The role of cardiac adipose tissue in coronary artery disease: a narrative review of cardiac imaging studies
Introduction
Coronary artery disease (CAD) is the leading cause of death across the world. It is the cause of roughly 2 out of 10 deaths in people less than 65 years old in the United States, according to the National Health Center for Statistics (1). Currently, computed tomography angiography (CTA) is used to anatomically image coronary artery stenosis in patients with stable chest pain in the clinical setting (2).
Beyond anatomical lesion assessment and other clinical factors to evaluate patients’ risk for CAD, new research in cardiac adipose tissue (CAT) has shown promise as new biomarkers of CAD. CAT is linked with many stages of development of CAD, all of which can be tracked and linked to disease outcomes of CAD (3-6). The recent enthusiasm in researching CAT’s link to CAD endpoints has yielded interesting results; shifting the focus from anatomic imaging to functional imaging of atherosclerosis. Despite the impressive results of these studies, there still is lack of studies exploring these imaging methods for assessing CAT and their value for application in the assessment of coronary heart disease and its associated risk events. The purpose of this review is to understand how CAT affect the pathogenesis of CAD, discuss recent imaging solutions available for analyzing CAT, and provide insights for future researchers to potentially integrate CAT metrics in CAD risk assessment. We present this article in accordance with the Narrative Review reporting checklist (available at https://qims.amegroups.com/article/view/10.21037/qims-24-1245/rc).
Methods
This review paper was conducted with the approval of the Medical Ethics Committee of the Second Affiliated Hospital of Zhejiang University School of Medicine [Institutional Review Board (IRB) approval number (2024) (0566)]. A comprehensive literature search in PubMed using the keywords: “epicardial adipose tissue”, “paracardial adipose tissue”, “pericoronary adipose tissue”, “major adverse coronary events”, “coronary plaques”, "high-risk plaque", “risk factor” and “coronary artery disease” was conducted. The search was carried out during January 2004 to May 2024 timeframe, and limited to articles published in English. Review, systematic reviews, meta-analyses, and human clinical trials were included in the literature review. Editorials, comments, case reports, redundant articles and studies carried out with imaging techniques other than computed tomography, cardiac magnetic resonance (CMR) and echocardiography and, articles not discussing the relationship between CAT and CAD were excluded (Table 1, Figure S1). There are 95 studies retrieved. After a comprehensive reading of the research along with their relevant references, a total of 63 articles were included in this paper.
Table 1
Items | Specification |
---|---|
Date of search | May 31, 2024 |
Database searched | PubMed |
Search terms used | A combination of “epicardial adipose tissue”, “paracardial adipose tissue”, “pericoronary adipose tissue”, “coronary plaque”, “high-risk plaque”, “major adverse coronary events”, “risk factor”, and “coronary artery disease” |
Timeframe | January 1 2004 to May 31, 2024 |
Inclusion criteria and exclusion criteria | Inclusion criteria: reviews, systematic reviews, meta-analysis, clinical trials conducted on human subjects published in English |
Exclusion criteria: editorials, comments, case reports, redundant articles, articles that did not discuss the relationship between CAT and CAD, and studies carried out with imaging techniques other than computed tomography, cardiac magnetic resonance or echocardiography | |
Selection process | R.B. and Xiaojun Xu carried out selection |
CAT, cardiac adipose tissue; CAD, coronary artery disease.
CAT
CAT is composed of different fat depot types: (I) epicardial adipose tissue (EAT), (II) pericoronary adipose tissue (PCAT), and (III) paracardial adipose tissue (PAT) (Figure 1). These tissues have different locations, distributions, and functions.
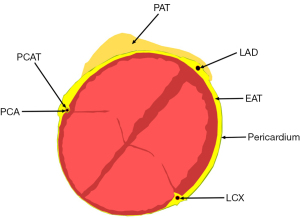
EAT
EAT is defined as the fat collection between visceral pericardium and the myocardium without any interfering structure from the latter and the epicardial vessels. It is part of visceral fat, which was evolved from the splanchnopleuric mesoderm (7). It is non-uniformly distributed over the heart and its properties vary from region to region (8).
EAT though being a white adipose tissue, also possesses brown fat-like capabilities which has thermogenic properties; protecting the heart against hypothermia (9). High levels of free-fatty acid (FFA) in the blood hinders the myocardium to properly generate and propagate contraction cycles. EAT stores surplus FFA from the circulation protecting the heart (10). Excessive FFA within EAT leads to a decrease in the metabolism of FFA. This leads to higher circulating levels of FFA that cannot be cleared, accumulating in non-adipose tissues in a process defined as lipotoxicity (11). EAT also functions as an endocrine organ interacting with the myocardium through vasocrine and paracrine pathways. Adiponectin secretion maintains the coronary circulation by decreasing oxidative stress and reducing inflammatory cytokines such as interleukin-6 and c-reactive protein (12).
Dysfunctioning EAT contributes to the development of CAD through many pathways. It also triggers endothelial disruption by releasing stress mediators, which induces the expression of adhesion molecules like VCAM-1. This process leads to the migration of monocytes to coronary artery endothelial cells (13). EAT tissue samples extracted from patients with CAD demonstrated a higher density of inflammatory M1 macrophages than M2 anti-inflammatory macrophages. This imbalance leads to atheroma instability and their subsequent rupture (14). Furthermore, leptin and resistin, hormones secreted by EAT cause an increase in vascular oxidative stress which escalates cardiovascular risk (15).
PCAT
PCAT is a special EAT around the cardiovascular system. However, there is no clear anatomical or structural boundary between PCAT and EAT (16). PCAT shares blood supply with the blood vessels of the coronary artery and regulates myocardial metabolism. It maintains vascular homeostasis by local secretion of anti-inflammatory and antioxidant chemokines (17). The exchange of information between the artery wall and PCAT happens in a bidirectional fashion. This communication occurs via local paracrine signaling and endocrine pathways involving circulating fatty cytokines, resistin, chemerin, and leptin (18,19). These abnormalities promote the occurrence of atherosclerotic plaques.
Furthermore, the structure of PCAT is also affected by inflammation of adjacent coronary vessels. Inflammation inhibits the differentiation of fat cells; and prevents the accumulation of lipids in cells, causing the size of fat cells and their contents to change from the lipid phase to the aqueous phase (16).
