Natural course of apparent diffusion coefficient (ADC) change after brain ischemic stroke: an alternative explanation by the triphasic relationship between T2 and ADC
Recently, we proposed that in vivo apparent diffusion coefficient (ADC) measure is strongly associated with T2 relaxation time (T2) (1-5). In this context, T2 can be divided into short T2 band (<60 ms), intermediate T2 band (60–80 ms), and long T2 band (>80 ms, all 3T values). For the short T2 time band, there is a negative correlation between T2 and ADC. For the long T2 time band, there is a positive correlation between T2 and ADC (Figure 1, Figure S1). A tissue likely measures a low ADC if its T2 is close to 70 ms. On the other hand, a tissue is likely to measure a high ADC if its T2 is far away from 70 ms.
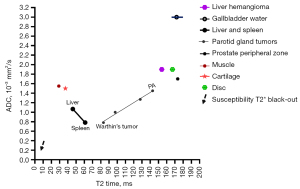
This ‘T2-ADC curve’ shown in Figure 1 can help explain the counterintuitive ADC values commonly seen in several tissues. The liver and the spleen are almost equally perfused. However, the spleen has a much lower ADC value than the liver (spleen: 0.8×10−3 mm2, liver: 1.1×10−3 mm2), we consider this is due to that the spleen has a longer T2 than the liver (spleen: 60 ms, liver 42: ms, 3T values). Experimental studies have shown that the hepatic blood volume including that of the large vessels is about 25 mL/100 g, whereas this value is 3 mL/100 g in skeletal muscle (6). Thus, it could be thought that the ADC of muscles is not higher than that of liver with the liver more richly perfused by hepatic artery and portal vein and with lots of sinusoids and space of Disse. However, muscle has a shorter T2 time than the liver. In the study of Wall et al. (7), muscle measured a T2 of 29 ms whereas liver measured a T2 of 45 ms at 0.35T. In the study of de Bazelaire et al. (8), muscle measured a T2 of 29 ms whereas liver measured a T2 of 46 ms at 1.5T. Figure 1 shows, with liver data as the reference, the shorter T2 of muscle is associated with an increased ADC value for the muscle (relative to the liver). Figure 1 also helps to explain why cartilage and intervertebral disc nucleus pulposus and inner annulus fibrosus measure very high ADC. This is not because these tissues have true high tissue diffusivity, but instead because of their T2 being both away from the intermediate T2 of 60–80 ms (at 3T). Moreover, cartilage and intervertebral disc nucleus pulposus and inner annulus fibrosus demonstrate high ADC due to the opposite reasons, with cartilage having a relatively short T2 and non-degenerated disc having a long T2. Pyogenic abscess fluid (i.e., pus) tends to demonstrate a very low ADC (e.g., 0.63×10−3 mm2/s) regardless of the location of the abscess (9-12). We noted that (4), abscess pus having a T2 of around 80 ms contributes to its very low ADC measured by MRI. Lymphoma has a T2 close to 60–70 ms at 3T. For example, Dooms et al. (13) reported a lymphoma mean T2 of 58 ms at 0.35T; Weissleder et al. (14) reported a lymphoma mean T2 of 84 ms and a liver mean T2 of 51 ms at 0.6T (thus, lymphoma will have a mean T2 of around 74 ms at 3T). Consistent with the ‘T2-ADC curve’, lymphoma shows low ADC (15-17). Wu et al. (17) further reported that there was no correlation between the ADC value and cellularity of the tumor in patients with diffuse large B-cell lymphoma and follicular lymphoma. Note that a lack of correlation between ADC and cell density is a common finding in various pathologies (16-21). Morán et al. (22) and Einarsdóttir et al. (23) reported myxoma ADC values of 2.38×10−3 and 2.80×10−3 mm2/s respectively, which are quite high. Myxoid substance has a long T2, and myxoma has a high ADC likely due to myxoid substance’s long T2. Another disease is chondrosarcoma. Chondrosarcoma has a long T2 (e.g., 120 ms) and high ADC measure [e.g., 2.3×10−3 mm2 (24)]. It is unlikely that chondrosarcoma has a true high tissue diffusivity. For parotid gland tumors, Warthin’s tumor [T2: 83.3 ms, 3T data of Baohong et al. (25)] is associated with low ADC and pleomorphic adenoma is associated with high ADC [T2: 142.9 ms, 3T data of Baohong et al. (25)]. For pleomorphic adenoma, long T2 (and thus high ADC measure) can be attributed to its myxomatous and chondroid contents (26). For Warthin’s tumor, low ADC can be attributed to its mature lymphocytic components (27,28). Spleen, which also has T2 of around 60 ms at 3T and is associated with low ADC, is also part of the lymphatic system.
