Assessment of cerebral perfusion alterations in dementia with Lewy bodies and Alzheimer’s disease
Introduction
Dementia with Lewy bodies (DLB) is the second most prevalent form of dementia after Alzheimer’s disease (AD). The main clinical features of DLB are fluctuating cognitive dysfunction, visual hallucinations, and resting tremor (1). AD is a progressive disease in the elderly, with a slow onset, mainly characterized by loss of memory, cognitive function, and daily social interaction. Conversely, DLB at the early stage may have no significant cognitive changes (2). Moreover, patients with advanced DLB may present with AD symptoms such as weakness in visual memory, short-term memory, and so on, making the diagnosis of DLB difficult to establish (1,3-5). AD neuropathological changes are common in individuals with a clinical diagnosis of DLB, and a large study has shown that the majority of DLB patients (>70%) have AD neuropathological changes (6). The clinical manifestations and pathological underpinnings of DLB are multifaceted, exhibiting similarities with those of AD. Consequently, the diagnosis and differential diagnosis of these conditions pose significant challenges.
Recent studies have shown that blood-brain barrier (BBB) dysfunction also plays an important role in the onset and progression of dementia (7-11). These processes are intricately associated with brain activity and energy consumption. One important technique for the evaluation of neural activity in brain tissue is arterial spin labeling (ASL), a non-invasive, ubiquitously available, and radiation-free magnetic resonance imaging (MRI) technique (12,13). ASL measures cerebral blood flow (CBF) by using magnetically-labeled arterial blood flow as the endogenous contrast agent and the pseudo-continuous ASL (pcASL) allows for the acquisition of high signal-to-noise images by using a large string of short pulses of approximately 1 ms in length to form a pulse sequence of 1–2 seconds in length (14). The pcASL technique uses multiple post-labeling delay (PLD) times to calculate arterial transit time (ATT), offers superior quantification of blood perfusion, and improves the precision of mean CBF (mCBF) measurements (15). Previous studies have shown that ASL-MRI could demonstrate comparable regional perfusion patterns to those employing single photon emission computed tomography (SPECT) in AD (16-18). Similarly, ASL studies have yielded comparable results in DLB (19,20).
Reductions in CBF have been found in both DLB and AD. Several studies have reported that in AD, CBF changes mainly occur in the temporal parietal regions (21) and posterior cingulate gyrus (22,23). Compared with AD, studies have shown hypoperfusion in the temporal parietal (24), frontal lobe, posterior cingulate, medial, and lateral occipital lobes (25) in DLB (26,27). Furthermore, DLB exhibits a lack of occipital perfusion (28,29) and relative preservation of perfusion in the medial temporal lobe (30); CBF has been observed to be decreased throughout the brain (31). One study revealed CBF reduction in the frontal, insular, and temporal cortices in DLB, whereas decreased perfusion in the parietal and temporal cortices was observed in AD (27).
With the increase of age or the presence of vascular pathology, the ATT is prolongated, which reflects the extended time between the labeling of blood and its arrival in brain tissue. Few previous studies have reported ATT changes in DLB and AD. In an early study, Yoshiura et al. (32) suggested regions of localized zones of hypoperfusion in AD independent of prolongation of the regional ATT, speculating that the mechanism of hypoperfusion in AD differs from cerebrovascular disease. One study (33) reported that regional ATT of AD in the left inferior frontal gyrus and cingulate gyrus was prolonged, and ATT was correlated with mini-mental state examination (MMSE) scores. Furthermore, ATT prolongation might be a neuroimaging marker to detect the presence of neuropsychiatric symptoms in dementia (34). However, the difference between ATT in DLB and AD has not been fully investigated. To date, the literature on ATT extension is limited and remains conflicting.
Therefore, this study mainly focuses on the CBF changes in DLB and AD, seeks to investigate the pattern of cerebral perfusion alterations, and explores the correlation between pcASL-derived indices and cognitive scales. The primary goal is to detect whether perfusion indexes could be a new imaging marker that effectively differentiates between the two diseases. We present this article in accordance with the STROBE reporting checklist (available at https://qims.amegroups.com/article/view/10.21037/qims-24-946/rc).
Methods
Participants
The study was conducted in accordance with the Declaration of Helsinki (as revised in 2013). The study was approved by the Medical Ethics Committee of Tianjin First Central Hospital (No. 2018N141KY) and informed consent was provided by all individual participants. A total of 99 participants enrolled in this study, including 25 patients with DLB, 34 patients with AD, and 40 healthy elderly individuals, all of whom were recruited in Tianjin First Central Hospital between 1 September 2022 and 31 January 2024. All patients were screened for dementia, including history, physical, neuropsychological testing, and brain MRI. Neuropsychological scales used included the MMSE and Montreal Cognitive Assessment (MoCA).
AD patients met the criteria for probable AD proposed by the National Institute on Aging and Alzheimer’s Disease Association (NIA-AA) (35,36); DLB diagnosis was established following the clinical criteria proposed by the DLB Consortium’s Fourth Consensus Report for probable DLB (1). The exclusion criteria were as follows: (I) left-handedness; (II) presence of severe cerebrovascular disease; (III) patients with mental disorders or a history of severe head trauma; (IV) recent diagnosis with a serious systemic disease; and (V) poor post-processed image quality.
MRI acquisition
MRI data were acquired using a 3-T MAGNETOM Prisma MR scanner (Siemens Healthcare, Erlangen, Germany) with a 64-channel head-neck coil. Structural images including high-resolution T1-weighted using three-dimensional (3D) magnetization-prepared rapid acquisition gradient echo sequences (3DT1WI-MPRAGE) were acquired with parameters echo time (TE) =2.98 ms, repetition time (TR) =1,550 ms, flip angle (FA) =9°, voxel size =1.0×1.0×1.0 mm3, field of view (FOV) =256×256 mm2, and a T2 fluid-attenuated inversion recovery (dark-fluid) sequence [TR =8,000 ms, TE =85 ms, inversion time (TI) =2,373 ms, FA =150°]. pcASL images were acquired using the following parameters: multiple PLD time =500, 1,000, 1,500, 2,000, and 2,500 ms; TE =36.48 ms; TR =4,100 ms; FA =120°, FOV =240×240 mm2; slice thickness =3.0 mm; voxel size: 2.5×2.5×3.0 mm; and acquisition time =7 min 11 s.
