Association between perforator stroke after middle cerebral artery elective stenting and arterial remodeling patterns: a high-resolution MRI-based retrospective cohort study
Introduction
Intracranial atherosclerotic stenosis (ICAS) is an important cause of ischemic stroke (1,2). For patients with ICAS for whom medical treatment has failed, endovascular treatment (EVT) is an effective alternative. However, patients undergoing EVT have an increased risk of perioperative complications, of which procedure-related perforator stroke (PS) is the most common (3). The Stenting and Aggressive Medical Management for Preventing Recurrent Stroke in Intracranial Stenosis (SAMMPRIS) trial reported that PS accounted for more than 60% of ischemic complications following EVT for ICAS (4). Consequently, it is critical to prevent procedure-related PS to improve the safety of EVT.
Arterial remodeling refers to a long-lasting alteration in the structure or composition of blood vessels (5), and comprises positive remodeling (PR), negative remodeling (NR), and intermediate remodeling (IR). PR refers to the compensatory outward expansion of the vessel size to preserve the lumen, while NR refers to the inward constriction of the vessel at the site of the lesion, exacerbating the loss of lumen (5), and IR is between the NR and PR. The pattern of arterial remodeling may be associated with the postoperative outcomes of patients with severe ICAS after EVT. Previous research has shown that NR in patients with severe basilar artery stenosis is associated with procedure-related PS (6). Several studies have shown that PR may be associated with increased plaque vulnerability, clinical symptoms, and a poor clinical outcome after coronary intervention, while NR may also increase the risk of perioperative complications during treatment (7-9).
High-resolution magnetic resonance imaging (HR-MRI) is a reproducible and effective technique that can be used to identify and characterize atherosclerotic lesions (8-12). It enables the examination of vascular remodeling patterns and other characteristics of plaque. A previous study (6) evaluated the association between arterial remodeling patterns and PS after stenting for basilar artery stenosis using HR-MRI; however, the applicability of these findings to middle cerebral artery (MCA) stenosis remains unknown.
In this study, we sought to use HR-MRI to evaluate plaque features in patients with symptomatic MCA stenosis, and to investigate the relationship between arterial remodeling patterns and PS after elective stenting in patients with severe MCA stenosis. We present this article in accordance with the STROBE reporting checklist (available at https://qims.amegroups.com/article/view/10.21037/qims-24-143/rc).
Methods
Subjects
The study was conducted in accordance with the Declaration of Helsinki (as revised in 2013). The study was approved by the Ethics Board of the First Affiliated Hospital of Zhengzhou University (2022-KY-0086-001), and the requirement of individual consent for this retrospective analysis was waived.
In this retrospective cohort study, we consecutively analyzed the clinical and surgical data of all patients with MCA atherosclerotic disease who underwent EVT at the First Affiliated Hospital of Zhengzhou University from January 2018 to June 2023. To be eligible for inclusion in this study, the patients had to meet the following inclusion criteria: (I) have symptomatic MCA atherosclerotic disease and be aged 18–80 years; (II) have a lesion with a degree of stenosis between 70% and 99% as confirmed by digital subtraction angiography (DSA); (III) have undergone HR-MRI before surgery; (IV) have one or more risk factors associated with atherosclerosis, including hypertension, hyperlipidemia, diabetes mellitus, and cigarette smoking; and (V) have a modified Rankin Scale (mRS) score ≤3. Patients were excluded from the study if they met any of the following exclusion criteria: (I) had suffered from an acute stroke caused by MCA occlusion; (II) had a non-atherosclerotic cause of MCA stenosis, such as Moyamoya disease, vasculitis, or vascular dissection; (III) had simultaneously undergone EVT for another intracranial and extracranial vessel disease; (IV) had incomplete clinical data or images; and/or (V) had uninterpretable images due to motion artifacts.
The baseline and treatment variable data of the patients were collected, including age, gender, vascular risk factors (e.g., hypertension, diabetes, hyperlipidemia, smoking history, and coronary heart disease), preoperative imaging characteristics, procedure details, National Institute of Health stroke scale (NIHSS) score (13), and mRS score (14) at admission.