For prostate cancer, an injection of gadolinium contrast agent, which shortens T2 of the cancerous tissues and shifts the T2 time of cancerous tissues toward 70 ms, has been noted to be associated with a lower ADC measure without the gadolinium agent actually changing the diffusivity of the tissues (29). For the purpose of evaluating in vivo perfusion, we have been using the subtraction of signal intensity on non-zero low b value images from signal intensity on b=0 image (30-35). Diffusion-derived ‘vessel density’ (DDVD), initially proposed as an imaging biomarker to evaluate liver perfusion (30), is calculated from a simple diffusion MRI protocol as
where Sb0 refers to the measured liver sum signal intensity when b=0 s/mm2, and Sb2 refers to the measured liver sum signal intensity when b=2 s/mm2. ROIarea0 and ROIarea2 refer to the number of pixels in the selected region-of-interest (ROI) on b=0 and 2 s/mm2 images, respectively. Sb2 and ROIarea2 can also be approximated by other low b value diffusion image data (33-35). In a recent study (36), when DDVD was calculated from b=0 and 1 (or 2) s/mm2 images, then following the principle that liver and spleen have similar amounts of perfusion, there is little difference between liver DDVD and spleen DDVD. Simple liver cyst shows very low DDVD reflecting there is no perfusion inside the cyst. However, when DDVD was calculated from b=0 and 20 s/mm2 images, then, with liver value as the reference, spleen DDVD was ‘suppressed’ due to the spleen T2 being around 60 ms. Since cyst has a long T2 (maybe similar to gallbladder being around 160 ms), liver cyst DDVD calculated from b=0 and 20 s/mm2 became higher than liver DDVD (Figure 2). These results further support that T2 of the tissues/fluid always heavily contributes to diffusion metrics computed with ≥ two b values and when these b values are not very close to each other (3,4).
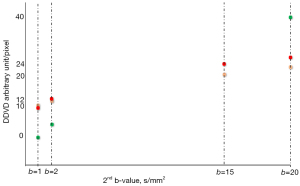
Note that, in addition to T2 (which is often dominant), we consider diffusion indeed contributes to ADC (3). This is also evidenced by the success of the diffusion tensor imaging technique. When a cluster of voxels has a homogeneous T2 value, then their dominant diffusion direction can still be measured.
Brain acute ischemic stroke is a major cause of death and a leading cause of disability globally. Neuroimaging is a cornerstone in the diagnosis and treatment decision-making. It has been well documented that, once a stroke occurs, stroke lesion’s ADC starts to decrease and T2 starts to increase (37-46). In human studies, T2 typically follows a pattern of stepwise gradual increase till plateau (39,44). On the other hand, ADC follows a course of (I) decrease, (II) pseudo-normalization, (III) elevation [note that experimental results based on rodents are different from those of humans, the current analyses are based only on human data (45)]. The initial ADC decrease has been proposed to be due to cytotoxic oedema, which results in cellular swelling and a reduction in the extracellular volume and increased restricted diffusion on MRI. However, the evidence to support this theory of restricted diffusion remains limited.