PAT
PAT is located anterior to EAT and on the outside layer of the pericardium. It is derived from the thoracic mesoderm. Pericardium acts as a barrier between the EAT and PAT. PAT is more closely related to abdominal visceral adipose tissue and is also metabolically active. It is supplied by non-coronary arteries. PAT may contribute to the formation of atherosclerosis through endocrine mechanisms, although this role is not fully understood. A study by El Khoudary et al. suggested that the quantity of PAT is regulated by hormone levels of estradiol E2 as the tissue expresses the estrogen receptor Er-ɑ, which is found in the subcutaneous and visceral adipose tissue of the human body (20).
Imaging of CAT
CAT can be studied and quantified by different imaging techniques like transthoracic echocardiography, cardiac computed tomography (CCT), and CMR imaging.
Echocardiography
Transthoracic echocardiography is readily available, low cost, rapid, and offers no radiation exposure to the patient. EAT is illustrated by echocardiography as the echo-lucent area between the epicardium of the right ventricle and the parietal pericardium, which is seen as a thick line above the right ventricular free wall on echo (21). PAT is the hypoechoic area in front of EAT and the parietal pericardium. It can be easily differentiated from EAT since it does not change with the cardiac cycle.
Cardiac echo has many limitations (22). It cannot assess EAT that is situated at the back of the heart and also cannot visualize PCAT. It is usually inadequate for the assessment of obese patients and the repeatability of the measurements is relatively poor since it is operator dependent.
Magnetic resonance imaging
Fast spin echo T1-weighted black blood sequence allows excellent visualization of the visceral and parietal pericardium, permitting easy evaluation of EAT and PAT. However, it is not performed routinely. Steady-state free-precession imaging technique provides a precise and reproducible definition of EAT (23). EAT is hyperintense, the light grey region in between the hypointense myocardium and visceral pericardium (7).
Though CMR imaging is relatively safe for patients since it does not use any radiation; it is contraindicated in people with morbid obesity, MR-unsafe implants, or people suffering from claustrophobia. It is limited by motion artifacts, which may blur images that need to be analyzed. Furthermore, similar to cardiac echocardiography, it cannot assess PCAT.
Computed tomography
CCT is the best imaging technique for evaluating cardiac fat tissue as it has a high spatial resolution and offers good visibility of the pericardium. It can easily evaluate volume and density metrics of all components of CAT. In the literature, EAT and PCAT measurement is based on non-contrast CCT scans with the following most common scan parameters: ECG gating, tube voltage of 120 kVp, and a slice thickness of 3 mm.
EAT volumetric quantification is done by identifying the upper and inferior limits of the heart and slice tracing of the inner thoracic cavity and pericardium through the use of semi-automatic software (24). Fully automated methods using machine learning techniques also exist (25). EAT and PCAT are generally visualized with attenuation ranging from −190 to −30 Hounsfield units (HU) surrounding the myocardium (Figure 2).
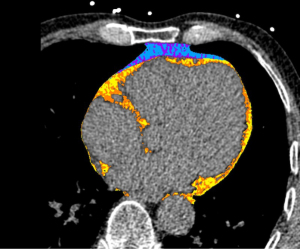
PCAT metrics are obtained using coronary CTA. Measurements around the right coronary artery (RCA) are preferred since it has fewer side branches, an abundance of fat depot, and a uniform diameter that spans from its ostial to distal segments. At present, PCATMA (mean attenuation of PCAT) is the most common metric used in PCAT measurement (26). It is defined as the mean attenuation of adipose tissue of 3 mm thickness around the RCA with a length of 40 mm and the measurement starting 10 mm from the ostium and with a 1 mm gap surrounding the blood vessel wall to allow for a standardized measurement and correct for blooming artifacts of contrast medium (Figure 3) (16).
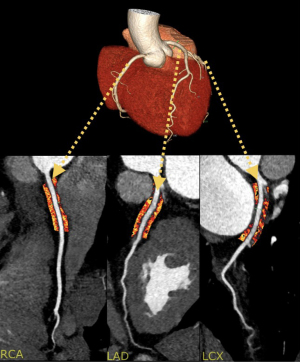
PCATMA’s value is dependent upon adipocyte size, and lipid content of the tissue. Inflammation in the coronary arteries prevents adipocyte maturation and lipid accumulation within the adipocytes leading to more aqueous and smaller adipocytes. Histological specimens confirm that the higher the PCAT attenuation (PCATa) value, the smaller and more aqueous the adipocytes representing inflamed coronary arteries (27).
Fat attenuation index (FAI), an advanced metric of PCATa, is appropriately corrected and weighted for different criteria such as obesity, anatomy, and technical scanning parameters. It has several advantages as a measure of vascular inflammation. It is not affected by the degree of coronary calcification, nor is it affected by systemic inflammation, and lastly, it does not correlate with the intensity of coronary stenosis (16).
CAT and CAD
CAT as a predictor of CAD
Smoking, high blood pressure, diabetes, and obesity are all known and well-researched clinical risk factors for CAD. Emerging reports have shown the role of CAT albeit complex as a predictor for CAD.
In 2008, Eroglu et al. found that EAT thickness measured by echocardiography correlated with the occurrence of CAD (28). EAT was also found to be noticeably higher in patients with metabolic syndrome (MS) as well as patients with both CAD and MS. One possible mechanism postulated for EAT relationship with CAD is that EAT being a component of visceral adipose tissue is correlated with MS, which itself forms part of a traditional risk factor for CAD (29).
Further, a meta-analysis showed that location-specific EAT was related with obstructive CAD (30). EAT thickness at the left atrioventricular groove followed by the right free ventricular groove had the greatest association with obstructive CAD even though results suffered in homogeneity. This may be due to the low reproducibility and variability of cardiac echography EAT measurements as compared to EAT thickness metrics obtained from CT scans (31). Nonetheless, this showed a pattern emerging among which EAT near coronary artery segments were most likely to be correlated with obstructive CAD.
