/coif). Yuting Wang reports grants from the National Natural Science Foundation of China (Youth Project) (grant No. 82202123) and Sichuan Provincial Natural Science Foundation (Youth Project) (grant No. 2022NSFSC1473), during the conduct of the study. The other authors have no conflicts of interest to declare.
Ethical Statement: The authors are accountable for all aspects of the work in ensuring that questions related to the accuracy or integrity of any part of the work are appropriately investigated and resolved. This prospective study was approved by the Institutional Review Board of Sichuan Provincial People’s Hospital (No. 2023515) and conformed to the provisions of the Declaration of Helsinki (as revised in 2013). Written informed consent was obtained from each participating patient.
Open Access Statement: This is an Open Access article distributed in accordance with the Creative Commons Attribution-NonCommercial-NoDerivs 4.0 International License (CC BY-NC-ND 4.0), which permits the non-commercial replication and distribution of the article with the strict proviso that no changes or edits are made and the original work is properly cited (including links to both the formal publication through the relevant DOI and the license). See: https://creativecommons.org/licenses/by-nc-nd/4.0/.
References
- Fleetwood IG, Steinberg GK. Arteriovenous malformations. Lancet 2002;359:863-73. [Crossref] [PubMed]
- Chen W, Choi EJ, McDougall CM, Su H. Brain arteriovenous malformation modeling, pathogenesis, and novel therapeutic targets. Transl Stroke Res 2014;5:316-29. [Crossref] [PubMed]
- Spetzler RF, Martin NA. A proposed grading system for arteriovenous malformations. J Neurosurg 1986;65:476-83. [Crossref] [PubMed]
- Kamada H, Nakamura M, Ota H, Higuchi S, Takase K. Blood flow analysis with computational fluid dynamics and 4D-flow MRI for vascular diseases. J Cardiol 2022;80:386-96. [Crossref] [PubMed]
- Schubert T, Wu Y, Johnson KM, Wieben O, Maksimovic J, Mistretta C, Turski P. Time-of-Arrival Parametric Maps and Virtual Bolus Images Derived From Contrast-Enhanced Time-Resolved Radial Magnetic Resonance Angiography Improve the Display of Brain Arteriovenous Malformation Vascular Anatomy. Invest Radiol 2016;51:706-13. [Crossref] [PubMed]
- Lim EY, Yang DW, Cho AH, Shim YS. Cerebrovascular Hemodynamics on Transcranial Doppler Ultrasonography and Cognitive Decline in Mild Cognitive Impairment. J Alzheimers Dis 2018;65:651-7. [Crossref] [PubMed]
- Lin TM, Yang HC, Lee CC, Wu HM, Hu YS, Luo CB, Guo WY, Kao YH, Chung WY, Lin CJ. Stasis index from hemodynamic analysis using quantitative DSA correlates with hemorrhage of supratentorial arteriovenous malformation: a cross-sectional study. J Neurosurg 2020;132:1574-82. [Crossref] [PubMed]
- Riesenkampff E, Fernandes JF, Meier S, Goubergrits L, Kropf S, Schubert S, Berger F, Hennemuth A, Kuehne T. Pressure fields by flow-sensitive, 4D, velocity-encoded CMR in patients with aortic coarctation. JACC Cardiovasc Imaging 2014;7:920-6. [Crossref] [PubMed]
- Rizk J. 4D flow MRI applications in congenital heart disease. Eur Radiol 2021;31:1160-74. [Crossref] [PubMed]