Using our ‘T2-ADC curve’ (3), we attempt to offer an alternative explanation for the ADC evolution course of stroke lesions. Normal brain tissues are generally noted to have a short T2 with grey/dark signal on T2 weighted image. Wansapura et al. described that, at 3T the average T2 values for occipital and frontal gray matter are 41.6 and 51.8 ms, respectively, and average T2 values for occipital and frontal white matter are 48.4 and 44.7 ms, respectively (47-50). When an abscess develops inside the brain and abscess pus is roughly estimated to have a T2 of around 80 ms (4), according to Figures 1,3 (4,50), an increase of T2 time from grey/white matter values toward 80 ms (at 3T) would be associated with a lower ADC. The same is true for the development of brain lymphoma (13,14,16). Following the examples of brain abscess and lymphoma, an initial increase of T2 of a stroke lesion will lead to a decrease of ADC measure. With the further increase of the lesion’s T2 and when the T2 reaches the value of around 90 ms at 3T or 110 ms at 1.5T (approximately estimated from Figures 1,3), the lesion’s ADC will pseudo-normalize. After that, the stroke lesion’s T2 becomes even longer, and lesion’s ADC will start to show elevated compared to the contra-lateral brain tissue.
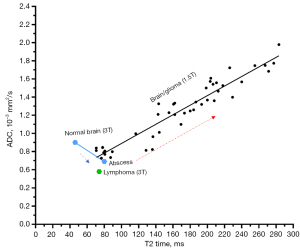
We try to tentatively answer the questions: do we have evidence to show the timepoint of ADC lesion pseudo-normalization is the timepoint when T2 reaches around 90 ms at 3T or around 110 ms at 1.5T? Since it has been shown that pseudo-normalization of stroke lesion’s ADC occurs ‘somewhat’ 10 days after the stroke incident (37-39,42), is stroke lesion’s T2 around 90 ms at 3T or 110 ms at 1.5T 10 days after the stroke incident? According to the report of Lutsep et al. (1.5T data) (39,40), about 5 months after infarction incidence, lesion’s T2-weighted image signal intensity is about ×2 of contra-lateral brain tissue (and appears to be plateaued). Figure 4 shows, if normal brain tissue signal intensity is increased ×2, then the signal intensity will be overall close to the signal intensity of most of the gliomas. Noted that, according to the report of Oh et al. (50), at 1.5T, brain white matter had a median T2 value of 77 ms, and glioma had a median T2 value of 160 ms. According to Lutsep et al. (39) and Lansberg et al. (44), about 10 days after an infarction incidence, lesion’s T2-weighted image signal intensity is about ×1.7 of contra-lateral brain tissue. If normal brain tissue signal intensity is increased ×1.7, then the signal intensity will be lower than the signal intensity of most of glioma tissues shown in Figure 5. It is difficult to estimate how the normal brain tissue corresponds to a ‘new T2 value’ if normal brain tissue signal intensity is elevated by ×1.7, but it is possible that the ‘new T2’ might be around 90–100 ms at 3T and 110–120 ms at 1.5T (roughly approximated based on Figures 3,5).
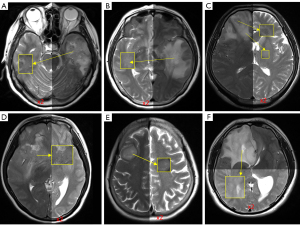
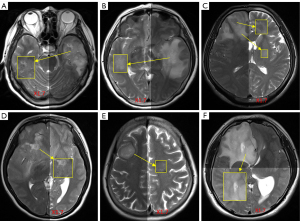
In conclusion, for the brain ischemic stroke lesion ADC triphasic evolution course of decrease, pseudo-normalization, and elevation, we propose that this can be explained by the triphasic relationship between T2 and ADC. This model may support the differential diagnosis of various brain abnormalities.