In line with the above meta-analysis (30), PCAT which forms part of EAT and is closer to coronary arteries was found to be correlated with stenotic lesions in patients with CAD (32). Mahabadi et al. (33) found that PCAT was associated with atherosclerosis development after multivariate adjustment, which included traditional cardiovascular risk factors. An imbalance between pro- and anti-inflammatory mediators and cytokines may be a probable mechanism for the role of PCAT in CAD. A high level of inflammatory activity was revealed by 18F-fluorodeoxyglucose positron emission tomography-computed tomography (18FDG PET-CT) in patients with stable CAD (34).
Though not directly associated with the endpoints of CAD, there is evidence that postmenopausal women with higher PAT demonstrated more coronary calcification than premenopausal women (35). The same authors found that middle-aged women with higher PAT had lower levels of estradiol E2 (20). Additionally, analysis of the KEEPS trial showed that women on oral conjugated equine estrogens had less atherosclerosis progression compared to others on different hormonal treatments for each standard deviation increase in PAT (36). Thus, PAT may be a potential specific CAD risk indicator for menopausal women that needs to be further researched.
In conclusion, the evidence so far shows that CAT and the risk of CAD are associated. Furthermore, the adipose tissue close to the coronary artery, known as PCAT, is the most associated with the pathomechanisms of CAD, due to its close correlation with local inflammation, and may play the greatest role in risk prediction of poor prognosis of CAD, which shows potential to improve risk management in patients with CAD.
CAT and atherosclerotic burden
Multiple detector spiral CT (MDCT) has become an essential tool in exploring the link between CAT and atherosclerotic burden, as it provides simultaneous assessment of plaque and CAT. Coronary calcium scoring (CAC) is a reliable predictor of coronary events and is used as a surrogate for atherosclerosis burden in research since it correlates positively with plaque burden (37,38). EAT volume (EATv) is positively correlated to CAC (39,40). With each additional 10 mL of EATv, CAC score increased by 3.7% after adjustment for known confounders (40). EATv relationship with CAC score was found to be independent of visceral fat, age, and sex. This suggests that a different mechanism other than visceral fat is responsible for the association between EATv and CAC score. Subsequent longitudinal studies found that an increase in EATv was associated with a progression in CAC scores (41,42). However, the link disappeared after correction for traditional cardiovascular (CV) risk factors.
In former studies, EATv was the greatest amongst subjects with mixed plaque (MP) and non-calcified plaque (NCP) (39,40). Patients with that kind of plaque were mostly younger and had lower atherosclerotic burden than patients with calcified plaques (CP), indicating that EAT might be connected to the initial development of plaque (39,43). This conviction that EAT might be associated with early onset of NCP is supported by a prospective 5-year study of 122 subjects which showed de-novo appearance of NCP in patients with a greater amount of EAT at baseline (44). Increasing evidence indicates that EAT promotes the early stages of atherosclerosis through the secretion of inflammatory mediators and cytokines, causing increased oxidative stress and the accumulation of lipids in the coronary lumen (45).
PCAT is also linked with plaque burden. A recent study published in 2024 found PCATa to be associated with a high level of NCP burden (46). The high NCP group had a much higher PCATa as compared to the low NCP group (−69.6±10.0 versus −73.5±10.6 HU). In a longitudinal study (47), PCATa was positively correlated with total plaque burden. Changes in PCATa were strongly correlated with changes in NCP and after adjustment of many confounding variables, baseline PCATa was independently associated with an increased NCP burden within the RCA.
In summary, CAT is strongly associated with plaque burden, especially with NCP. EAT has been demonstrated to initiate the process of atherosclerosis with the buildup of NCP while PCAT can track the inflammatory process ongoing within NCP. Therefore, CAT may help to quantitatively assess the disease load of CAD, as well as predict its risk of poor prognosis.
CAT and high-risk plaque (HRP)
Beyond plaque morphology and plaque burden described above, MDCT also allows the assessment of HRP. MDCT detects four main vulnerable plaque features: positive remodeling (PR), low attenuation (<30 HU), napkin ring sign, and spotty calcium. A study by Motoyama et al. showed that either one or two HRP features were found to independently predict acute coronary syndrome (ACS) (48). These particular plaques have been found to be prone to plaque rupture, which causes slow or arrested blood flow, ultimately leading to acute coronary events (49). In the past decade, EAT and PCAT have been linked with these vulnerable plaque characteristics (4,50,51).
EAT attenuation (EATa) and EATv are both independently associated with the presence of HRP (50). Subjects in the HRP cohort had higher values of both EATa and EATv as compared to those in the non-HRP cohort. For each standard deviation rise in EATa, the risk of HRP was three times higher compared to non-HRP [EATa (per 1 SD) odds ratio (OR) =3.120, 95% confidence interval (CI): 2.147–4.533]. Likewise, for each standard deviation increment in EATv, the risk of HRP was one and a half times higher than that of non-HRP [EATv (per 1 SD) OR =1.499, 95% CI: 1.068–1.965]. EATa demonstrated a higher predictive value than EATv for the occurrence of vulnerable plaques (AUC =0.761 vs. 0.606, 95% CI: 0.701–0.822 vs. 0.534–0.678). As described earlier, inflammation of the coronary artery wall affects the EAT surrounding the vessels, causing a change in its density (16). Franssens et al. reported an increase in adipose density around HRP versus stable plaque, along with an increase in macrophage population in the EAT linked with the formation of HRP (52). Furthermore, it was noteworthy to discover that EATv did not correlate linearly with EATa. EATv increased in subjects with CAD, while EATa decreased initially, and then increased thereafter.
An increase in EATv was similarly associated with more prevalent HRP lesions in a longitudinal study of 517 non-obese CAD patients despite medical management of CAD risk factors (51). Patients with increased EATv on follow-up had more HRP lesions as compared to patients with constant EATv which displayed an almost similar number of HRP lesions. On the other hand, a decrease in EATv on follow-up was associated with fewer HRP lesions. EATv reversible association with HRP, potentially implies that therapeutic interventions can target EAT to decrease CAD risk. This is evidenced by animal models which have shown that surgical removal of EAT slowed CAD progression (53). Correspondingly, in a randomized control trial, patients treated with the GLP-1 analogue (Liraglutide) witnessed a decrease in EATv. This link has also been hypothesized to be behind the decrease in cardiovascular mortality associated with the drug (54).