HR-MRI protocols
In this study, all HR-MRI images were acquired and processed by operators with over five years of experience who were unaware of the patients’ clinical data. HR-MRI was performed on all patients using a 64-channel head/neck coil and a 3.0T magnetic resonance scanner (Magnetom Prisma, Siemens Healthineers, Germany). All vessel wall imaging (VWI) was performed on a three T MRI system (Magnetom Prisma, Siemens, Erlangen, Germany) with a 64-channel coil. The parameters for VWI were as follows: repetition time (TR): 900 ms; echo time (TE): 15.0 ms; field of view (FOV): 230 mm × 230 mm; matrix: 256×265; and spatial resolution: 0.6 mm (isotropic). The MRI protocol also included standard sequences, such as T1 weighted image (T1WI), T2 weighted image (T2WI), fluid-attenuated inversion recovery (FLAIR), diffusion weighted imaging (DWI), and a three-dimensional (3D) time-of-flight magnetic resonance angiography (TOF-MRA) sequence of the arteries above the aortic arch. Magnevist was used as the contract media (Bayer Schering Pharma AG, Germany). First, the TOF-MRA sequence was obtained by scanning. According to the stenosis segment of the susceptible lumen of the TOF-MRA, 3D T1W sampling perfection with application-optimized contrasts using a different flip-angle evolution (3D T1-SPACE) sequence was used to scan perpendicular to the target vessel. Axial, sagittal, coronal, and curved reconstructions of the 3D T1-SPACE images were obtained. The above procedure was repeated, and gadolinium diethylenetriaminepentaacetic acid was injected through a high-pressure syringe to obtain the enhanced T1-SPACE axial, sagittal, coronal, and curved plane reconstruction images. The parameters for HR-MRI were as follows: TR: 900 ms; TE: 15.0 ms; FOV: 230 mm × 230 mm; matrix: 256×265; and spatial resolution: 0.6 mm (isotropic). Curved reconstruction images were generated using Siemens post-processing software (Magnetom Prisma, Siemens, Erlangen, Germany).
Imaging analysis
All image assessments were conducted by two independent neuroradiologists (R.C. and C.L., with >7 and >15 years of experience, respectively), who were blinded to the clinical data of the patients. Any discrepancies were resolved by consensus. Lumen and outer wall boundaries were manually segmented on both the pre-contrast and post-contrast T1-weighted images using the medical imaging viewer software, RadiAnt Digital Imaging and Communications in Medicine (DICOM) Viewer (Medixant, Poland). The original data with the type of DICOM were required for the image analysis. The MCA cross-sectional area was measured at both the reference site and the maximal lumen narrowing site after zooming in 800%. The reference site was selected based on the Warfarin vs. Aspirin for Symptomatic Intracranial Disease (WASID) criteria (15).
The remodeling index was calculated as the ratio of the total vessel area at the maximal lumen narrowing site to that at the reference site. PR was defined as remodeling index values >1.05, IR as values between 0.95 and 1.05, and NR as values <0.95. Non-NR was defined as both PR and IR (16). Plaque area was calculated as follows: plaque area = total vessel area – lumen area. Plaque burden was measured on the maximal stenosis site, and was calculated as follows: plaque burden = (plaque area/vessel area) × 100% (11). Two straight lines in the axial section of the M1 segment lumen of the MCA were used to divide the lumen into the following four quadrants: superior, inferior, ventral, and dorsal (17). Plaque was classified as belonging to the quadrant that had the thickest part of the plaque. To normalize signal intensity, the contrast-enhancement ratio was calculated at the slice with the greatest enhancement using adjacent brain parenchyma. The enhancement ratio was calculated as follows: enhancement ratio = [signal of plaque (post-contrast)/signal of brain parenchyma (post-contrast)]/[signal of plaque (pre-contrast)/signal of brain parenchyma (pre-contrast)] × 100% (11). Preoperative perforator infarction in the MCA territory was defined as DWI showing infarctions in the putamen, globus pallidus, caudate head and body, anterior and posterior (proximal) internal capsules, and corona radiata around the ventricles (18).
DSA was routinely performed before surgery to determine the stenotic degree and lesion length. Steel beads were placed on the patients’ heads or faces as a reference to calculate the stenotic degree and vessel diameter of the lesion. The length of the lesion was defined as the distance between the proximal and distal filling defects. The vessel diameter was defined as the minimum distance between the upper and lower walls of the vessel lumen. The degree of stenosis was determined using the (WASID) method (15). The Mori type was categorized as Mori A, Mori B and Mori C as per the criteria of previous studies (19).