Data processing
The ASL data were processed utilizing the cereflow software (http://www.cereflow.cn) (37), where motion correction was systematically applied to all ASL image sequences. Subtraction of the label images from the control images was performed in pairs, followed by averaging to generate the mean difference image. M0 images were registered to the structural image. Subsequently, the resultant cerebral perfusion parameter maps including mCBF and ATT (38) were also aligned with the structural images.
The origin and head motion corrections were performed before T1-weighted imaging (T1WI) image preprocessing. Then, the anatomical images were segmented into gray matter (GM), white matter (WM), and cerebrospinal fluid (CSF) tissue. Perfusion images were co-registered with those images. Partial volume correction [partial volume effects (PVE) correction, PVEc] was implemented by tissue probability maps in statistical parametric mapping (SPM) toolbox PETPVE12 (39). We used the Müller-Gärtner (40) approach. Ultimately, the arterial supply region brain atlas (41) and Alberta Stroke Program Early CT Score (ASPECTS) (42) were overlaid. The mCBF and ATT values for each region of interest (ROI) were automatically extracted from automated anatomical labeling (AAL) atlas in precision.
Statistical analyses
The statistical software SPSS 26.0 (IBM Corp., Armonk, NY, USA) was performed for the demographic data and cognitive scores of all cases. A P value <0.05 was considered statistically significant. The Kolmogorov-Smirnov was used to test whether the statistics follow a normal distribution. Categorical variables were expressed as counts. Variables that conform to a normal distribution were represented as mean ± standard deviation (SD). Non-normal distribution data were represented as median (interquartile range). A Chi-squared test was used for sex distribution. For continuous measures, differences among the groups were tested with analysis of variance (ANOVA) and Bonferroni tests for multiple comparisons.
Differences in mCBF between groups were analyzed in SPM 12 (https://www.fil.ion.ucl.ac.uk/spm/software/spm12/) package running on Matlab 2022a (MathWorks, Natick, MA, USA), with age, sex, and education years as covariates and general linear model (GLM) was created to identify regional changes. A family-wise error (FWE) correction P<0.05 was considered statistically significant. After that, we used xjview10.0 (https://www.alivelearn.net/xjview/xjview-10-0-released/) toolbox to record cluster voxels by the atlas of AAL3. The perfusion values in the ROIs of the AAL3 atlas were extracted in Matlab 2022a using the DPABIV 7.0 (https://rfmri.org/DPABI).
Moreover, we used ANOVA to assess the ATT differences, with age, gender, and educational year as covariates in the deep perforating branches of the bilateral anterior arteries, the meningeal branches of the bilateral anterior arteries, the bilateral anterior choroidal arteries, the anterior perforating branches of the bilateral middle arteries, the meningeal branches of the bilateral middle arteries, the meningeal branches of the bilateral posterior arteries, and the meningeal branches of the bilateral posterior arteries. Post-hoc comparisons were made using the Bonferroni test.
Spearman correlation analysis was performed on the mCBF indexes and neuropsychological scores, and the correlation coefficient was calculated. Receiver operating characteristic (ROC) curve analysis was used to assess the classified performance of cerebral perfusion indexes.
Results
Demographics and clinical scores
Totals of 25 DLB patients, 34 AD patients, and 40 healthy controls were included in the study; the demographic data for all cases are summarized in Table 1. There were no observed significant differences in age, sex, and years of education among the three groups (P>0.05). The MMSE, MoCA, and Clinical Dementia Rating (CDR) scores were significantly different between the groups (P<0.05). Bonferroni comparison analyses further showed that there was no significant difference between DLB and AD in terms of MMSE and MoCA scores (P>0.05).
Table 1
Variables | DLB (n=25) | AD (n=34) | NC (n=40) | P value |
---|---|---|---|---|
Sex (male/female) | 15/10 | 10/24 | 17/23 | 0.06† |
Age (years) | 71.76±6.34 | 70.74±9.24 | 67.68±7.35 | 0.09‡ |
Education (years) | 10.68±4.85 | 10.41±3.41 | 12.38±3.22 | 0.058‡ |
MMSE scores | 18.0 (12.00, 22.50)1 | 15.50 (10.00, 20.00)2 | 29.00 (28.00, 30.00) | <0.001§** |
MoCA scores | 14.00 (7.50, 18.00)1 | 10.00 (7.75, 16.00)2 | 27.00 (25.25, 28.00) | <0.001§** |
CDR scores | 1.56±0.181 | 1.10±0.102 | 0.00 | <0.001‡,** |
A Chi-squared test was performed for sex comparisons. Age, education, CDR scores are presented as mean ± SD. MMSE, and MoCA scores are presented as median (interquartile range). †, P values are from the Chi-squared test; ‡, P values are from the ANOVA test; §, P values are from Kruskal-Wallis H test. Post-hoc comparison was significant (P<0.05): 1, DLB vs. NC; 2, AD vs. NC. **, P<0.001 was considered a significant difference. DLB, dementia with Lewy bodies; AD, Alzheimer’s disease; NC, normal controls; MMSE, mini-mental state examination; MoCA, Montreal Cognitive Assessment; CDR, Clinical Dementia Rating; ANOVA, analysis of variance; SD, standard deviation.
The contrast of mCBF changes among the three groups
The location of differences in mCBF among the three groups is shown in Figures 1-3, and Table 2 lists detailed information about these parts of the brain. As shown in Figure 1, compared with NC, there was a notable decrease in mCBF in DLB, with the most pronounced reductions occurring in the bilateral frontal lobes, bilateral parietal lobes, bilateral temporal lobes, and the right occipital lobe. In comparison to NC, AD exhibited a notable reduction perfusion, predominantly affecting the bilateral frontal lobes, the right temporal lobe, and the left parietal lobe (Figure 2). Contrasted with AD, DLB exhibited a decreased mCBF primarily in the bilateral frontal and parietal lobes (Figure 3). No statistically significant brain regions were detected where CBF in DLB was higher than it was in AD.