PCATa is likewise correlated with HRP lesions found in patients with stable CAD who ultimately developed ACS (4). Both PCATa at the level of the lesion and the RCA showed lower attenuation values for patients with vulnerable plaque as compared to non-HRP (PCAT RCA: −80.7±6.50 vs. −84.2±8.09 HU; PCAT lesion: −79.6±7.86 vs. −84.2±10.3 HU). PCATa was found to be independently linked with HRP presence after multivariable logistic regression. PCATa, a surrogate marker of inflammation, suggests that HRP lesions have a higher inflammation component than stable plaque and thus are more likely to lead to a coronary event.
Finally in a meta-analysis by Nerlekar et al., which analyzed 9 observational studies with 3,772 subjects; low attenuation plaque (LAP) and PR were the two strongest HRP features to be correlated with EATv (55). HRP features detected by optical coherence tomography (OCT) such as lipid-rich plaque and macrophages at the culprit plaque also demonstrated a higher level of PCATa, showing that the relationship between HRP and PCAT is not specific to MDCT (49).
EAT and PCAT have been shown to correlate with the presence of HRP lesions. They have the potential to be new targets for the research and development of future pharmacological interventions aimed at CAD prevention.
CAT and major adverse cardiovascular events (MACE)
MACE definition in research is variable. It includes myocardial infarction (MI), stroke, and cardiovascular death; and may also extend to and revascularization procedures, unstable angina and heart failure. A meta-analysis of 29 articles with varied patient demography illustrated the robustness of EATt and EATv as powerful biomarkers in predicting MACE outcomes in CAD patients (56). Multi-cox analysis adjusted for CAC and ASCVD score showed that EATa [hazard ratio (HR) 0.8, 95% CI: 0.7–0.98] is more correlated to MACE than EATv (HR 1.5, 95% CI: 1.1–2.1) (57). Lower CT attenuation values are linked with adipose tissue hypertrophy and hyperplasia. This hypertrophy in adipocytes leads to a decrease in capillary density within the tissue inducing hypoxia and necrosis finally cascading into inflammation affecting the neighboring coronary arteries (58).
Likewise, PCAT metrics have further been shown to forecast MACE. In the CRISP-CT study, perivascular FAI around the RCA provided a significant prognostic value in stratifying patients at risk for cardiac mortality, even above CAC, vessel stenosis assessment, HRP, and traditional CV risk factors (59). A cut-off value of >−70.1 HU was linked with an abrupt rise in cardiac mortality [HR 9.04, 95% CI: 3.35–24.40]. Additionally, Pergola V. et al. demonstrated that a mean FAI ≥−70 HU, is associated with an increase in cardiovascular mortality and heart catheterization procedures (P=0.05) in stable patients with non-obstructive CAD. Multivariate analysis corrected for typical CAD risk factors revealed that mean FAI was found to be independently linked with MACE (60). Aspirin and statins nullified perivascular FAI predictive values for cardiac mortality (adjusted HR 2.85, 95% CI: 0.44–18.49). Aspirin reduces vascular inflammation and enhances the stability of atherosclerotic plaque, contributing to its antiatherogenic effect (61). Similarly, statin, through its pleiotropic effects decreases inflammation within the coronary plaques and stabilizes them (62). Statins have been shown in a long-term study to increase the calcified component of atherosclerotic plaques while decreasing the non-calcified component, possibly highlighting the plaque’s resilience to rupture (63). Aspirin and statins decrease the risk for MACE, thus unequivocally compromising the ability of FAI to foretell coronary events. On the other hand, for subjects who did not take these medications, perivascular FAI remained a powerful predictor of cardiac mortality (adjusted HR 18.71, 95% CI: 2.01–174.04).
A recent study by Tzolos et al. also showed that PCATa and LAP burden had significant and incremental value in predicting fatal and non-fatal MI (64). Receiver operator curve analysis showed that LAP burden had a higher area under the curve (AUC) compared to PCATa for prediction of MI [0.71 (0.62–0.80) against 0.64 (0.54–0.74)], with increased AUC when the two measures were combined [0.75 (0.65–0.85)]. Additionally, PCATaRCA was higher in patients with obstructive disease, presence of plaque, and HRP anywhere in the coronary vasculature. This implies that PCATa is representative of the global state of the coronary tree rather than representing specific local effects of CAD. Furthermore, van Diemen et al showed that plaque burden and ischemia had a more significant correlation with clinical outcomes as compared to PCATa (65). PCATa is also known to be affected by scan parameters such as tube voltage and image reconstruction techniques (66). PCATa cannot be solely used for stratifying patients at risk of coronary events, but rather as an incremental predictor of MACE.
Both EAT and PCAT have been proven to be equal predictors of MACE. FAI is the only CAT metric that has found a reliable cut-off for forecasting MACE. As the main predictor of MACE, the anatomic assessment of stenosis and plaque is still superior to the functional assessment of CAT. Further studies are needed to evaluate the incremental value of FAI in predicting the risk of MACE in patients with CAD, especially those with non-obstructive lesions.
Future perspectives
CAT metrics are correlated with many aspects of CAD. They are involved in the synthesis of new plaques, atherosclerosis burden, plaque development, vulnerable plaques, MACE, and are also major risk factors for CAD. Therefore, assessment of these adipose tissues is bound to provide additional information on a patient’s CAD status beyond the current benchmarks. The main questions that lie ahead are:
Firstly, how to integrate CAT evaluation with existing assessment systems such as CAC score add valuable information for the patient. CAC currently provides information about the burden of calcified plaque which is a marker of established atherosclerosis, guiding health management of asymptomatic subjects based on their risk levels. However, it fails to identify NCP which is also a critical predictor of coronary events. CAT metrics identify coronary inflammation, a crucial process in the progression of atherosclerosis and subsequent MACE. This ongoing inflammation happening within coronary arteries may precede calcification. Thus, the inclusion of both CAC and FAI in clinical practice would provide a more comprehensive CAD risk assessment combining the current standard CAC score along with the novel FAI metric.
