Prognostic value of left atrial strain in patients with stage II light-chain cardiac amyloidosis receiving cardiac magnetic resonance imaging: a prospective cohort study
Introduction
Light-chain amyloidosis (AL) is a life-threatening condition caused by clonal plasma cell dyscrasia that results in the progressive deposition of misfolded immunoglobulin light chains in the extracellular space. Cardiac involvement is the primary determinant of outcome in patients AL and influences the related therapeutic choices (1). The severity of cardiac AL (AL-CA) is evaluated using staging systems based on cardiac biomarkers including N-terminal pro-B-type natriuretic peptide (NT-proBNP) and cardiac troponin I or T (2). The Mayo 2004 staging system identifies stage II patients with varying risk factors, including elevated NT-proBNP or troponin levels (2). These patients are considered to have an average prognosis. However, the outcome of certain patients remains suboptimal in clinical practice despite the introduction of novel and effective treatments. Most studies on this subject have focused on the AL-CA cohort as a whole (3,4), yet to specifically address stage II patients, a subgroup analysis is needed.
Cardiac magnetic resonance (CMR) parameters, such as extracellular volume (ECV) and left ventricular (LV) ejection fraction (EF), offer prognostic information on patients with AL-CA (5). Furthermore, myocardial strain is a better indicator of cardiac function than are volume measurements, with LV strain being a strong independent predictor of mortality (6). Recently, the left atrium (LA) as an indicator of LV diastolic function has attracted interest in cardiomyopathy research. LA function can be divided into three components: reservoir function for collecting blood from the pulmonary veins during ventricular systole, conduit function for transferring blood to the LV during early LV diastole and diastasis, and booster function for enhancing ventricular filling via LA contraction during late diastole (7). Assessment of LA strain, representing LA function, has been proven to provide incremental prognosis of clinical outcomes in patients with myocardial infarction and cardiomyopathy (8-11). In a recent retrospective study of patients with cardiac amyloidosis, LA volume and booster strain were significantly associated with the outcome (12). However, the prognostic value of LA strain in patients with AL-CA alone and when combined with the known predictors in these patients remains uncertain.
In this study, we prospectively examined 131 patients diagnosed with stage II AL-CA and sought to identify the factors capable of further stratifying patient outcomes. Additionally, we evaluated the prognostic value of adding LA strain to established clinical risk markers. We present this article in accordance with the STROBE reporting checklist (available at https://qims.amegroups.com/article/view/10.21037/qims-24-1025/rc).
Methods
Study population
Patients with AL, who were referred for CMR imaging at Peking Union Medical College Hospital between January 1, 2015 and October 31, 2021, were consecutively enrolled in the study. Patients with contraindications either to CMR imaging or contrast administration were excluded. All patients had biopsy evidence of AL amyloidosis with positive Congo red staining and light-chain deposition confirmed by immunohistochemistry, immunofluorescence, or mass spectrometry. The assays were performed in tissues of the kidney (n=59), myocardium (n=37), fat (n=9), bone marrow (n=7), tongue (n=7), liver (n=4), upper gastrointestinal tract (n=4), buccal mucosa (n=3), and skin (n=1). Subsequently, cardiac involvement was diagnosed according to the following criteria: (I) endomyocardial biopsy-proven cardiac amyloidosis; and (II) extracardiac biopsy-proven amyloidosis, with NT-proBNP >332 pg/mL and LV mean wall thickness >12 mm in the absence of hypertension or other potential causes of LV hypertrophy (2). Mayo stage was determined based on the Mayo 2004 staging system, which included assessment of NT-proBNP and cardiac troponin I for survival prognosis (2). Stage II disease was defined as NT-proBNP >332 pg/mL or cardiac troponin I >0.1 mg/L, and patients with stage II disease were selected for study inclusion. All patients received standard bortezomib- or melphalan-based chemotherapy directed at an abnormal plasma cell clone. Figure 1 outlines the overall inclusion and exclusion criteria. The final analysis included 131 patients with a median follow-up of 28 (IQR, 10–50) months. This prospective study adhered to the Declaration of Helsinki (as revised in 2013) and was approved by the Institutional Ethics Committee for Human Research at Peking Union Medical College Hospital (Beijing, China) [institutional review board (IRB) protocol No. JS1470]. All participants provided written informed consent before enrollment.
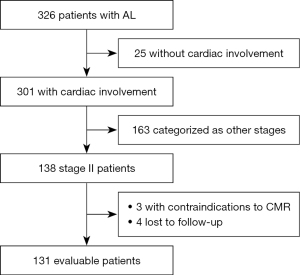
Follow-up
A physician blinded to the results of CMR imaging conducted monthly telephone and clinical follow-ups. All-cause mortality was the primary endpoint while the combination of all-cause mortality and cardiac progression was defined as the secondary endpoint. Cardiac progression was defined as NT-proBNP progression (430% and 4,300 ng/L increase) or cardiac troponin progression (≥33% increase) or EF progression (≥10% decrease) (13). Patients were censored at the end of the study unless they reached the endpoints. For cases in which patients were lost to follow-up, the data from the last visit to clinic were used.