Acknowledgments
The author would like to thank Mr. Ben-Heng Xiao, Research student at the Chinese University of Hong Kong, for the processing of Figures 4,5, and Dr. Ming-Hua Sun, Visting radiologist at the Chinese University of Hong Kong, for providing the raw images used in Figures 4,5.
Funding: This work was supported by
Footnote
Conflicts of Interest: The author has completed the ICMJE uniform disclosure form (available at https://qims.amegroups.com/article/view/10.21037/qims-24-2383/coif). Y.X.J.W. serves as the Editor-in-Chief of Quantitative Imaging in Medicine and Surgery. The author has no other conflicts of interest to declare.
Ethical Statement: The author is accountable for all aspects of the work in ensuring that questions related to the accuracy or integrity of any part of the work are appropriately investigated and resolved.
Open Access Statement: This is an Open Access article distributed in accordance with the Creative Commons Attribution-NonCommercial-NoDerivs 4.0 International License (CC BY-NC-ND 4.0), which permits the non-commercial replication and distribution of the article with the strict proviso that no changes or edits are made and the original work is properly cited (including links to both the formal publication through the relevant DOI and the license). See: https://creativecommons.org/licenses/by-nc-nd/4.0/.
References
- Yu WL, Xiao BH, Ma FZ, Zheng CJ, Tang SN, Wáng YXJ. Underestimation of the spleen perfusion fraction by intravoxel incoherent motion MRI. NMR Biomed 2023;36:e4987. [Crossref] [PubMed]
- Wáng YXJ, Zhao KX, Ma FZ, Xiao BH. The contribution of T2 relaxation time to MRI-derived apparent diffusion coefficient (ADC) quantification and its potential clinical implications. Quant Imaging Med Surg 2023;13:7410-6. [Crossref] [PubMed]
- Wáng YXJ, Ma FZ. A tri-phasic relationship between T2 relaxation time and magnetic resonance imaging (MRI)-derived apparent diffusion coefficient (ADC). Quant Imaging Med Surg 2023;13:8873-80. [Crossref] [PubMed]
- Wáng YXJ. The very low magnetic resonance imaging apparent diffusion coefficient (ADC) measure of abscess is likely due to pus's specific T2 relaxation time. Quant Imaging Med Surg 2023;13:8881-5. [Crossref] [PubMed]
- Wáng YXJ, Aparisi Gómez MP, Ruiz Santiago F, Bazzocchi A. The relevance of T2 relaxation time in interpreting MRI apparent diffusion coefficient (ADC) map for musculoskeletal structures. Quant Imaging Med Surg 2023;13:7657-66. [Crossref] [PubMed]
- Greenway CV, Stark RD. Hepatic vascular bed. Physiol Rev 1971;51:23-65. [Crossref] [PubMed]
- Wall SD, Fisher MR, Amparo EG, Hricak H, Higgins CB. Magnetic resonance imaging in the evaluation of abscesses. AJR Am J Roentgenol 1985;144:1217-21. [Crossref] [PubMed]
- de Bazelaire CM, Duhamel GD, Rofsky NM, Alsop DC. MR imaging relaxation times of abdominal and pelvic tissues measured in vivo at 3.0 T: preliminary results. Radiology 2004;230:652-9. [Crossref] [PubMed]
- Feraco P, Donner D, Gagliardo C, Leonardi I, Piccinini S, Del Poggio A, Franciosi R, Petralia B, van den Hauwe L. Cerebral abscesses imaging: A practical approach. J Popul Ther Clin Pharmacol 2020;27:e11-24. [Crossref] [PubMed]