Endovascular procedure and analysis
All patients received standard dual antiplatelet treatment (clopidogrel 75 mg/d, aspirin 100 mg/d) for at least 5 days before EVT, and the thromboelastographic results (arachidonic acid >50%, adenosine diphosphate >30%) reached the standard. All lesion sites were treated by neurointerventionalists with over 10 years of clinical experience. Under general anesthesia, the femoral artery was punctured using the modified Seldinger technique, a 6F artery sheath and a 6F Envoy guide catheter were inserted, and 1 mg/kg of heparin sodium was injected intravenously to achieve systemic heparinization. The imaging position was selected once more, the stenosis degree of the responsible vessel was determined, and the diameter of the vessel was measured. First, a 200-centimeter-long micro-guidewire was used to cross the lesion segments with an microcatheter, and the microcatheter’s distal segment was advanced to the distal branch of the target vessel. The 300-centimeter micro-guidewire and microcatheter were exchanged, and the balloon catheter was inserted into the stenosis. The diameter of the balloon was chosen based on the diameter of the target lesion, and the normal diameter of the stenosis’ proximal end was between 50% and 80%. After angiography confirmed that the balloon was across the lesion, the balloon was steadily inflated under fluoroscopy for at least 3 minutes until the lesion segment was dilated by working pressure. The balloon was then withdrawn for angiography. If the procedure was successful, the micro-guidewire was fixed and the balloon catheter was removed, and the results were observed by angiography 15 minutes later. If the vascular lumen was unobstructed without recoil, the procedure was concluded. If there was dissection or obvious vessel wall recoil, the self-expanding stent was placed through the microcatheter along the micro-guidewire. After crossing the stenosis segment and releasing the stent, balloon dilation was conducted if the residual stenosis rate was still 50%. Angiography was then performed to confirm that cerebral perfusion was normal, after which the procedure concluded.
Immediately after the procedure, Xper computed tomography (CT) was conducted to check for cerebral hemorrhage, and vasco CT was performed to monitor stent patency. Successful stent placement was defined as stent coverage of the target lesion entirely, adequate adherence, an absence of stent filling defects, and residual stenosis ≤50%. Anticoagulation with heparin of low molecular weight was administered for 2 to 3 days following the procedure, and blood pressure was closely monitored for 3 to 5 days to prevent hyperperfusion. After discharge, dual oral antiplatelet medications were continued for to 6 months. If no restenosis occurred during follow-up and other stroke risk factors were under control, the patient’s oral antiplatelet treatment was modified.
Outcomes
The outcomes of the patients were evaluated using the mRS score. The primary endpoint was the incidence of procedure-related PS. Procedure-related PS is characterized by acute neurological symptoms (e.g., hemiplegia or upper limb monoplegia, motor aphasia, and unilateral facial paresis) corresponding to the area of the MCA perforator artery that occur during or shortly after the procedure, with CT angiography ruling out occlusion of the MCA main stem. Brain CT or MRI can be performed to reveal new infarction lesions or enlarged infarctions (18,20). The secondary endpoint was any symptomatic stroke or death within 30 days.
Statistical analysis
The statistical data in the study were analyzed and processed using SPSS 25.0 (IBM Corporation, Armonk, New York, USA). The data were assessed for normality using the Shapiro-Wilk test. The continuous variables are presented as the mean ± standard deviation (SD) for the normally distributed data, and the median with the interquartile range (IQR) for the non-normally distributed data. The categorical variables are presented as the percentage. To assess interrater reliability, the intraclass correlation coefficient (ICC) was applied for the plaque area, enhancement ratio, and remodeling index (21). The Kappa statistic was applied for the interrater assessment of the lesion distribution and remodeling patterns (22). Differences in the categorical variables between the NR and non-NR patients were assessed using the Chi-square test (χ2) or Fisher’s exact test. Differences in the continuous variables between the two groups were assessed using the Student t-test or Mann-Whitney U-test. A two-sided P value <0.05 indicated statistical significance.
Results
Patient baseline features
In total, 65 patients with symptomatic MCA stenosis were included in the study. A flow chart of the selection criteria for the study is shown in Figure 1. Of the patients, 34 were male and 31 were female. The patients had an average age of 57.6±8.7 years. Based on the arterial remodeling patterns, 45 (69.2%) patients were allocated to the NR group, and 20 (30.8%) to the non-NR group. There were no significant differences between the two groups in terms of the baseline characteristics (e.g., gender, age, risk factors, and preoperative mRS scores) (Table 1).