Secondly, there is no unified technical approach to fat quantification and analysis, which may lead to a lack of consistency between the results (56). Big data-based trained artificial intelligence (AI) can help speed up the segmentation of fat. This will help standardize future CAT metrics measurements. Additionally, radiomics, which is the extraction of quantitative metrics from radiological studies, can mine more relevant and predictive indicators.
Conclusions
PCAT and EAT are involved in the pathogenesis of atherosclerosis. EAT has been demonstrated to be involved in the early stages of plaque formation. PCAT highlights local inflammation within coronary artery plaques. FAI is a new biomarker of CAD based on PCAT. Combining these potential CAT indicators with existing evaluation methods, such as CAC scores, will help in the stratified management, precise treatment and prognosis of CAD patients. How to achieve rapid and accurate analysis and measurement of these indicators is an important issue that needs to be resolved before their clinical application.
Acknowledgments
Funding: None.
Footnote
Reporting Checklist: The authors have completed the Narrative Review reporting checklist. Available at https://qims.amegroups.com/article/view/10.21037/qims-24-1245/rc
Conflicts of Interest: All authors have completed the ICMJE uniform disclosure form (available at https://qims.amegroups.com/article/view/10.21037/qims-24-1245/coif). The authors have no conflicts of interest to declare.
Ethical Statement: The authors are accountable for all aspects of the work in ensuring that questions related to the accuracy or integrity of any part of the work are appropriately investigated and resolved. This review paper was conducted with the approval of the Medical Ethics Committee of the Second Affiliated Hospital of Zhejiang University School of Medicine [IRB approval number (2024) (0566)].
Open Access Statement: This is an Open Access article distributed in accordance with the Creative Commons Attribution-NonCommercial-NoDerivs 4.0 International License (CC BY-NC-ND 4.0), which permits the non-commercial replication and distribution of the article with the strict proviso that no changes or edits are made and the original work is properly cited (including links to both the formal publication through the relevant DOI and the license). See: https://creativecommons.org/licenses/by-nc-nd/4.0/.
References
- Centers for Disease Control and Prevention. National Center for Health Statistics Mortality Data on CDC Wonder CDC. 2022. Available online: https://wonder.cdc.gov/mcd.html. Accessed May 31 2024.
- Gulati M, Levy PD, Mukherjee D, Amsterdam E, Bhatt DL, Birtcher KK, et al. 2021 AHA/ACC/ASE/CHEST/SAEM/SCCT/SCMR Guideline for the Evaluation and Diagnosis of Chest Pain: A Report of the American College of Cardiology/American Heart Association Joint Committee on Clinical Practice Guidelines. Circulation 2021;144:e368-454. [Crossref] [PubMed]
- Waksman R, Di Mario C, Torguson R, Ali ZA, Singh V, Skinner WH, et al. Identification of patients and plaques vulnerable to future coronary events with near-infrared spectroscopy intravascular ultrasound imaging: a prospective, cohort study. Lancet 2019;394:1629-37. [Crossref] [PubMed]
- Yuvaraj J, Lin A, Nerlekar N, Munnur RK, Cameron JD, Dey D, Nicholls SJ, Wong DTL. Pericoronary Adipose Tissue Attenuation Is Associated with High-Risk Plaque and Subsequent Acute Coronary Syndrome in Patients with Stable Coronary Artery Disease. Cells 2021;10:1143. [Crossref] [PubMed]
- Yu W, Chen Y, Zhang F, Liu B, Wang J, Shao X, Yang X, Shi Y, Wang Y. Association of epicardial adipose tissue volume with increased risk of hemodynamically significant coronary artery disease. Quant Imaging Med Surg 2023;13:2582-93. [Crossref] [PubMed]
- Zou L, Xiao X, Jia Y, Yin F, Zhu J, Gao Q, Xue M, Dong S. Predicting coronary atherosclerosis heart disease with pericoronary adipose tissue attenuation parameters based on dual-layer spectral detector computed tomography: a preliminary exploration. Quant Imaging Med Surg 2023;13:2975-88. [Crossref] [PubMed]
- Monti CB, Codari M, De Cecco CN, Secchi F, Sardanelli F, Stillman AE. Novel imaging biomarkers: epicardial adipose tissue evaluation. Br J Radiol 2020;93:20190770. [Crossref] [PubMed]
- Iacobellis G. Epicardial adipose tissue in contemporary cardiology. Nat Rev Cardiol 2022;19:593-606. [Crossref] [PubMed]
- Sacks HS, Fain JN, Holman B, Cheema P, Chary A, Parks F, Karas J, Optican R, Bahouth SW, Garrett E, Wolf RY, Carter RA, Robbins T, Wolford D, Samaha J. Uncoupling protein-1 and related messenger ribonucleic acids in human epicardial and other adipose tissues: epicardial fat functioning as brown fat. J Clin Endocrinol Metab 2009;94:3611-5. [Crossref] [PubMed]
- Marchington JM, Mattacks CA, Pond CM. Adipose tissue in the mammalian heart and pericardium: structure, foetal development and biochemical properties. Comp Biochem Physiol B 1989;94:225-32. [Crossref] [PubMed]
- Engin AB. What Is Lipotoxicity? Adv Exp Med Biol 2017;960:197-220. [Crossref] [PubMed]