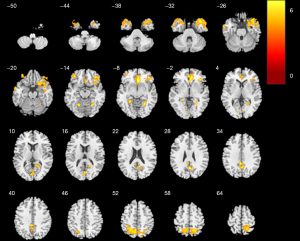
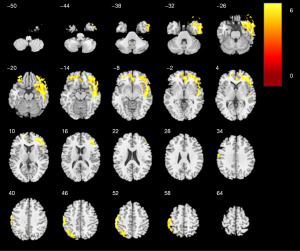
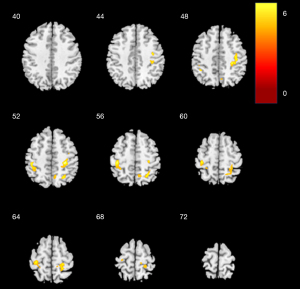
Table 2
Peak region | Hemisphere | Cluster (voxels) | Peak-MNI coordinates | Peak t-statistic | ||
---|---|---|---|---|---|---|
x | y | z | ||||
NC > DLB | ||||||
Fusiform | R | 823 | 25 | −58 | −11 | 6.0693 |
Anterior cingulate cortex, subgenual | R | 944 | 5 | 30 | −7 | 5.7246 |
Precuneus | L | 2,311 | −5 | −55 | 53 | 5.2229 |
Lingual | L | 644 | −23 | −44 | −6 | 5.8298 |
NC > AD | ||||||
Temporal pole: superior temporal gyrus | R | 6,511 | 52 | 12 | −9 | 5.7251 |
Inferior parietal gyrus | L | 3,334 | −55 | −24 | 49 | 5.3134 |
AD > DLB | ||||||
Parietal lobe | R | 1,733 | 29 | −30 | 50 | 6.5214 |
Postcentral | L | 1,298 | −39 | −29 | 56 | 5.3076 |
Superior parietal gyrus | R | 701 | 25 | −60 | 54 | 5.0668 |
Cluster index and size for Figures 1-3. Cluster index was extracted using the xjview tools and are corrected for multiple comparisons. AD, Alzheimer’s disease; DLB, dementia with Lewy bodies; NC, normal controls; MNI, Montreal Neurological Institute; R, right; L, left.
The contrast of ATT changes among the three groups
The differences in ATT values among the three groups in bilateral anterior deep perforator arteries (ACAPerf), bilateral anterior choroidal arteries (AchA), bilateral anterior meningeal arteries (ACAm), bilateral middle meningeal arteries (MCAm), bilateral middle deep perforator arteries (MCAPerf), and bilateral posterior meningeal arteries (PCAm) were statistically significant (P<0.05, Bonferroni comparison was used for multiple comparison, Table 3). The ATT values of the above region in DLB were significantly longer than NC. The left ACAPerf’s ATT of AD was longer than that of NC, but the other regions did not reach the level of statistical significance. Moreover, the ATT of DLB in almost all of the above-mentioned arteries were longer than AD (except the left ACAPerf).
Table 3
Hemisphere | Artery | DLB (s) | AD (s) | NC (s) | F | P value |
---|---|---|---|---|---|---|
L | Anterior deep perforator | 1.19±0.18‡ | 1.10±0.18§ | 1.00±0.18 | 8.153 | 0.001* |
R | Anterior deep perforator | 1.18±0.23†‡ | 1.02±0.22 | 1.01±0.17 | 5.924 | 0.004* |
L | Anterior choroidal | 1.07±0.23†‡ | 0.90±0.18 | 0.89±0.13 | 9.054 | <0.001** |
R | Anterior choroidal | 1.09±0.25†‡ | 0.90±0.16 | 0.85±0.14 | 14.201 | <0.001** |
L | Anterior meningeal | 1.25±0.12†‡ | 1.10±0.14 | 1.08±0.14 | 13.425 | <0.001** |
R | Anterior meningeal | 1.25±0.13†‡ | 1.09±0.15 | 1.08±0.15 | 12.407 | <0.001** |
L | Middle meningeal | 1.26±0.12†‡ | 1.10±0.15 | 1.10±0.12 | 14.17 | <0.001** |
R | Middle meningeal | 1.27±0.15†‡ | 1.11±0.15 | 1.09±0.13 | 14.595 | <0.001** |
L | Middle deep perforator | 1.14±0.20†‡ | 0.96±0.20 | 0.90±0.16 | 13.744 | <0.001** |
R | Middle deep perforator | 1.17±0.20†‡ | 0.98±0.21 | 0.90±0.16 | 16.424 | <0.001** |
L | Posterior meningeal | 1.32±0.15†‡ | 1.21±0.13 | 1.20±0.14 | 6.557 | <0.001** |
R | Posterior meningeal | 1.30±0.16†‡ | 1.19±0.11 | 1.18±0.13 | 7.891 | <0.001* |
Data are presented as mean ± SD. *, P<0.05; **, P<0.001 (†, DLB vs. AD; ‡, DLB vs. NC; §, AD vs. NC). ATT, arterial transit time; DLB, dementia with Lewy bodies; AD, Alzheimer’s disease; NC, normal controls; s, second; R, right; L, left; SD, standard deviation.
Relationship between cerebral perfusion indexes and neuropsychological scales
The relationship between cerebral perfusion indexes and neuropsychological scales is shown in Figure 4. In DLB, MMSE scores were significantly positively correlated with the left superior parietal gyrus’s mCBF (r=0.596, P=0.002) (Figure 4A). Similarly, the DLB’s mCBF in the right precuneus was positively correlated with MMSE scores (r=0.498, P=0.01) (Figure 4B). Likewise, the AD showed that mCBF in the right middle temporal gyrus was significantly positively correlated with MoCA scores (r=0.550, P=0.001) (Figure 4C), and the AD’s mCBF in the left inferior parietal gyrus was significantly positively correlated with MoCA scores (r=0.571, P<0.001) (Figure 4D).
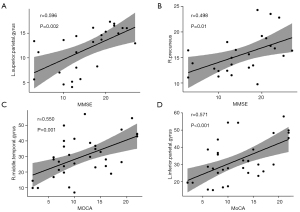
Assessment for diagnosis value of cerebral perfusion indices
As shown in Figure 5, ROC curve analysis showed that the area under the curve (AUC) was 0.709 for the precuneus (mCBF), and 0.861 for the anterior perforating branches of the middle arteries (ATT) in diagnosing DLB and NC. Between DLB and AD, the AUC values of mCBF for precuneus and anterior perforating branches of the middle arteries ATT were 0.759 and 0.724, respectively. The AUC values for mCBF and ATT were 0.639 (parahippocampal) and 0.634 (right middle deep perforator artery), respectively, between AD and NC.