- Harish S, Chiavaras MM, Kotnis N, Rebello R. MR imaging of skeletal soft tissue infection: utility of diffusion-weighted imaging in detecting abscess formation. Skeletal Radiol 2011;40:285-94. [Crossref] [PubMed]
- Subhawong TK, Durand DJ, Thawait GK, Jacobs MA, Fayad LM. Characterization of soft tissue masses: can quantitative diffusion weighted imaging reliably distinguish cysts from solid masses? Skeletal Radiol 2013;42:1583-92. [Crossref] [PubMed]
- Erdogan C, Hakyemez B, Yildirim N, Parlak M. Brain abscess and cystic brain tumor: discrimination with dynamic susceptibility contrast perfusion-weighted MRI. J Comput Assist Tomogr 2005;29:663-7. [Crossref] [PubMed]
- Dooms GC, Hricak H, Moseley ME, Bottles K, Fisher M, Higgins CB. Characterization of lymphadenopathy by magnetic resonance relaxation times: preliminary results. Radiology 1985;155:691-7. [Crossref] [PubMed]
- Weissleder R, Stark DD, Elizondo G, Hahn PF, Compton C, Saini S, Wittenberg J, Ferrucci JT. MRI of hepatic lymphoma. Magn Reson Imaging 1988;6:675-81. [Crossref] [PubMed]
- Haldorsen IS, Espeland A, Larsson EM. Central nervous system lymphoma: characteristic findings on traditional and advanced imaging. AJNR Am J Neuroradiol 2011;32:984-92. [Crossref] [PubMed]
- Stadnik TW, Chaskis C, Michotte A, Shabana WM, van Rompaey K, Luypaert R, Budinsky L, Jellus V, Osteaux M. Diffusion-weighted MR imaging of intracerebral masses: comparison with conventional MR imaging and histologic findings. AJNR Am J Neuroradiol 2001;22:969-76.
- Wu X, Pertovaara H, Dastidar P, Vornanen M, Paavolainen L, Marjomäki V, Järvenpää R, Eskola H, Kellokumpu-Lehtinen PL. ADC measurements in diffuse large B-cell lymphoma and follicular lymphoma: a DWI and cellularity study. Eur J Radiol 2013;82:e158-64. [Crossref] [PubMed]
- Sadeghi N, D'Haene N, Decaestecker C, Levivier M, Metens T, Maris C, Wikler D, Baleriaux D, Salmon I, Goldman S. Apparent diffusion coefficient and cerebral blood volume in brain gliomas: relation to tumor cell density and tumor microvessel density based on stereotactic biopsies. AJNR Am J Neuroradiol 2008;29:476-82. [Crossref] [PubMed]
- Rosenkrantz AB, Matza BW, Sabach A, Hajdu CH, Hindman N. Pancreatic cancer: lack of association between apparent diffusion coefficient values and adverse pathological features. Clin Radiol 2013;68:e191-7. [Crossref] [PubMed]
- Nonomura Y, Yasumoto M, Yoshimura R, Haraguchi K, Ito S, Akashi T, Ohashi I. Relationship between bone marrow cellularity and apparent diffusion coefficient. J Magn Reson Imaging 2001;13:757-60. [Crossref] [PubMed]
- Rygh CB, Wang J, Thuen M, Gras Navarro A, Huuse EM, Thorsen F, Poli A, Zimmer J, Haraldseth O, Lie SA, Enger PØ, Chekenya M. Dynamic contrast enhanced MRI detects early response to adoptive NK cellular immunotherapy targeting the NG2 proteoglycan in a rat model of glioblastoma. PLoS One 2014;9:e108414. [Crossref] [PubMed]
- Morán LM, Vega J, Gómez-León N, Royuela A. Myxomas and myxoid liposarcomas of the extremities: Our preliminary findings in conventional, perfusion, and diffusion magnetic resonance. Acta Radiol Open 2022;11:20584601221131481. [Crossref] [PubMed]