- Li R, Wang WQ, Zhang H, Yang X, Fan Q, Christopher TA, Lopez BL, Tao L, Goldstein BJ, Gao F, Ma XL. Adiponectin improves endothelial function in hyperlipidemic rats by reducing oxidative/nitrative stress and differential regulation of eNOS/iNOS activity. Am J Physiol Endocrinol Metab 2007;293:E1703-8. [Crossref] [PubMed]
- Karastergiou K, Evans I, Ogston N, Miheisi N, Nair D, Kaski JC, Jahangiri M, Mohamed-Ali V. Epicardial adipokines in obesity and coronary artery disease induce atherogenic changes in monocytes and endothelial cells. Arterioscler Thromb Vasc Biol 2010;30:1340-6. [Crossref] [PubMed]
- Hirata Y, Kurobe H, Akaike M, Chikugo F, Hori T, Bando Y, Nishio C, Higashida M, Nakaya Y, Kitagawa T, Sata M. Enhanced inflammation in epicardial fat in patients with coronary artery disease. Int Heart J 2011;52:139-42. [Crossref] [PubMed]
- Raman P, Khanal S. Leptin in Atherosclerosis: Focus on Macrophages, Endothelial and Smooth Muscle Cells. Int J Mol Sci 2021;22:5446. [Crossref] [PubMed]
- Antonopoulos AS, Sanna F, Sabharwal N, Thomas S, Oikonomou EK, Herdman L, et al. Detecting human coronary inflammation by imaging perivascular fat. Sci Transl Med 2017;9:eaal2658. [Crossref] [PubMed]
- Tan N, Dey D, Marwick TH, Nerlekar N. Pericoronary Adipose Tissue as a Marker of Cardiovascular Risk: JACC Review Topic of the Week. J Am Coll Cardiol 2023;81:913-23. [Crossref] [PubMed]
- Kim HW, Belin de Chantemèle EJ, Weintraub NL. Perivascular Adipocytes in Vascular Disease. Arterioscler Thromb Vasc Biol 2019;39:2220-7. [Crossref] [PubMed]
- Qi XY, Qu SL, Xiong WH, Rom O, Chang L, Jiang ZS. Perivascular adipose tissue (PVAT) in atherosclerosis: a double-edged sword. Cardiovasc Diabetol 2018;17:134. [Crossref] [PubMed]
- El Khoudary SR, Shields KJ, Janssen I, Hanley C, Budoff MJ, Barinas-Mitchell E, Everson-Rose SA, Powell LH, Matthews KA. Cardiovascular Fat, Menopause, and Sex Hormones in Women: The SWAN Cardiovascular Fat Ancillary Study. J Clin Endocrinol Metab 2015;100:3304-12. [Crossref] [PubMed]
- Eroğlu S. How do we measure epicardial adipose tissue thickness by transthoracic echocardiography? Anatol J Cardiol 2015;15:416-9. [Crossref] [PubMed]
- Napoli G, Pergola V, Basile P, De Feo D, Bertrandino F, Baggiano A, Mushtaq S, Fusini L, Fazzari F, Carrabba N, Rabbat MG, Motta R, Ciccone MM, Pontone G, Guaricci AI. Epicardial and Pericoronary Adipose Tissue, Coronary Inflammation, and Acute Coronary Syndromes. J Clin Med 2023;12:7212. [Crossref] [PubMed]
- Guglielmo M, Lin A, Dey D, Baggiano A, Fusini L, Muscogiuri G, Pontone G. Epicardial fat and coronary artery disease: Role of cardiac imaging. Atherosclerosis 2021;321:30-8. [Crossref] [PubMed]
- Goeller M, Achenbach S, Duncker H, Dey D, Marwan M. Imaging of the Pericoronary Adipose Tissue (PCAT) Using Cardiac Computed Tomography: Modern Clinical Implications. J Thorac Imaging 2021;36:149-61. [Crossref] [PubMed]
- Commandeur F, Goeller M, Betancur J, Cadet S, Doris M, Chen X, Berman DS, Slomka PJ, Tamarappoo BK, Dey D. Deep Learning for Quantification of Epicardial and Thoracic Adipose Tissue From Non-Contrast CT. IEEE Trans Med Imaging 2018;37:1835-46. [Crossref] [PubMed]
- Ma R, Fari R, van der Harst P, N, De Cecco C, E, Stillman A, Vliegenthart R, van Assen M. Evaluation of pericoronary adipose tissue attenuation on CT. Br J Radiol 2023;96:20220885. [Crossref] [PubMed]
- Klüner LV, Oikonomou EK, Antoniades C. Assessing Cardiovascular Risk by Using the Fat Attenuation Index in Coronary CT Angiography. Radiol Cardiothorac Imaging 2021;3:e200563. [Crossref] [PubMed]
- Eroglu S, Sade LE, Yildirir A, Bal U, Ozbicer S, Ozgul AS, Bozbas H, Aydinalp A, Muderrisoglu H. Epicardial adipose tissue thickness by echocardiography is a marker for the presence and severity of coronary artery disease. Nutr Metab Cardiovasc Dis 2009;19:211-7. [Crossref] [PubMed]
- Iacobellis G, Ribaudo MC, Assael F, Vecci E, Tiberti C, Zappaterreno A, Di Mario U, Leonetti F. Echocardiographic epicardial adipose tissue is related to anthropometric and clinical parameters of metabolic syndrome: a new indicator of cardiovascular risk. J Clin Endocrinol Metab 2003;88:5163-8. [Crossref] [PubMed]
- Wu FZ, Chou KJ, Huang YL, Wu MT. The relation of location-specific epicardial adipose tissue thickness and obstructive coronary artery disease: systemic review and meta-analysis of observational studies. BMC Cardiovasc Disord 2014;14:62. [Crossref] [PubMed]
- Gorter PM, van Lindert AS, de Vos AM, Meijs MF, van der Graaf Y, Doevendans PA, Prokop M, Visseren FL. Quantification of epicardial and peri-coronary fat using cardiac computed tomography; reproducibility and relation with obesity and metabolic syndrome in patients suspected of coronary artery disease. Atherosclerosis 2008;197:896-903. [Crossref] [PubMed]
- Gorter PM, de Vos AM, van der Graaf Y, Stella PR, Doevendans PA, Meijs MF, Prokop M, Visseren FL. Relation of epicardial and pericoronary fat to coronary atherosclerosis and coronary artery calcium in patients undergoing coronary angiography. Am J Cardiol 2008;102:380-5. [Crossref] [PubMed]