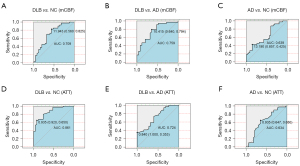
Discussion
In our study, we explored CBF and characteristics of perfusion changes in DLB and AD, and evaluated whether pcASL-derived perfusion indices would help for diagnosis. There were three main outcomes: (I) both DLB and AD have notable reductions in CBF, and prolonged ATT. Hypoperfusion in DLB was observed more extensively; (II) reduced CBF was strongly associated with cognitive performance; (III) CBF and ATT can provide a new view for distinguishing DLB from AD.
The leakage of the BBB and the clearance of CSF play crucial roles in DLB and AD (8-11,43). These processes are closely associated with brain activity and energy demand, which could be detected by ASL and other functional techniques. Our findings indicate that DLB and AD exhibit distinct patterns of CBF alterations. DLB had reduced blood flow in several regions, particularly bilateral frontal lobes, bilateral parietal lobes, bilateral temporal lobes, and the right occipital lobe in contrast to NC. One of the core symptoms of DLB is the presence of visual hallucinations. Previous studies have speculated on a potential relationship between visual hallucinations and the visual cortex (44), but failed to demonstrate any correlation between decreased occipital perfusion and visual hallucinations (25). One study found that DLB with or without hallucinations has no significant difference in occipital blood flow (45). An ASL-based study (27) also did not show occipital hypoperfusion in mild DLB. Is noteworthy that the scores of mild DLB were similar to those of the DLB patients in our study. Whether visual hallucinations in DLB are related to occipital hypoperfusion and can be tracked using the dynamic tracking of the characteristic brain regions with altered blood flow by ASL remains questionable, requiring a large number of cases and great effort to ascertain. We also found that the mCBF in the parietal lobe and temporal lobe of the DLB cases were significantly lower in comparison to NC, which was also found in a previous study (45). As the disease progressed, there was a spread in cerebral hypoperfusion to the frontal cortex and temporal lobe, which was also seen in our study (46). Moreover, studies have also reported that DLB patients have reduced blood flow in the supraorbital gyrus, parietal gyrus, middle temporal gyrus, and insular and inferior frontal gyrus compared to controls (27,47). Equivalently, AD patients had lower mCBF primarily in the bilateral frontal lobes, the right temporal lobe, and the left parietal lobe, which is similar to the findings of previous studies (48,49). Furthermore, it has been shown that the left middle temporal gyrus is an important hub for AD to connect GM atrophy, cerebral perfusion deficits, and cognitive deficits (21). We have also confirmed the presence of DLB had a more diffuse pattern of functional changes than AD, including perfusion changes in the frontal lobe and temporal regions (27,50). In our present study, DLB showed more extensive mCBF changes, with areas of hypoperfusion concentrated in the postcentral gyrus, parietal lobe, and precuneus in contrast to AD (30,51-56).
Our results indicated the presence of prolonged ATT in DLB and AD. PLD defines the interval between blood labeling and image acquisition, and ATT refers to the time required for arterial blood to reach the acquisition level from the labeling level (12,57). Aging may affect the lengthening of ATT and subsequently the CBF (58). The anterior cerebral artery (ACA), the main artery supplying the medial part of the cerebral hemispheres, reduces blood flow to the brain regions supplied by the deep perforating branches of the anterior arteries and anterior choroidal arteries (41), including the medial frontal, medial parietal, and anterior basal ganglia, which may lead to loss of the olfaction, hemiparesis of the muscles of the lower limbs, loss of sensation in the legs and perineum, and incontinence (59-61). DLB patients were more likely to exhibit those symptoms, which may be associated with more severe damage to blood vessels. Moreover, a reduction in CBF to the middle cerebral artery (MCA) results in impaired function of the brain areas supplied by the deep and meningeal branches of the MCA, including the frontal lobe, temporal lobe, parietal lobe, corona radiata, pallidum, and caudate nucleus, which may lead to facial paralysis (62), sensory impairment of the limbs or hemiparesis, speech impairment, and visual impairment (63-65). This could be an explanation for patients with DLB and moderate AD having symptoms of facial stiffness and slow movements. Reduced blood supply of the posterior meningeal arteries can lead to reduced blood flow to the occipital lobe, medial temporal lobe, most of the thalamus, and other regions, which may cause vision loss (66). ATT may be prolonged due to hypoperfusion caused by degeneration of local nerve tissue or the “remote effect” of nerve degeneration in distant brain regions for AD. The study of ATT in AD is controversial and not fully understudied. Yoshiura et al. (32) suggested that region ATT in the hypoperfusion area of AD was not significantly prolonged. However, Mak et al. (33) showed that AD involves a significant hemodynamic disorder, whereby ATT in the left inferior frontal gyrus and middle cingulate gyrus were prolonged. Sun et al. (67) found that the multi-delay combination of CBF and ATT could gain high performance in the identification of AD. Furthermore, the prolongation of ATT in the main artery was significant in DLB, which may be evidence of greater hemodynamic disorder in DLB.
Additionally, we found that some regions of mCBF are positively correlated with neuropsychological scores. We found that the left cingulate cortex, left angular, and supramarginal gyri in DLB have a weak correlation with scores (68). Furthermore, research has shown that reduced CBF is associated with a faster rate of cognitive decline (69). One study (33) found significant hemodynamic deficits in AD, with regional CBF positively correlated with MMSE scores.
We suggest that perfusion indices could offer a new point to distinguish between different conditions (67). It is worth noting that DLB and AD exhibited distinct characteristics of CBF and ATT changes. Our findings suggest that ATT sometimes may be a more accurate indicator than CBF for the differential diagnosis between DLB and AD. It may be associated with reduced blood flow in the medial frontal lobe and basal ganglia of AD, as well as decreased memory and cognition. This undoubtedly facilitates a more comprehensive comprehension of the underlying mechanisms that contribute to cognitive impairment.