- Einarsdóttir H, Karlsson M, Wejde J, Bauer HC. Diffusion-weighted MRI of soft tissue tumours. Eur Radiol 2004;14:959-63. [Crossref] [PubMed]
- Subhawong TK, Jacobs MA, Fayad LM. Insights into quantitative diffusion-weighted MRI for musculoskeletal tumor imaging. AJR Am J Roentgenol 2014;203:560-72. [Crossref] [PubMed]
- Baohong W, Jing Z, Zanxia Z, Kun F, Liang L, Eryuan G, Yong Z, Fei H, Jingliang C, Jinxia Z. T2 mapping and readout segmentation of long variable echo-train diffusion weighted imaging for the differentiation of parotid gland tumors. Eur J Radiol 2022;151:110265. [Crossref] [PubMed]
- Matsumiya-Matsumoto Y, Morita Y, Uzawa N. Pleomorphic Adenoma of the Salivary Glands and Epithelial-Mesenchymal Transition. J Clin Med 2022;11:4210. [Crossref] [PubMed]
- Teymoortash A, Schrader C, Shimoda H, Kato S, Werner JA. Evidence of lymphangiogenesis in Warthin's tumor of the parotid gland. Oral Oncol 2007;43:614-8. [Crossref] [PubMed]
- Sučić M, Ljubić N, Perković L, Ivanović D, Pažanin L, Sučić Radovanović T, Župnić-Krmek D, Knežević F. Cytopathology and diagnostics of Warthin's tumour. Cytopathology 2020;31:193-207. [Crossref] [PubMed]
- Mazaheri Y, Hötker AM, Shukla-Dave A, Akin O, Hricak H. Effect of intravascular contrast agent on diffusion and perfusion fraction coefficients in the peripheral zone and prostate cancer. Magn Reson Imaging 2018;51:120-7. [Crossref] [PubMed]
- Wáng YXJ. Living tissue intravoxel incoherent motion (IVIM) diffusion MR analysis without b=0 image: an example for liver fibrosis evaluation. Quant Imaging Med Surg 2019;9:127-33. [Crossref] [PubMed]
- Xiao BH, Huang H, Wang LF, Qiu SW, Guo SW, Wáng YXJ. Diffusion MRI Derived per Area Vessel Density as a Surrogate Biomarker for Detecting Viral Hepatitis B-Induced Liver Fibrosis: A Proof-of-Concept Study. SLAS Technol 2020;25:474-83. [Crossref] [PubMed]
- Huang H, Zheng CJ, Wang LF, Che-Nordin N, Wáng YXJ. Age and gender dependence of liver diffusion parameters and the possibility that intravoxel incoherent motion modeling of the perfusion component is constrained by the diffusion component. NMR Biomed 2021;34:e4449. [Crossref] [PubMed]
- Hu GW, Li CY, Zhang G, Zheng CJ, Ma FZ, Quan XY, Chen W, Sabarudin A, Zhu MSY, Li XM, Wáng YXJ. Diagnosis of liver hemangioma using magnetic resonance diffusion-derived vessel density (DDVD) pixelwise map: a preliminary descriptive study. Quant Imaging Med Surg 2024; [Crossref]
- Chen JQ, Li CY, Wang W, Yao DQ, Jiang RF, Wáng YXJ. Diffusion-derived vessel density (DDVD) for penumbra delineation in acute ischemic stroke: initial proof-of-concept results using single NEX DWI. Quant Imaging Med Surg 2024; [Crossref]
- Li XM, Yao DQ, Quan XY, Li M, Chen W, Wáng YXJ. Perfusion of hepatocellular carcinomas measured by diffusion-derived vessel density biomarker: Higher hepatocellular carcinoma perfusion than earlier intravoxel incoherent motion reports. NMR Biomed 2024;37:e5125. [Crossref] [PubMed]
- Ju ZG, Leng XM, Xiao BX, Sun MH, Huang H, Hu GW, Zhang G, Sun JH, Zhu MSY, Guglielmi G, Wáng YXJ. Influences of the second motion probing gradient b-value and T2 relaxation time on magnetic resonance diffusion derived ‘vessel density’ (DDVD) calculation: the examples of liver, spleen, and liver simple cyst. Quant Imaging Med Surg 2024; [Crossref]