- Liu J, Koskas L, Faraji F, Kao E, Wang Y, Haraldsson H, Kefayati S, Zhu C, Ahn S, Laub G, Saloner D. Highly accelerated intracranial 4D flow MRI: evaluation of healthy volunteers and patients with intracranial aneurysms. MAGMA 2018;31:295-307. [Crossref] [PubMed]
- Holmgren M, Wåhlin A, Dunås T, Malm J, Eklund A. Assessment of Cerebral Blood Flow Pulsatility and Cerebral Arterial Compliance With 4D Flow MRI. J Magn Reson Imaging 2020;51:1516-25. [Crossref] [PubMed]
- Shakur SF, Liesse K, Amin-Hanjani S, Carlson AP, Aletich VA, Charbel FT, Alaraj A. Relationship of Cerebral Arteriovenous Malformation Hemodynamics to Clinical Presentation, Angioarchitectural Features, and Hemorrhage. Neurosurgery 2016;63:136-40. [Crossref] [PubMed]
- Wu C, Ansari SA, Honarmand AR, Vakil P, Hurley MC, Bendok BR, Carr J, Carroll TJ, Markl M. Evaluation of 4D vascular flow and tissue perfusion in cerebral arteriovenous malformations: influence of Spetzler-Martin grade, clinical presentation, and AVM risk factors. AJNR Am J Neuroradiol 2015;36:1142-9. [Crossref] [PubMed]
- Lin CJ, Chen KK, Hu YS, Yang HC, Lin CF, Chang FC. Quantified flow and angioarchitecture show similar associations with hemorrhagic presentation of brain arteriovenous malformations. J Neuroradiol 2023;50:79-85. [Crossref] [PubMed]
- Li R, Chen P, Han H, Li Z, Chen X, Chen Y, Zhao Y. Association of nidus size and rupture in brain arteriovenous malformations: Insight from angioarchitecture and hemodynamics. Neurosurg Rev 2023;46:216. [Crossref] [PubMed]
- Pan J, He H, Feng L, Viñuela F, Wu Z, Zhan R. Angioarchitectural characteristics associated with complications of embolization in supratentorial brain arteriovenous malformation. AJNR Am J Neuroradiol 2014;35:354-9. [Crossref] [PubMed]
- Li CQ, Hsiao A, Hattangadi-Gluth J, Handwerker J, Farid N. Early Hemodynamic Response Assessment of Stereotactic Radiosurgery for a Cerebral Arteriovenous Malformation Using 4D Flow MRI. AJNR Am J Neuroradiol 2018;39:678-81. [Crossref] [PubMed]
- Atkinson RP, Awad IA, Batjer HH, Dowd CF, Furlan A, et al. Reporting terminology for brain arteriovenous malformation clinical and radiographic features for use in clinical trials. Stroke 2001;32:1430-42. [Crossref] [PubMed]
- Ansari SA, Schnell S, Carroll T, Vakil P, Hurley MC, Wu C, Carr J, Bendok BR, Batjer H, Markl M. Intracranial 4D flow MRI: toward individualized assessment of arteriovenous malformation hemodynamics and treatment-induced changes. AJNR Am J Neuroradiol 2013;34:1922-8. [Crossref] [PubMed]
- Fu M, Peng F, Zhang M, Chen S, Niu H, He X, Xu B, Liu A, Li R. Aneurysmal wall enhancement and hemodynamics: pixel-level correlation between spatial distribution. Quant Imaging Med Surg 2022;12:3692-704. [Crossref] [PubMed]
- Dyverfeldt P, Bissell M, Barker AJ, Bolger AF, Carlhäll CJ, Ebbers T, Francios CJ, Frydrychowicz A, Geiger J, Giese D, Hope MD, Kilner PJ, Kozerke S, Myerson S, Neubauer S, Wieben O, Markl M. 4D flow cardiovascular magnetic resonance consensus statement. J Cardiovasc Magn Reson 2015;17:72. [Crossref] [PubMed]