Previous studies have predominantly employed rotational labeling of arteries with a single PLD or two PLDs, yet these methodologies are susceptible to erroneous CBF measurements. In this study, pcASL was employed to reduce the total acquisition time and to permit the simultaneous acquisition of ATT and mCBF (70). This sequence also facilitated the selection of appropriate PLDs based on known ATT, thereby ensuring more accurate mCBF measurements (67).
Limitations
Nevertheless, there are several limitations to this study: (I) the sample sizes were small and the distribution of each group was not equitable, which may have caused some bias; (II) the incomplete biomarkers may conceal co-AD-pathology in DLB patients. This study failed to incorporate the various stages of progressive diseases. Moreover, our approach was limited to cross-sectional diagnoses across the patient population, necessitating future longitudinal studies for a more comprehensive understanding. Prolonged clinical follow-up could significantly refine the precision of ASL techniques in identifying CBF modifications linked to neurodegenerative disorders, thereby improving diagnostic accuracy.
Conclusions
DLB and AD display distinct cerebral perfusion changes, and DLB has more extensive brain perfusion alterations. Cerebral perfusion indices can help distinguish DLB from AD. The measurements of CBF and ATT indices by pcASL could advance our understanding of the potential perfusion alteration of DLB and AD patients.
Acknowledgments
Funding: This work was supported by
Footnote
Reporting Checklist: The authors have completed the STROBE reporting checklist. Available at https://qims.amegroups.com/article/view/10.21037/qims-24-946/rc
Conflicts of Interest: All authors have completed the ICMJE uniform disclosure form (available at https://qims.amegroups.com/article/view/10.21037/qims-24-946/coif). The authors have no conflicts of interest to declare.
Ethical Statement: The authors are accountable for all aspects of the work in ensuring that questions related to the accuracy or integrity of any part of the work are appropriately investigated and resolved. The study was conducted in accordance with the Declaration of Helsinki (as revised in 2013). The study was approved by the Medical Ethics Committee of Tianjin First Central Hospital (No. 2018N141KY) and informed consent was provided by all individual participants.
Open Access Statement: This is an Open Access article distributed in accordance with the Creative Commons Attribution-NonCommercial-NoDerivs 4.0 International License (CC BY-NC-ND 4.0), which permits the non-commercial replication and distribution of the article with the strict proviso that no changes or edits are made and the original work is properly cited (including links to both the formal publication through the relevant DOI and the license). See: https://creativecommons.org/licenses/by-nc-nd/4.0/.
References
- McKeith IG, Boeve BF, Dickson DW, Halliday G, Taylor JP, Weintraub D, et al. Diagnosis and management of dementia with Lewy bodies: Fourth consensus report of the DLB Consortium. Neurology 2017;89:88-100. [Crossref] [PubMed]
- Ferman TJ, Smith GE, Kantarci K, Boeve BF, Pankratz VS, Dickson DW, Graff-Radford NR, Wszolek Z, Van Gerpen J, Uitti R, Pedraza O, Murray ME, Aakre J, Parisi J, Knopman DS, Petersen RC. Nonamnestic mild cognitive impairment progresses to dementia with Lewy bodies. Neurology 2013;81:2032-8. [Crossref] [PubMed]
- Orad RI, Shiner T. Differentiating dementia with Lewy bodies from Alzheimer's disease and Parkinson's disease dementia: an update on imaging modalities. J Neurol 2022;269:639-53. [Crossref] [PubMed]
- Kaufer D. Dementia and Lewy bodies. Rev Neurol 2003;37:127-30.
- Colloby SJ, Watson R, Blamire AM, O'Brien JT, Taylor JP. Cortical thinning in dementia with Lewy bodies and Parkinson disease dementia. Aust N Z J Psychiatry 2020;54:633-43. [Crossref] [PubMed]
- Irwin DJ, Grossman M, Weintraub D, Hurtig HI, Duda JE, Xie SX, et al. Neuropathological and genetic correlates of survival and dementia onset in synucleinopathies: a retrospective analysis. Lancet Neurol 2017;16:55-65. [Crossref] [PubMed]
- Raz L, Knoefel J, Bhaskar K. The neuropathology and cerebrovascular mechanisms of dementia. J Cereb Blood Flow Metab 2016;36:172-86. [Crossref] [PubMed]
- Uchida Y, Kan H, Sakurai K, Horimoto Y, Hayashi E, Iida A, Okamura N, Oishi K, Matsukawa N. APOE ɛ4 dose associates with increased brain iron and β-amyloid via blood-brain barrier dysfunction. J Neurol Neurosurg Psychiatry 2022; Epub ahead of print. [Crossref]
- Uchida Y, Kan H, Sakurai K, Oishi K, Matsukawa N. Contributions of blood-brain barrier imaging to neurovascular unit pathophysiology of Alzheimer's disease and related dementias. Front Aging Neurosci 2023;15:1111448. [Crossref] [PubMed]
- Uchida Y, Kan H, Furukawa G, Onda K, Sakurai K, Takada K, Matsukawa N, Oishi K. Relationship between brain iron dynamics and blood-brain barrier function during childhood: a quantitative magnetic resonance imaging study. Fluids Barriers CNS 2023;20:60. [Crossref] [PubMed]