- Marks MP, de Crespigny A, Lentz D, Enzmann DR, Albers GW, Moseley ME. Acute and chronic stroke: navigated spin-echo diffusion-weighted MR imaging. Radiology 1996;199:403-8. [Crossref] [PubMed]
- Schlaug G, Siewert B, Benfield A, Edelman RR, Warach S. Time course of the apparent diffusion coefficient (ADC) abnormality in human stroke. Neurology 1997;49:113-9. [Crossref] [PubMed]
- Lutsep HL, Albers GW, DeCrespigny A, Kamat GN, Marks MP, Moseley ME. Clinical utility of diffusion-weighted magnetic resonance imaging in the assessment of ischemic stroke. Ann Neurol 1997;41:574-80. [Crossref] [PubMed]
- de Crespigny AJ, Marks MP, Enzmann DR, Moseley ME. Navigated diffusion imaging of normal and ischemic human brain. Magn Reson Med 1995;33:720-8. [Crossref] [PubMed]
- Knight RA, Dereski MO, Helpern JA, Ordidge RJ, Chopp M. Magnetic resonance imaging assessment of evolving focal cerebral ischemia. Comparison with histopathology in rats. Stroke 1994;25:1252-61; discussion 1261-2. [Crossref] [PubMed]
- Li TQ, Chen ZG, Hindmarsh T. Diffusion-weighted MR imaging of acute cerebral ischemia. Acta Radiol 1998;39:460-73. [Crossref] [PubMed]
- Calamante F, Lythgoe MF, Pell GS, Thomas DL, King MD, Busza AL, Sotak CH, Williams SR, Ordidge RJ, Gadian DG. Early changes in water diffusion, perfusion, T1, and T2 during focal cerebral ischemia in the rat studied at 8.5 T. Magn Reson Med 1999;41:479-85. [Crossref] [PubMed]
- Lansberg MG, Thijs VN, O'Brien MW, Ali JO, de Crespigny AJ, Tong DC, Moseley ME, Albers GW. Evolution of apparent diffusion coefficient, diffusion-weighted, and T2-weighted signal intensity of acute stroke. AJNR Am J Neuroradiol 2001;22:637-44.
- Liu Y, D'Arceuil HE, Westmoreland S, He J, Duggan M, Gonzalez RG, Pryor J, de Crespigny AJ. Serial diffusion tensor MRI after transient and permanent cerebral ischemia in nonhuman primates. Stroke 2007;38:138-45. [Crossref] [PubMed]
- McGarry BL, Rogers HJ, Knight MJ, Jokivarsi KT, Sierra A, Gröhn OH, Kauppinen RA. Stroke onset time estimation from multispectral quantitative magnetic resonance imaging in a rat model of focal permanent cerebral ischemia. Int J Stroke 2016;11:677-82. [Crossref] [PubMed]
- Wansapura JP, Holland SK, Dunn RS, Ball WS Jr. NMR relaxation times in the human brain at 3.0 tesla. J Magn Reson Imaging 1999;9:531-8. [Crossref] [PubMed]
- Jezzard P, Duewell S, Balaban RS. MR relaxation times in human brain: measurement at 4 T. Radiology 1996;199:773-9. [Crossref] [PubMed]
- Krauss W, Gunnarsson M, Andersson T, Thunberg P. Accuracy and reproducibility of a quantitative magnetic resonance imaging method for concurrent measurements of tissue relaxation times and proton density. Magn Reson Imaging 2015;33:584-91. [Crossref] [PubMed]
- Oh J, Cha S, Aiken AH, Han ET, Crane JC, Stainsby JA, Wright GA, Dillon WP, Nelson SJ. Quantitative apparent diffusion coefficients and T2 relaxation times in characterizing contrast enhancing brain tumors and regions of peritumoral edema. J Magn Reson Imaging 2005;21:701-8. [Crossref] [PubMed]