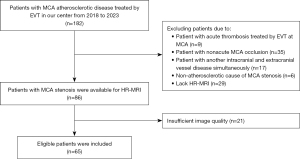
Table 1
Variable | Total (n=65) | NR (n=45) | NNR (n=20) | P value |
---|---|---|---|---|
Male sex | 34 (52.3) | 26 (57.8) | 8 (40.0) | 0.185 |
Age, years | 57.6±8.7 | 56.8±9.3 | 59.4±7.2 | 0.290 |
Hypertension | 37 (56.9) | 27 (60.0) | 10 (50.0) | 0.452 |
Diabetes | 21 (32.3) | 15 (33.3) | 6 (30.0) | 0.791 |
Hyperlipidemia | 29 (44.6) | 19 (42.2) | 10 (50.0) | 0.560 |
Smoking | 23 (35.4) | 18 (40.0) | 5 (25.0) | 0.243 |
Coronary artery disease | 6 (9.2) | 5 (11.1) | 1 (5.0) | 0.657 |
mRS score | 1 [0–2] | 1 [0–2] | 0 [0–2] | 0.596 |
NIHSS score | 1 [0–2] | 1 [0–3] | 1 [0–2] | 0.436 |
Time from latest ischemic event to procedure, d | 21 [15–35] | 21 (15–35) | 19.5 [15–25] | 0.564 |
Responsible artery | 0.702 | |||
LMCA | 40 (61.5) | 27 (60.0) | 18 (40.0) | |
RMCA | 25 (38.5) | 13 (65.0) | 7 (35.0) | |
Preoperative perforator infarction | 27 (41.5) | 20 (44.4) | 7 (35.0) | 0.476 |
Stenosis of symptomatic qualifying artery | ||||
Stenosis, % | 78.0±6.4 | 79.2±6.5 | 76.6±6.0 | 0.129 |
Lesion length, mm | 4.2±2.2 | 4.4±2.4 | 3.9±1.9 | 0.346 |
Qualifying event | > 0.99 | |||
Ischemic stroke | 49 (75.4) | 34 (75.6) | 15 (75.0) | |
TIA | 16 (24.6) | 11 (24.4) | 5 (25.0) | |
Treatment for intracranial stenosis | > 0.99 | |||
PTA | 11 (16.9) | 8 (17.8) | 3 (15.0) | |
PTAS | 54 (83.1) | 37 (82.2) | 17 (85.5) | |
Mori type | 0.327 | |||
A | 45 (69.2) | 29 (64.4) | 16 (80.0) | |
B | 17 (26.2) | 13 (28.9) | 4 (20.0) | |
C | 3 (4.6) | 3 (6.7) | 0 (0.0) |
Values are presented as number (%), mean ± standard deviation or median [interquartile range]. NR, negative remodeling; NNR, non-negative remodeling; mRS, modified Rankin Scale; NIHSS, National Institute of Health Stroke Scale; LMCA, left middle cerebral artery; RMCA, right middle cerebral artery; TIA, transient ischemic attack; PTA, percutaneous transluminal angioplasty; PTAS, percutaneous transluminal angioplasty and stenting;
Imaging characteristics of lesions
The preoperative mean ± SD lesion stenosis was 78.0%±6.4% and 4.2±2.2 mm in length. Among the patients, 45 had Mori A lesions (69.2%), 17 had Mori B lesions (26.2%), and 3 had Mori C lesions (4.6%). On HR-MRI, the mean ± SD plaque area was 10.0±2.69 mm2, the mean ± SD plaque burden was 92.0%±5.64%, and the mean ± SD enhancement ratio was 1.3±0.3. Of the patients, 46 (70.8%) had a non-diffuse distribution (Tables 1,2). Of the patients, 35 (35/65, 53.8%) underwent MRI examination after EVT. The details of the DWI characteristics of the postoperative patients are shown in Table S1.
Table 2
Variable | Total (n=65) | NR (n=45) | NNR (n=20) | P value |
---|---|---|---|---|
Plaque area (mm2) | 10.0±2.7 | 9.2±2.5 | 11.6±2.4 | 0.001 |
Plaque burden (%) | 92.0±5.6 | 92.3±6.1 | 91.5±4.7 | 0.621 |
Enhancement ratio | 1.3±0.3 | 1.3±0.3 | 1.4±0.3 | 0.771 |
Lesion distribution (axial view) | 0.219 | |||
Ventral | 6 (9.2) | 4 (8.9) | 2 (10.0) | |
Dorsal | 2 (3.1) | 2 (4.4) | 0 (0.0) | |
Superior | 4 (6.2) | 3 (6.7) | 1 (5.0) | |
Inferior | 23 (35.4) | 12 (26.7) | 11 (55.0) | |
≥2 quadrants | 30 (46.2) | 24 (53.3) | 6 (30.0) |
Values are presented as number (%) or mean ± standard deviation. NR, negative remodeling; NNR, non-negative remodeling.