- Ryman SG, Vakhtin AA, Mayer AR, van der Horn HJ, Shaff NA, Nitschke SR, Julio KR, Tarawneh RM, Rosenberg GA, Diaz SV, Pirio Richardson SE, Lin HC. Abnormal Cerebrovascular Activity, Perfusion, and Glymphatic Clearance in Lewy Body Diseases. Mov Disord 2024;39:1258-68. [Crossref] [PubMed]
- Alsop DC, Detre JA, Golay X, Günther M, Hendrikse J, Hernandez-Garcia L, Lu H, MacIntosh BJ, Parkes LM, Smits M, van Osch MJ, Wang DJ, Wong EC, Zaharchuk G. Recommended implementation of arterial spin-labeled perfusion MRI for clinical applications: A consensus of the ISMRM perfusion study group and the European consortium for ASL in dementia. Magn Reson Med 2015;73:102-16. [Crossref] [PubMed]
- Buxton RB, Frank LR, Wong EC, Siewert B, Warach S, Edelman RR. A general kinetic model for quantitative perfusion imaging with arterial spin labeling. Magn Reson Med 1998;40:383-96. [Crossref] [PubMed]
- van Laar PJ, van der Grond J, Hendrikse J. Brain perfusion territory imaging: methods and clinical applications of selective arterial spin-labeling MR imaging. Radiology 2008;246:354-64. [Crossref] [PubMed]
- Wang DJ, Alger JR, Qiao JX, Gunther M, Pope WB, Saver JL, Salamon N, Liebeskind DS. Multi-delay multi-parametric arterial spin-labeled perfusion MRI in acute ischemic stroke - Comparison with dynamic susceptibility contrast enhanced perfusion imaging. Neuroimage Clin 2013;3:1-7. [Crossref] [PubMed]
- Chen Y, Wolk DA, Reddin JS, Korczykowski M, Martinez PM, Musiek ES, Newberg AB, Julin P, Arnold SE, Greenberg JH, Detre JA. Voxel-level comparison of arterial spin-labeled perfusion MRI and FDG-PET in Alzheimer disease. Neurology 2011;77:1977-85. [Crossref] [PubMed]
- Takahashi H, Ishii K, Hosokawa C, Hyodo T, Kashiwagi N, Matsuki M, Ashikaga R, Murakami T. Clinical application of 3D arterial spin-labeled brain perfusion imaging for Alzheimer disease: comparison with brain perfusion SPECT. AJNR Am J Neuroradiol 2014;35:906-11. [Crossref] [PubMed]
- Musiek ES, Chen Y, Korczykowski M, Saboury B, Martinez PM, Reddin JS, Alavi A, Kimberg DY, Wolk DA, Julin P, Newberg AB, Arnold SE, Detre JA. Direct comparison of fluorodeoxyglucose positron emission tomography and arterial spin labeling magnetic resonance imaging in Alzheimer's disease. Alzheimers Dement 2012;8:51-9. [Crossref] [PubMed]
- Urso D, Gnoni V, De Blasi R, Anastasia A, Aarsland D, Chaudhuri Ray K, Logroscino G. Neuroimaging Biomarkers in a Patient With Probable Psychiatric-Onset Prodromal Dementia With Lewy Bodies. Neurology 2022;99:654-7. [Crossref] [PubMed]
- Nedelska Z, Senjem ML, Przybelski SA, Lesnick TG, Lowe VJ, Boeve BF, Arani A, Vemuri P, Graff-Radford J, Ferman TJ, Jones DT, Savica R, Knopman DS, Petersen RC, Jack CR, Kantarci K. Regional cortical perfusion on arterial spin labeling MRI in dementia with Lewy bodies: Associations with clinical severity, glucose metabolism and tau PET. Neuroimage Clin 2018;19:939-47. [Crossref] [PubMed]
- Dong H, Guo L, Yang H, Zhu W, Liu F, Xie Y, Zhang Y, Xue K, Li Q, Liang M, Zhang N, Qin W. Association between gray matter atrophy, cerebral hypoperfusion, and cognitive impairment in Alzheimer's disease. Front Aging Neurosci 2023;15:1129051. [Crossref] [PubMed]
- Huang CW, Hsu SW, Chang YT, Huang SH, Huang YC, Lee CC, Chang WN, Lui CC, Chen NC, Chang CC. Cerebral Perfusion Insufficiency and Relationships with Cognitive Deficits in Alzheimer's Disease: A Multiparametric Neuroimaging Study. Sci Rep 2018;8:1541. [Crossref] [PubMed]
- Li Q, Lv X, Jin F, Liao K, Gao L, Xu J. Associations of Polygenic Risk Score for Late-Onset Alzheimer's Disease With Biomarkers. Front Aging Neurosci 2022;14:849443. [Crossref] [PubMed]
- Pasquier J, Michel BF, Brenot-Rossi I, Hassan-Sebbag N, Sauvan R, Gastaut JL. Value of (99m)Tc-ECD SPET for the diagnosis of dementia with Lewy bodies. Eur J Nucl Med Mol Imaging 2002;29:1342-8. [Crossref] [PubMed]
- Tateno M, Utsumi K, Kobayashi S, Takahashi A, Saitoh M, Morii H, Fujii K, Teraoka M. Usefulness of a blood flow analyzing program 3DSRT to detect occipital hypoperfusion in dementia with Lewy bodies. Prog Neuropsychopharmacol Biol Psychiatry 2008;32:1206-9. [Crossref] [PubMed]
- Shimizu S, Hanyu H, Kanetaka H, Iwamoto T, Koizumi K, Abe K. Differentiation of dementia with Lewy bodies from Alzheimer's disease using brain SPECT. Dement Geriatr Cogn Disord 2005;20:25-30. [Crossref] [PubMed]
- Roquet D, Sourty M, Botzung A, Armspach JP, Blanc F. Brain perfusion in dementia with Lewy bodies and Alzheimer's disease: an arterial spin labeling MRI study on prodromal and mild dementia stages. Alzheimers Res Ther 2016;8:29. [Crossref] [PubMed]
- Goto H, Ishii K, Uemura T, Miyamoto N, Yoshikawa T, Shimada K, Ohkawa S. Differential diagnosis of dementia with Lewy Bodies and Alzheimer Disease using combined MR imaging and brain perfusion single-photon emission tomography. AJNR Am J Neuroradiol 2010;31:720-5. [Crossref] [PubMed]
- Hanyu H, Shimizu S, Hirao K, Kanetaka H, Sakurai H, Iwamoto T, Koizumi K, Abe K. Differentiation of dementia with Lewy bodies from Alzheimer's disease using Mini-Mental State Examination and brain perfusion SPECT. J Neurol Sci 2006;250:97-102. [Crossref] [PubMed]