- Mahabadi AA, Reinsch N, Lehmann N, Altenbernd J, Kälsch H, Seibel RM, Erbel R, Möhlenkamp S. Association of pericoronary fat volume with atherosclerotic plaque burden in the underlying coronary artery: a segment analysis. Atherosclerosis 2010;211:195-9. [Crossref] [PubMed]
- Mazurek T, Kobylecka M, Zielenkiewicz M, Kurek A, Kochman J, Filipiak KJ, Mazurek K, Huczek Z, Królicki L, Opolski G. PET/CT evaluation of (18)F-FDG uptake in pericoronary adipose tissue in patients with stable coronary artery disease: Independent predictor of atherosclerotic lesions' formation? J Nucl Cardiol 2017;24:1075-84. [Crossref] [PubMed]
- El Khoudary SR, Shields KJ, Janssen I, Budoff MJ, Everson-Rose SA, Powell LH, Matthews KA. Postmenopausal Women With Greater Paracardial Fat Have More Coronary Artery Calcification Than Premenopausal Women: The Study of Women's Health Across the Nation (SWAN) Cardiovascular Fat Ancillary Study. J Am Heart Assoc 2017;6:e004545. [Crossref] [PubMed]
- El Khoudary SR, Venugopal V, Manson JE, Brooks MM, Santoro N, Black DM, Harman M, Naftolin F, Hodis HN, Brinton EA, Miller VM, Taylor HS, Budoff MJ. Heart fat and carotid artery atherosclerosis progression in recently menopausal women: impact of menopausal hormone therapy: The KEEPS trial. Menopause 2020;27:255-62. [Crossref] [PubMed]
- Shreya D, Zamora DI, Patel GS, Grossmann I, Rodriguez K, Soni M, Joshi PK, Patel SC, Sange I. Coronary Artery Calcium Score - A Reliable Indicator of Coronary Artery Disease? Cureus 2021;13:e20149. [Crossref] [PubMed]
- Greenland P, Bonow RO, Brundage BH, Budoff MJ, Eisenberg MJ, Grundy SM, Lauer MS, Post WS, Raggi P, Redberg RF, Rodgers GP, Shaw LJ, Taylor AJ, Weintraub WS. ACCF/AHA 2007 clinical expert consensus document on coronary artery calcium scoring by computed tomography in global cardiovascular risk assessment and in evaluation of patients with chest pain: a report of the American College of Cardiology Foundation Clinical Expert Consensus Task Force (ACCF/AHA Writing Committee to Update the 2000 Expert Consensus Document on Electron Beam Computed Tomography) developed in collaboration with the Society of Atherosclerosis Imaging and Prevention and the Society of Cardiovascular Computed Tomography. J Am Coll Cardiol 2007;49:378-402. [Crossref] [PubMed]
- Alexopoulos N, McLean DS, Janik M, Arepalli CD, Stillman AE, Raggi P. Epicardial adipose tissue and coronary artery plaque characteristics. Atherosclerosis 2010;210:150-4. [Crossref] [PubMed]
- Bettencourt N, Toschke AM, Leite D, Rocha J, Carvalho M, Sampaio F, Xará S, Leite-Moreira A, Nagel E, Gama V. Epicardial adipose tissue is an independent predictor of coronary atherosclerotic burden. Int J Cardiol 2012;158:26-32. [Crossref] [PubMed]
- Yerramasu A, Dey D, Venuraju S, Anand DV, Atwal S, Corder R, Berman DS, Lahiri A. Increased volume of epicardial fat is an independent risk factor for accelerated progression of sub-clinical coronary atherosclerosis. Atherosclerosis 2012;220:223-30. [Crossref] [PubMed]
- Mahabadi AA, Berg MH, Lehmann N, Kälsch H, Bauer M, Kara K, Dragano N, Moebus S, Jöckel KH, Erbel R, Möhlenkamp S. Association of epicardial fat with cardiovascular risk factors and incident myocardial infarction in the general population: the Heinz Nixdorf Recall Study. J Am Coll Cardiol 2013;61:1388-95. [Crossref] [PubMed]
- Greif M, Becker A, von Ziegler F, Lebherz C, Lehrke M, Broedl UC, Tittus J, Parhofer K, Becker C, Reiser M, Knez A, Leber AW. Pericardial adipose tissue determined by dual source CT is a risk factor for coronary atherosclerosis. Arterioscler Thromb Vasc Biol 2009;29:781-6. [Crossref] [PubMed]
- Hwang IC, Park HE, Choi SY. Epicardial Adipose Tissue Contributes to the Development of Non-Calcified Coronary Plaque: A 5-Year Computed Tomography Follow-up Study. J Atheroscler Thromb 2017;24:262-74. [Crossref] [PubMed]
- Iacobellis G. Local and systemic effects of the multifaceted epicardial adipose tissue depot. Nat Rev Endocrinol 2015;11:363-71. [Crossref] [PubMed]
- Suzuki K, Kinoshita D, Yuki H, Niida T, Sugiyama T, Yonetsu T, Araki M, Nakajima A, Seegers LM, Dey D, Lee H, McNulty I, Takano M, Kakuta T, Mizuno K, Jang IK. Higher Noncalcified Plaque Volume Is Associated With Increased Plaque Vulnerability and Vascular Inflammation. Circ Cardiovasc Imaging 2024;17:e015769. [Crossref] [PubMed]
- Goeller M, Tamarappoo BK, Kwan AC, Cadet S, Commandeur F, Razipour A, Slomka PJ, Gransar H, Chen X, Otaki Y, Friedman JD, Cao JJ, Albrecht MH, Bittner DO, Marwan M, Achenbach S, Berman DS, Dey D. Relationship between changes in pericoronary adipose tissue attenuation and coronary plaque burden quantified from coronary computed tomography angiography. Eur Heart J Cardiovasc Imaging 2019;20:636-43. [Crossref] [PubMed]
- Motoyama S, Sarai M, Harigaya H, Anno H, Inoue K, Hara T, Naruse H, Ishii J, Hishida H, Wong ND, Virmani R, Kondo T, Ozaki Y, Narula J. Computed tomographic angiography characteristics of atherosclerotic plaques subsequently resulting in acute coronary syndrome. J Am Coll Cardiol 2009;54:49-57. [Crossref] [PubMed]
- Narula J, Garg P, Achenbach S, Motoyama S, Virmani R, Strauss HW. Arithmetic of vulnerable plaques for noninvasive imaging. Nat Clin Pract Cardiovasc Med 2008;5:S2-10. [Crossref] [PubMed]