Comparison of the baseline features and lesion segment imaging characteristics between the NR and non-NR groups
There were no significant differences between the two groups in terms of gender, age, vascular risk factors, or procedure. The time intervals from the latest ischemic event to the procedure were similar between the two groups. There was no statistically significant difference between the two groups in terms of the distribution of responsible vessel locations or vascular characteristics based on DSA. Plaque burden was comparable between the two groups. Compared to the non-NR group, the NR group had a smaller plaque area (9.2 vs. 11.6 mm2, P=0.001).
Endpoints
Procedure-related PS occurred in three patients (4.6%). The symptoms included hemiparesis, weakness, and numbness on one side. The three patients developed symptoms within 24 hours of the procedure. Despite treatment, one patient deteriorated two days after the onset of symptoms (Table 3). Except for PS, hemorrhage complications occurred in two patients (3.1%), including one subarachnoid hemorrhage and one intraparenchymal hemorrhage (Tables 3,4). The probability of the primary end point was 15.0% (three patients) in the non-NR group and 0.0% (0 patients) in the NR group (P=0.026). Figures 2,3 show examples of patients in the non-NR and NR groups. The rate of any stroke or death within 30 day of enrollment was significantly higher in the non-NR group than the NR group (25.0% vs. 0.0%, P=0.002) (Table 3).
Table 3
Variable | Total (n=65) | NR (n=45) | NNR (n=20) | P value |
---|---|---|---|---|
The primary endpoint | ||||
Procedure-related PS | 3 (4.6) | 0 (0.0) | 3 (15.0) | 0.026 |
The secondary endpoint | ||||
Stroke or death within 30 days of enrollment | 5 (7.7) | 0 (0.0) | 5 (25.0) | 0.002 |
Intracranial hemorrhage | 2 (3.1) | 0 (0.0) | 2 (10.0) | 0.091 |
Values are presented as number (%); P values are the results of the Fisher’s exact test between the NR and NNR groups. NR, negative remodeling; NNR, non-negative remodeling; PS, perforator stroke.
Table 4
Patient | Remodeling pattern | Remodeling index | Plaque burden (%) | Preoperative perforator infarction (yes/no) | Time from latest ischemic event to randomization, median, d | Treatment type | Symptoms of procedure-related PS | MRI findings |
---|---|---|---|---|---|---|---|---|
1 | NNR | 1.04 | 87.6 | No | 16 | PTAS | Right upper limb weakness | Single small infarction (<1.5 cm) in the left corona radiata |
2 | NNR | 1.30 | 95.0 | Yes | 15 | PTAS | Right extremities weakness and numbness | Multiple small infarctions (<1.5 cm) in the left corona radiata |
3 | NNR | 1.08 | 98.2 | Yes | 18 | PTAS | Left-side hemiparesis | A comma-shaped infarction in the right striatocapsular area |
PS, perforator stroke; MRI, magnetic resonance imaging; NNR, non-negative remodeling; PTAS, percutaneous transluminal angioplasty and stenting.
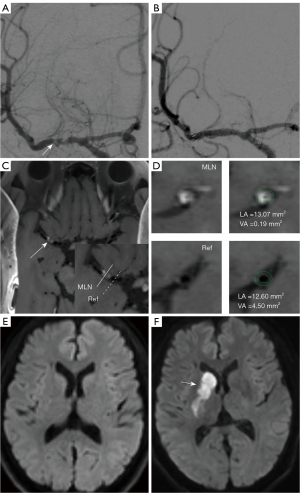
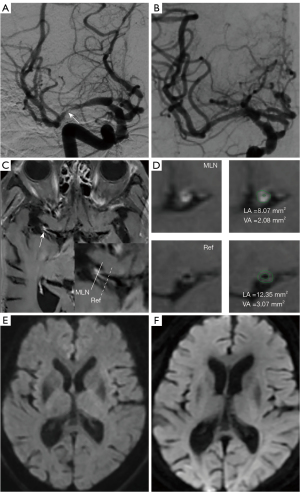
Reproducibility
Interobserver agreement was excellent for the identification of lesion distribution [Kappa: 0.95; 95% confidence interval (CI): 0.89–0.99] and remodeling patterns (Kappa: 0.93; 95% CI: 0.83–0.99). Interobserver agreement was also excellent in terms of measuring the plaque area (ICC: 0.99; 95% CI: 0.98–0.99), the enhancement ratio (ICC: 0.98; 95% CI: 0.97–0.99), and the remodeling index (ICC: 0.98; 95% CI: 0.97–0.99).