- van Hespen KM, Kuijf HJ, Hendrikse J, Luijten PR, Zwanenburg JJM. Blood Flow Velocity Pulsatility and Arterial Diameter Pulsatility Measurements of the Intracranial Arteries Using 4D PC-MRI. Neuroinformatics 2022;20:317-26. [Crossref] [PubMed]
- Naesens M, Heylen L, Lerut E, Claes K, De Wever L, Claus F, Oyen R, Kuypers D, Evenepoel P, Bammens B, Sprangers B, Meijers B, Pirenne J, Monbaliu D, de Jonge H, Metalidis C, De Vusser K, Vanrenterghem Y. Intrarenal resistive index after renal transplantation. N Engl J Med 2013;369:1797-806. [Crossref] [PubMed]
- Takeda Y, Kin T, Sekine T, Hasegawa H, Suzuki Y, Uchikawa H, Koike T, Kiyofuji S, Shinya Y, Kawashima M, Saito N. Hemodynamic Analysis of Cerebral AVMs with 3D Phase-Contrast MR Imaging. AJNR Am J Neuroradiol 2021;42:2138-45. [Crossref] [PubMed]
- Kato Y, Dong VH, Chaddad F, Takizawa K, Izumo T, Fukuda H, Hara T, Kikuta K, Nakai Y, Endo T, Kurita H, Xu B, Beneš V, Christian R, Pavesi G, Hodaie M, Sharma RK, Agarwal H, Mohan K, Liew BS. Expert Consensus on the Management of Brain Arteriovenous Malformations. Asian J Neurosurg 2019;14:1074-81. [Crossref] [PubMed]
- Fennell VS, Martirosyan NL, Atwal GS, Kalani MYS, Ponce FA, Lemole GM Jr, Dumont TM, Spetzler RF. Hemodynamics Associated With Intracerebral Arteriovenous Malformations: The Effects of Treatment Modalities. Neurosurgery 2018;83:611-21. [Crossref] [PubMed]
- Stefani MA, Porter PJ, terBrugge KG, Montanera W, Willinsky RA, Wallace MC. Large and deep brain arteriovenous malformations are associated with risk of future hemorrhage. Stroke 2002;33:1220-4. [Crossref] [PubMed]
- Chang W, Loecher MW, Wu Y, Niemann DB, Ciske B, Aagaard-Kienitz B, Kecskemeti S, Johnson KM, Wieben O, Mistretta C, Turski P. Hemodynamic changes in patients with arteriovenous malformations assessed using high-resolution 3D radial phase-contrast MR angiography. AJNR Am J Neuroradiol 2012;33:1565-72. [Crossref] [PubMed]
- Zhou G, Zhu Y, Yin Y, Su M, Li M. Author Correction: Association of wall shear stress with intracranial aneurysm rupture: systematic review and meta-analysis. Sci Rep 2018;8:5244. [Crossref] [PubMed]
- Brunozzi D, Theiss P, Andrews A, Amin-Hanjani S, Charbel FT, Alaraj A. Correlation Between Laminar Wall Shear Stress and Growth of Unruptured Cerebral Aneurysms: In Vivo Assessment. World Neurosurg 2019;131:e599-605. [Crossref] [PubMed]
- Bai X, Fu M, Li Z, Gao P, Zhao H, Li R, Sui B. Distribution and regional variation of wall shear stress in the curved middle cerebral artery using four-dimensional flow magnetic resonance imaging. Quant Imaging Med Surg 2022;12:5462-73. [Crossref] [PubMed]
- Srinivas S, Retson T, Simon A, Hattangadi-Gluth J, Hsiao A, Farid N. Quantification of hemodynamics of cerebral arteriovenous malformations after stereotactic radiosurgery using 4D flow magnetic resonance imaging. J Magn Reson Imaging 2021;53:1841-50. [Crossref] [PubMed]
- Aristova M, Vali A, Ansari SA, Shaibani A, Alden TD, Hurley MC, Jahromi BS, Potts MB, Markl M, Schnell S. Standardized Evaluation of Cerebral Arteriovenous Malformations Using Flow Distribution Network Graphs and Dual-venc 4D Flow MRI. J Magn Reson Imaging 2019;50:1718-30. [Crossref] [PubMed]