- Gao Z, Zuo Y, Jia L, Yin Y, Yang X, Fan Y, Liu H. Association between epicardial adipose tissue density and characteristics of coronary plaques assessed by coronary computed tomographic angiography. Int J Cardiovasc Imaging 2022;38:673-81. [Crossref] [PubMed]
- Nakanishi K, Fukuda S, Tanaka A, Otsuka K, Jissho S, Taguchi H, Yoshikawa J, Shimada K. Persistent epicardial adipose tissue accumulation is associated with coronary plaque vulnerability and future acute coronary syndrome in non-obese subjects with coronary artery disease. Atherosclerosis 2014;237:353-60. [Crossref] [PubMed]
- Franssens BT, Nathoe HM, Leiner T, van der Graaf Y, Visseren FL. Relation between cardiovascular disease risk factors and epicardial adipose tissue density on cardiac computed tomography in patients at high risk of cardiovascular events. Eur J Prev Cardiol 2017;24:660-70. [Crossref] [PubMed]
- McKenney-Drake ML, Rodenbeck SD, Bruning RS, Kole A, Yancey KW, Alloosh M, Sacks HS, Sturek M. Epicardial Adipose Tissue Removal Potentiates Outward Remodeling and Arrests Coronary Atherogenesis. Ann Thorac Surg 2017;103:1622-30. [Crossref] [PubMed]
- Iacobellis G, Mohseni M, Bianco SD, Banga PK. Liraglutide causes large and rapid epicardial fat reduction. Obesity (Silver Spring) 2017;25:311-6. [Crossref] [PubMed]
- Nerlekar N, Brown AJ, Muthalaly RG, Talman A, Hettige T, Cameron JD, Wong DTL. Association of Epicardial Adipose Tissue and High-Risk Plaque Characteristics: A Systematic Review and Meta-Analysis. J Am Heart Assoc 2017;6:e006379. [Crossref] [PubMed]
- Chong B, Jayabaskaran J, Ruban J, Goh R, Chin YH, Kong G, Ng CH, Lin C, Loong S, Muthiah MD, Khoo CM, Shariff E, Chan MY, Lajeunesse-Trempe F, Tchernof A, Chevli P, Mehta A, Mamas MA, Dimitriadis GK, Chew NWS. Epicardial Adipose Tissue Assessed by Computed Tomography and Echocardiography Are Associated With Adverse Cardiovascular Outcomes: A Systematic Review and Meta-Analysis. Circ Cardiovasc Imaging 2023;16:e015159. [Crossref] [PubMed]
- Goeller M, Achenbach S, Marwan M, Doris MK, Cadet S, Commandeur F, Chen X, Slomka PJ, Gransar H, Cao JJ, Wong ND, Albrecht MH, Rozanski A, Tamarappoo BK, Berman DS, Dey D. Epicardial adipose tissue density and volume are related to subclinical atherosclerosis, inflammation and major adverse cardiac events in asymptomatic subjects. J Cardiovasc Comput Tomogr 2018;12:67-73. [Crossref] [PubMed]
- Drager LF, Yao Q, Hernandez KL, Shin MK, Bevans-Fonti S, Gay J, Sussan TE, Jun JC, Myers AC, Olivecrona G, Schwartz AR, Halberg N, Scherer PE, Semenza GL, Powell DR, Polotsky VY. Chronic intermittent hypoxia induces atherosclerosis via activation of adipose angiopoietin-like 4. Am J Respir Crit Care Med 2013;188:240-8. [Crossref] [PubMed]
- Oikonomou EK, Marwan M, Desai MY, Mancio J, Alashi A, Hutt Centeno E, et al. Non-invasive detection of coronary inflammation using computed tomography and prediction of residual cardiovascular risk (the CRISP CT study): a post-hoc analysis of prospective outcome data. Lancet 2018;392:929-39. [Crossref] [PubMed]
- Pergola V, Cabrelle G, Mattesi G, Cattarin S, Furlan A, Dellino CM, Continisio S, Montonati C, Giorgino A, Giraudo C, Leoni L, Bariani R, Barbiero G, Bauce B, Mele D, Perazzolo Marra M, De Conti G, Iliceto S, Motta R. Added Value of CCTA-Derived Features to Predict MACEs in Stable Patients Undergoing Coronary Computed Tomography. Diagnostics (Basel) 2022.
- Cyrus T, Sung S, Zhao L, Funk CD, Tang S, Praticò D. Effect of low-dose aspirin on vascular inflammation, plaque stability, and atherogenesis in low-density lipoprotein receptor-deficient mice. Circulation 2002;106:1282-7. [Crossref] [PubMed]
- Diamantis E, Kyriakos G, Quiles-Sanchez LV, Farmaki P, Troupis T. The Anti-Inflammatory Effects of Statins on Coronary Artery Disease: An Updated Review of the Literature. Curr Cardiol Rev 2017;13:209-16. [Crossref] [PubMed]
- Mátyás BB, Benedek I, Raț N, Blîndu E, Parajkó Z, Mihăilă T, Benedek T. Assessing the Impact of Long-Term High-Dose Statin Treatment on Pericoronary Inflammation and Plaque Distribution-A Comprehensive Coronary CTA Follow-Up Study. Int J Mol Sci 2024;25:1700. [Crossref] [PubMed]
- Tzolos E, Williams MC, McElhinney P, Lin A, Grodecki K, Flores Tomasino G, et al. Pericoronary Adipose Tissue Attenuation, Low-Attenuation Plaque Burden, and 5-Year Risk of Myocardial Infarction. JACC Cardiovasc Imaging 2022;15:1078-88. [Crossref] [PubMed]
- van Diemen PA, Bom MJ, Driessen RS, Schumacher SP, Everaars H, de Winter RW, van de Ven PM, Freiman M, Goshen L, Heijtel D, Langzam E, Min JK, Leipsic JA, Raijmakers PG, van Rossum AC, Danad I, Knaapen P. Prognostic Value of RCA Pericoronary Adipose Tissue CT-Attenuation Beyond High-Risk Plaques, Plaque Volume, and Ischemia. JACC Cardiovasc Imaging 2021;14:1598-610. [Crossref] [PubMed]
- Etter D, Warnock G, Koszarski F, Niemann T, Mikail N, Bengs S, Buechel RR, Kaufmann P, Gebhard C, Rossi A. Towards universal comparability of pericoronary adipose tissue attenuation: a coronary computed tomography angiography phantom study. Eur Radiol 2023;33:2324-30. [Crossref] [PubMed]