Discussion
EVT has been shown to be an effective strategy for reducing the risk of stroke recurrence in severe ICAS patients who are refractory to medical treatment. However, the effect of EVT on branch vessels, especially for MCAs, which are rich in perforator arteries, carries the risk of postoperative PS. Therefore, preoperative evaluation of the wall based on HR-MRI is of great value in patients with MCA stenosis (23,24).
In the current study, three patients (4.6%) developed PS. In the SAMMPRIS study, the incidence of PS after EVT was 6.1%. Previous studies have confirmed the relatively low incidence of procedure-related PS in MCA compared with basilar artery (4,20). Our study showed that non-NR was associated with PS. The non-NR group had more patients with PS than the NR group (15.0% vs. 0.0%, P=0.026). The non-NR group also had a larger plaque area than the NR group (11.6 vs. 9.2 mm2, P=0.001). PR plaques often coexist with large lipid cores, and there may be inflammation within the plaque. Plaques in non-NR patients are more spongy and have a higher plaque vulnerability (25). As a result, plaques in non-NR patients are more likely to rupture and migrate during surgery, which may block the perforator arteries. Conversely, the fibrotic changes associated with NR may increase internal plaque resistance to rupture (26,27), which may decrease the risk of PS after EVT. A previous study on coronary arteries showed that non-NR may be associated with increased complications after EVT (7).
Ma et al. found that NR was associated with PS after stenting for basilar artery stenosis (6). This may be due to the fact that different sites of the lesion have different effects on the perforator arteries during EVT. The paramedian pontine arteries, which are perforator arteries that arise from the basilar artery, are characterized by their comparatively narrow diameter and absence of collateral circulation (18). Plaque compression during balloon dilation and stent implantation consequently increases susceptibility of perforator arteries to occlusion, which can lead to PS. Compared to the perforator arteries from the basilar artery, the lateral lenticulostriate arteries have much larger vessel diameters, and plaque rupture and embolization at the lesion caused by EVT may be the primary cause of procedure-related PS. We also found that two of the three patients (2/3) who experienced postoperative PSs had small (<5 mm) new distal subcortical infarctions, which supports our hypothesis.
For patients with non-NR, more rigorous statins should be used to stabilize plaque as much as possible to limit the plaque damage caused by EVT and avoid injury to the perforator arteries near the lesion site. We also suggest selecting a balloon with 50% to 70% of the normal vessel’s diameter for slow expansion [1 atmosphere (atm) per 30 seconds] to prevent damage to the vessels and plaque rupture that could lead to perforator artery injuries. Notably, in our study, two patients in the non-NR group had intracranial hemorrhages during the perioperative period. This might be related to local inflammation of the vessel wall (27). There are currently limited studies in this area, and more research needs to be conducted in the future to explain this phenomenon. The advancement of imaging technology, especially the gradual clinical application of 7 Tesla MRI, will provide insights into this issue.
In this study, there were no statistically significant differences in the plaque distribution or enhancement ratio between the two groups. The plaques observed at the lesions were mainly non-diffuse, which is consistent with previous findings that plaques in the MCA tend to have an eccentric distribution (24). The marginally higher plaque burden observed in the NR group (79.2% vs. 76.6%, P=0.129) compared to the non-NR group might be attributed to the greater degree of stenosis in the NR group in the current study. Previous research has reported a positive correlation between plaque burden and the degree of stenosis at the lesion site (11). The pattern of arterial remodeling in the patients in this study was mainly NR, which is consistent with the findings of previous studies that anterior circulation more often exhibits NR (8).
Our study had some limitations. First, it was a single-center retrospective study. Due to the limited sample size and the exclusion of a portion of patients with poor-quality HR-MRI images or those who lacked HR-MRI images, bias may have been introduced. Second, because our study population only comprised patients with severe MCA stenosis, the results might not be applicable to other intracranial stenotic sites. Third, the reference segments might also have had some plaque burden and undergone remodeling, which might have led to some bias. Fourth, there may be some cases of PS that were not detected by the clinicians because of mild or transient postoperative PS symptoms.
Conclusions
This retrospective study showed that PS after MCA elective stenting appears to be related to non-NR based on HR-MRI. Further prospective and multicenter studies need to be conducted to confirm these findings.