- Colloby SJ, Fenwick JD, Williams ED, Paling SM, Lobotesis K, Ballard C, McKeith I, O'Brien JT. A comparison of (99m)Tc-HMPAO SPET changes in dementia with Lewy bodies and Alzheimer's disease using statistical parametric mapping. Eur J Nucl Med Mol Imaging 2002;29:615-22. [Crossref] [PubMed]
- Binnewijzend MA, Kuijer JP, van der Flier WM, Benedictus MR, Möller CM, Pijnenburg YA, Lemstra AW, Prins ND, Wattjes MP, van Berckel BN, Scheltens P, Barkhof F. Distinct perfusion patterns in Alzheimer's disease, frontotemporal dementia and dementia with Lewy bodies. Eur Radiol 2014;24:2326-33. [Crossref] [PubMed]
- Yoshiura T, Hiwatashi A, Yamashita K, Ohyagi Y, Monji A, Takayama Y, Nagao E, Kamano H, Noguchi T, Honda H. Simultaneous measurement of arterial transit time, arterial blood volume, and cerebral blood flow using arterial spin-labeling in patients with Alzheimer disease. AJNR Am J Neuroradiol 2009;30:1388-93. [Crossref] [PubMed]
- Mak HK, Chan Q, Zhang Z, Petersen ET, Qiu D, Zhang L, Yau KK, Chu LW, Golay X. Quantitative assessment of cerebral hemodynamic parameters by QUASAR arterial spin labeling in Alzheimer's disease and cognitively normal Elderly adults at 3-tesla. J Alzheimers Dis 2012;31:33-44. [Crossref] [PubMed]
- Jiang J, Wang A, Liu Y, Yao Z, Sun M, Jiang T, Li W, Jiang S, Zhang X, Wang Y, Zhang Y, Jia Z, Zou X, Xu J. Spatiotemporal Characteristics of Regional Brain Perfusion Associated with Neuropsychiatric Symptoms in Patients with Alzheimer's Disease. J Alzheimers Dis 2023;95:981-93. [Crossref] [PubMed]
- McKhann GM, Knopman DS, Chertkow H, Hyman BT, Jack CR Jr, Kawas CH, Klunk WE, Koroshetz WJ, Manly JJ, Mayeux R, Mohs RC, Morris JC, Rossor MN, Scheltens P, Carrillo MC, Thies B, Weintraub S, Phelps CH. The diagnosis of dementia due to Alzheimer's disease: recommendations from the National Institute on Aging-Alzheimer's Association workgroups on diagnostic guidelines for Alzheimer's disease. Alzheimers Dement 2011;7:263-9. [Crossref] [PubMed]
- Dubois B. The Emergence of a New Conceptual Framework for Alzheimer's Disease. J Alzheimers Dis 2018;62:1059-66. [Crossref] [PubMed]
- van Osch MJ, Teeuwisse WM, Chen Z, Suzuki Y, Helle M, Schmid S. Advances in arterial spin labelling MRI methods for measuring perfusion and collateral flow. J Cereb Blood Flow Metab 2018;38:1461-80. [Crossref] [PubMed]
- Hernandez-Garcia L, Lahiri A, Schollenberger J. Recent progress in ASL. Neuroimage 2019;187:3-16. [Crossref] [PubMed]
- Gonzalez-Escamilla G, Lange C, Teipel S, Buchert R, Grothe MJ. PETPVE12: an SPM toolbox for Partial Volume Effects correction in brain PET - Application to amyloid imaging with AV45-PET. Neuroimage 2017;147:669-77. [Crossref] [PubMed]
- Müller-Gärtner HW, Links JM, Prince JL, Bryan RN, McVeigh E, Leal JP, Davatzikos C, Frost JJ. Measurement of radiotracer concentration in brain gray matter using positron emission tomography: MRI-based correction for partial volume effects. J Cereb Blood Flow Metab 1992;12:571-83. [Crossref] [PubMed]
- Tatu L, Moulin T, Bogousslavsky J, Duvernoy H. Arterial territories of the human brain: cerebral hemispheres. Neurology 1998;50:1699-708. [Crossref] [PubMed]
- Wang DJ, Alger JR, Qiao JX, Gunther M, Pope WB, Saver JL, Salamon N, Liebeskind DSUCLA Stroke Investigators. Multi-delay multi-parametric arterial spin-labeled perfusion MRI in acute ischemic stroke - Comparison with dynamic susceptibility contrast enhanced perfusion imaging. Neuroimage Clin 2013;3:1-7. [Crossref] [PubMed]
- Yang A, Zhuang H, Du L, Liu B, Lv K, Luan J, Hu P, Chen F, Wu K, Shu N, Shmuel A, Ma G, Wang Y. Evaluation of whole-brain oxygen metabolism in Alzheimer's disease using QSM and quantitative BOLD. Neuroimage 2023;282:120381. [Crossref] [PubMed]
- Howard R, David A, Woodruff P, Mellers I, Wright J, Brammer M, Bullmore E, Williams S. Seeing visual hallucinations with functional magnetic resonance imaging. Dement Geriatr Cogn Disord 1997;8:73-7. [Crossref] [PubMed]
- Lobotesis K, Fenwick JD, Phipps A, Ryman A, Swann A, Ballard C, McKeith IG, O'Brien JT. Occipital hypoperfusion on SPECT in dementia with Lewy bodies but not AD. Neurology 2001;56:643-9. [Crossref] [PubMed]
- Park KW, Hong YJ, Park JH, Park H, Cheon SM, Kim JW, Kim BC, Jeong YJ, Yoon HJ, Kang DY. Pattern of cerebral hypoperfusion according to the clinical staging in dementia with Lewy bodies. Neurocase 2018;24:83-9. [Crossref] [PubMed]
- Yoshida T, Mori T, Yamazaki K, Sonobe N, Shimizu H, Matsumoto T, Kikuchi K, Miyagawa M, Mochizuki T, Ueno S. Relationship between regional cerebral blood flow and neuropsychiatric symptoms in dementia with Lewy bodies. Int J Geriatr Psychiatry 2015;30:1068-75. [Crossref] [PubMed]
- Zhou TD, Zhang Z, Balachandrasekaran A, Raji CA, Becker JT, Kuller LH, Ge Y, Lopez OL, Dai W, Gach HM. Prospective Longitudinal Perfusion in Probable Alzheimer's Disease Correlated with Atrophy in Temporal Lobe. Aging Dis 2024;15:1855-71. [Crossref] [PubMed]
- Hauser T, Schönknecht P, Thomann PA, Gerigk L, Schröder J, Henze R, Radbruch A, Essig M. Regional cerebral perfusion alterations in patients with mild cognitive impairment and Alzheimer disease using dynamic susceptibility contrast MRI. Acad Radiol 2013;20:705-11. [Crossref] [PubMed]