Acknowledgments
Funding: This study was supported by
Footnote
Reporting Checklist: The authors have completed the STROBE reporting checklist. Available at https://qims.amegroups.com/article/view/10.21037/qims-24-143/rc
Conflicts of Interest: All authors have completed the ICMJE uniform disclosure form (available at https://qims.amegroups.com/article/view/10.21037/qims-24-143/coif). The authors have no conflicts of interest to declare.
Ethical Statement: The authors are accountable for all aspects of the work in ensuring that questions related to the accuracy or integrity of any part of the work are appropriately investigated and resolved. The study was conducted in accordance with the Declaration of Helsinki (as revised in 2013). The study was approved by the Ethics Board of the First Affiliated Hospital of Zhengzhou University (2022-KY-0086-001), and the requirement of individual consent for this retrospective analysis was waived.
Open Access Statement: This is an Open Access article distributed in accordance with the Creative Commons Attribution-NonCommercial-NoDerivs 4.0 International License (CC BY-NC-ND 4.0), which permits the non-commercial replication and distribution of the article with the strict proviso that no changes or edits are made and the original work is properly cited (including links to both the formal publication through the relevant DOI and the license). See: https://creativecommons.org/licenses/by-nc-nd/4.0/.
References
- Wang Y, Zhao X, Liu L, Soo YO, Pu Y, Pan Y, Wang Y, Zou X, Leung TW, Cai Y, Bai Q, Wu Y, Wang C, Pan X, Luo B, Wong KSCICAS Study Group. Prevalence and outcomes of symptomatic intracranial large artery stenoses and occlusions in China: the Chinese Intracranial Atherosclerosis (CICAS) Study. Stroke 2014;45:663-9. [Crossref] [PubMed]
- Wong LK. Global burden of intracranial atherosclerosis. Int J Stroke 2006;1:158-9. [Crossref] [PubMed]
- Jia B, Zhang X, Ma N, Mo D, Gao F, Sun X, et al. Comparison of Drug-Eluting Stent With Bare-Metal Stent in Patients With Symptomatic High-grade Intracranial Atherosclerotic Stenosis: A Randomized Clinical Trial. JAMA Neurol 2022;79:176-84. [Crossref] [PubMed]
- Fiorella D, Derdeyn CP, Lynn MJ, Barnwell SL, Hoh BL, Levy EI, et al. Detailed analysis of periprocedural strokes in patients undergoing intracranial stenting in Stenting and Aggressive Medical Management for Preventing Recurrent Stroke in Intracranial Stenosis (SAMMPRIS). Stroke 2012;43:2682-8. [Crossref] [PubMed]
- Ward MR, Pasterkamp G, Yeung AC, Borst C. Arterial remodeling. Mechanisms and clinical implications. Circulation 2000;102:1186-91. [Crossref] [PubMed]
- Ma N, Xu Z, Lyu J, Li M, Hou Z, Liu Y, Yang M, Mo D, Gao F, Song L, Sun X, Liu L, Liu X, Sui B, Shen M, Ma L, Wang Y, Wang Y, Miao Z, Lou X. Association of Perforator Stroke After Basilar Artery Stenting With Negative Remodeling. Stroke 2019;50:745-9. [Crossref] [PubMed]
- Qiao Y, Anwar Z, Intrapiromkul J, Liu L, Zeiler SR, Leigh R, Zhang Y, Guallar E, Wasserman BA. Patterns and Implications of Intracranial Arterial Remodeling in Stroke Patients. Stroke 2016;47:434-40. [Crossref] [PubMed]
- White AJ, Duffy SJ, Walton AS, Ng JF, Rice GE, Mukherjee S, Shaw JA, Jennings GL, Dart AM, Kingwell BA. Matrix metalloproteinase-3 and coronary remodelling: implications for unstable coronary disease. Cardiovasc Res 2007;75:813-20. [Crossref] [PubMed]
- Wexberg P, Gyöngyösi M, Sperker W, Kiss K, Yang P, Hassan A, Pasterkamp G, Glogar D. Pre-existing arterial remodeling is associated with in-hospital and late adverse cardiac events after coronary interventions in patients with stable angina pectoris. J Am Coll Cardiol 2000;36:1860-9. [Crossref] [PubMed]
- Kim JM, Jung KH, Sohn CH, Moon J, Shin JH, Park J, Lee SH, Han MH, Roh JK. Intracranial plaque enhancement from high resolution vessel wall magnetic resonance imaging predicts stroke recurrence. Int J Stroke 2016;11:171-9. [Crossref] [PubMed]
- Ran Y, Wang Y, Zhu M, Wu X, Malhotra A, Lei X, Zhang F, Wang X, Xie S, Zhou J, Zhu J, Cheng J, Zhu C. Higher Plaque Burden of Middle Cerebral Artery Is Associated With Recurrent Ischemic Stroke: A Quantitative Magnetic Resonance Imaging Study. Stroke 2020;51:659-62. [Crossref] [PubMed]
- Mandell DM, Mossa-Basha M, Qiao Y, Hess CP, Hui F, Matouk C, Johnson MH, Daemen MJ, Vossough A, Edjlali M, Saloner D, Ansari SA, Wasserman BA, Mikulis DJVessel Wall Imaging Study Group of the American Society of Neuroradiology. Intracranial Vessel Wall MRI: Principles and Expert Consensus Recommendations of the American Society of Neuroradiology. AJNR Am J Neuroradiol 2017;38:218-29. [Crossref] [PubMed]
- Brott T, Adams HP Jr, Olinger CP, Marler JR, Barsan WG, Biller J, Spilker J, Holleran R, Eberle R, Hertzberg V. Measurements of acute cerebral infarction: a clinical examination scale. Stroke 1989;20:864-70. [Crossref] [PubMed]
- Broderick JP, Adeoye O, Elm J. Evolution of the Modified Rankin Scale and Its Use in Future Stroke Trials. Stroke 2017;48:2007-12. [Crossref] [PubMed]
- Samuels OB, Joseph GJ, Lynn MJ, Smith HA, Chimowitz MI. A standardized method for measuring intracranial arterial stenosis. AJNR Am J Neuroradiol 2000;21:643-6.
- Ma N, Jiang WJ, Lou X, Ma L, Du B, Cai JF, Zhao TQ. Arterial remodeling of advanced basilar atherosclerosis: a 3-tesla MRI study. Neurology 2010;75:253-8. [Crossref] [PubMed]
- Xu WH, Li ML, Gao S, Ni J, Zhou LX, Yao M, Peng B, Feng F, Jin ZY, Cui LY. In vivo high-resolution MR imaging of symptomatic and asymptomatic middle cerebral artery atherosclerotic stenosis. Atherosclerosis 2010;212:507-11. [Crossref] [PubMed]
- Vogels V, Dammers R, van Bilsen M, Volovici V. Deep Cerebral Perforators: Anatomical Distribution and Clinical Symptoms: An Overview. Stroke 2021;52:e660-74. [Crossref] [PubMed]
- Mori T, Fukuoka M, Kazita K, Mori K. Follow-up study after intracranial percutaneous transluminal cerebral balloon angioplasty. AJNR Am J Neuroradiol 1998;19:1525-33.
- Jiang WJ, Srivastava T, Gao F, Du B, Dong KH, Xu XT. Perforator stroke after elective stenting of symptomatic intracranial stenosis. Neurology 2006;66:1868-72. [Crossref] [PubMed]
- Koo TK, Li MY. A Guideline of Selecting and Reporting Intraclass Correlation Coefficients for Reliability Research. J Chiropr Med 2016;15:155-63. [Crossref] [PubMed]
- McGinn T, Wyer PC, Newman TB, Keitz S, Leipzig R, For GGEvidence-Based Medicine Teaching Tips Working Group. Tips for learners of evidence-based medicine: 3. Measures of observer variability (kappa statistic). CMAJ 2004;171:1369-73. [Crossref] [PubMed]
- Bai X, Fu M, Li Z, Gao P, Zhao H, Li R, Sui B. Distribution and regional variation of wall shear stress in the curved middle cerebral artery using four-dimensional flow magnetic resonance imaging. Quant Imaging Med Surg 2022;12:5462-73. [Crossref] [PubMed]
- Xu WH, Li ML, Gao S, Ni J, Zhou LX, Yao M, Peng B, Feng F, Jin ZY, Cui LY. Plaque distribution of stenotic middle cerebral artery and its clinical relevance. Stroke 2011;42:2957-9. [Crossref] [PubMed]
- Varnava AM, Mills PG, Davies MJ. Relationship between coronary artery remodeling and plaque vulnerability. Circulation 2002;105:939-43. [Crossref] [PubMed]
- Falk E, Shah PK, Fuster V. Coronary plaque disruption. Circulation 1995;92:657-71. [Crossref] [PubMed]
- Schoenhagen P, Ziada KM, Kapadia SR, Crowe TD, Nissen SE, Tuzcu EM. Extent and direction of arterial remodeling in stable versus unstable coronary syndromes : an intravascular ultrasound study. Circulation 2000;101:598-603. [Crossref] [PubMed]