- Binnewijzend MA, Benedictus MR, Kuijer JP, van der Flier WM, Teunissen CE, Prins ND, Wattjes MP, van Berckel BN, Scheltens P, Barkhof F. Cerebral perfusion in the predementia stages of Alzheimer's disease. Eur Radiol 2016;26:506-14. [Crossref] [PubMed]
- Londos E, Passant U, Brun A, Rosén I, Risberg J, Gustafson L. Regional cerebral blood flow and EEG in clinically diagnosed dementia with Lewy bodies and Alzheimer's disease. Arch Gerontol Geriatr 2003;36:231-45. [Crossref] [PubMed]
- Nakata T, Shimada K, Iba A, Oda H, Terashima A, Koide Y, Kawasaki R, Yamada T, Ishii K. Differential diagnosis of MCI with Lewy bodies and MCI due to Alzheimer's disease by visual assessment of occipital hypoperfusion on SPECT images. Jpn J Radiol 2024;42:308-18. [Crossref] [PubMed]
- O'Brien JT, Firbank MJ, Davison C, Barnett N, Bamford C, Donaldson C, Olsen K, Herholz K, Williams D, Lloyd J. 18F-FDG PET and perfusion SPECT in the diagnosis of Alzheimer and Lewy body dementias. J Nucl Med 2014;55:1959-65. [Crossref] [PubMed]
- Chiba Y, Iseki E, Fujishiro H, Ota K, Kasanuki K, Suzuki M, Hirayasu Y, Arai H, Sato K. Early differential diagnosis between Alzheimer's disease and dementia with Lewy bodies: Comparison between (18)F-FDG PET and (123)I-IMP SPECT. Psychiatry Res Neuroimaging 2016;249:105-12. [Crossref] [PubMed]
- Ye BS, Lee S, Yoo H, Chung SJ, Lee YH, Choi Y, Lee PH, Sohn YH, Yun M. Distinguishing between dementia with Lewy bodies and Alzheimer's disease using metabolic patterns. Neurobiol Aging 2020;87:11-7. [Crossref] [PubMed]
- Fong TG, Inouye SK, Dai W, Press DZ, Alsop DC. Association cortex hypoperfusion in mild dementia with Lewy bodies: a potential indicator of cholinergic dysfunction? Brain Imaging Behav 2011;5:25-35. [Crossref] [PubMed]
- Suzuki Y, Clement P, Dai W, Dolui S, Fernández-Seara MA, Lindner T, Mutsaerts HJMM, Petr J, Shao X, Taso M, Thomas DL. ASL lexicon and reporting recommendations: A consensus report from the ISMRM Open Science Initiative for Perfusion Imaging (OSIPI). Magn Reson Med 2024;91:1743-60. [Crossref] [PubMed]
- Liu Y, Zhu X, Feinberg D, Guenther M, Gregori J, Weiner MW, Schuff N. Arterial spin labeling MRI study of age and gender effects on brain perfusion hemodynamics. Magn Reson Med 2012;68:912-22. [Crossref] [PubMed]
- Beason-Held LL, Kerley CI, Chaganti S, Moghekar A, Thambisetty M, Ferrucci L, Resnick SM, Landman BA. Health Conditions Associated with Alzheimer's Disease and Vascular Dementia. Ann Neurol 2023;93:805-18. [Crossref] [PubMed]
- Liu X, Halvorsen S, Blanke N, Downs M, Stein TD, Bigio IJ, Zaia J, Zhang Y. Progressive mechanical and structural changes in anterior cerebral arteries with Alzheimer's disease. Alzheimers Res Ther 2023;15:185. [Crossref] [PubMed]
- Soysal P, Koc Okudur S, Uslu F, Smith L. Functional loss and worsening geriatric assessment parameters are more common in dementia with Lewy bodies than Alzheimer's disease. Psychogeriatrics 2023;23:77-85. [Crossref] [PubMed]
- Morecraft RJ, Stilwell-Morecraft KS, Rossing WR. The motor cortex and facial expression: new insights from neuroscience. Neurologist 2004;10:235-49. [Crossref] [PubMed]
- Ng YS, Stein J, Salles SS, Black-Schaffer RM. Clinical characteristics and rehabilitation outcomes of patients with posterior cerebral artery stroke. Arch Phys Med Rehabil 2005;86:2138-43. [Crossref] [PubMed]
- Kyeong S, Kang H, Kim DH. Structural Integrity and Functional Neural Activity Associated with Oral Language Function after Stroke. J Clin Med 2022;11:3028. [Crossref] [PubMed]
- Alkolfat F, Abdel Galeel A, Bassiouny AR, Eldeeb H, Radwan A, Ashram YA. Patterns of Visual Task-based Functional MRI Activation in Chronic Posterior Cerebral Artery Stroke Patients. Clin Neuroradiol 2023;33:769-81. [Crossref] [PubMed]
- Vishteh AG, Smith KA, McDougall CG, Spetzler RF. Distal posterior cerebral artery revascularization in multimodality management of complex peripheral posterior cerebral artery aneurysms: technical case report. Neurosurgery 1998;43:166-70. [Crossref] [PubMed]
- Sun M, Wang YL, Li R, Jiang J, Zhang Y, Li W, Zhang Y, Jia Z, Chappell M, Xu J. Potential Diagnostic Applications of Multi-Delay Arterial Spin Labeling in Early Alzheimer's Disease: The Chinese Imaging, Biomarkers, and Lifestyle Study. Front Neurosci 2022;16:934471. [Crossref] [PubMed]
- Nakata T, Shimada K, Iba A, Oda H, Terashima A, Koide Y, Kawasaki R, Yamada T, Ishii K. Correlation between noise pareidolia test scores for visual hallucinations and regional cerebral blood flow in dementia with Lewy bodies. Ann Nucl Med 2022;36:384-92. [Crossref] [PubMed]
- Benedictus MR, Leeuwis AE, Binnewijzend MA, Kuijer JP, Scheltens P, Barkhof F, van der Flier WM, Prins ND. Lower cerebral blood flow is associated with faster cognitive decline in Alzheimer's disease. Eur Radiol 2017;27:1169-75. [Crossref] [PubMed]
- Wang X, Bishop C, O'Callaghan J, Gayhoor A, Albani J, Theriault W, Chappell M, Golay X, Wang D, Becerra L. MRI assessment of cerebral perfusion in clinical trials. Drug Discov Today 2023;28:103506. [Crossref] [PubMed]