CMR scanning protocol
CMR imaging was conducted using 3-T whole-body scanners (MAGNETOM Skyra, Siemens Healthineers, Erlangen, Germany) with an 18-element body matrix coil and a 32-element spine array coil for image acquisition. Electrocardiography gating and breath-holding were employed.
Cine images were acquired using an electrocardiography-gated balanced steady-state free precession sequence (bSSFP) in two-, three-, and four-chamber long-axis view and in short-axis views (repetition time/echo time, 3.3 ms/1.43 ms; flip angle, 55–70°; voxel size, 1.6×1.6×6.0 mm3; temporal resolution, 45.6 ms; bandwidth, 962 Hz/pixel). Native and 15- to 20-minute postcontrast T1 maps were acquired using a Modified Look-Lockers Inversion-recovery sequence in identical imaging locations, including a four-chamber long-axis view and basal-, mid-, and apical–ventricular short-axis views (repetition time/echo time/2.7 ms/1.12 ms; flip angle, 20°; voxel size, 1.4×1.4×8.0 mm3). Acquisition schemas 5(3)3 and 4(1)3(1)2 were used for precontrast and postcontrast T1 mapping, respectively. T2 mapping was acquired using a T2-prepared single-shot bSSFP sequence with slice positions matching the T1 mapping images (repetition time/echo time/, 2.4 ms/1.0 ms; flip angle, 70°; field of view, 320–340 × 262–278 mm2; slice thickness, 8 mm; bandwidth, 1,093 Hz/pixel; generalized autocalibrating partially parallel acquisitions acceleration factor, 2). Late gadolinium enhancement (LGE) images were collected using a phase-sensitive inversion-recovery gradient-echo pulse sequence (repetition time/echo time, 5.2 ms/1.96 ms; flip angle, 20°; voxel size, 1.4×1.4×8.0 mm3) in the same views as the cine images 10 minutes after intravenous gadolinium (0.015 mmol/kg body weight) injection (14).
CMR image analysis
CMR image analysis was conducted with clinicians blinded to the clinical data and included measurement of cardiac function, myocardial strain, native T1, ECV, and T2, which were semiautomatically measured using cvi42 software version 5.12 (Circle Cardiovascular Imaging, Calgary, Canada) by one observer (Y.G., 4 years of CMR experience). Standard parameters of cardiac structure, function, and feature tracking strain were calculated by outlining the endocardium and epicardium on long- and short-axis cine images at the end-systolic and end-diastolic phases. LA endocardium and epicardium border contours were initially outlined in the four-chamber and two-chamber cine images at the end-diastolic phase, and an automatic tracing algorithm was applied to obtain strain values for each tissue point and to generate strain. LA strain was calculated as the average of measurements from the four- and two-chamber long-axis cines (Figure 2 and Figure S1). Native T1 and T2 values were measured on three short-axis stacks by contouring the endocardium and epicardium and recorded globally (averaging over three short-axis slices). ECV values were obtained from pre- and postcontrast T1 maps indexing for hematocrit and measured within 3 days before each CMR study. The LV LGE pattern was graded based on the Moon Criteria as none, subendocardial, or transmural (15).
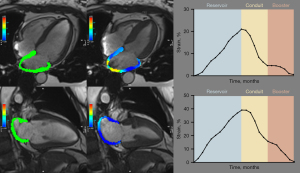
Statistical analysis
The normal distribution of all the clinical features was tested using the Shapiro-Wilk test. Variables are presented as frequencies (percentage), the mean ± standard deviation, or the median [interquartile range (IQR)] as appropriate. There are no missing data. Comparisons between groups were made using the Student’s t-test for normally distributed or the Wilcoxon test for not normally distributed continuous variables, while categorical variables were compared using chi-square tests. Correlations between LA strain and the cardiac structural and functional parameters were assessed using Spearman correlation coefficients.
Univariate Cox proportional hazard models were employed to test the association between the outcome and baseline covariates. Statistically significant predictors of outcome were entered into a multivariable Cox proportional hazards analysis with forward stepwise selection to determine which covariates were independent predictors of mortality. Receiver operating characteristic curves were built to identify the best threshold (highest Youden index) for quantitative imaging variables to predict the outcome. Dichotomized variables were then used for risk-prediction models, given their higher value for translation into clinical practice. Kaplan-Meier survival estimates were calculated, stratified by the optimal cutoff point, and subjected to a log-rank test. The Harrel concordance index (C-index) was calculated to test whether LA strain improved the risk prediction of the established biomarkers, and a likelihood ratio test was performed. As this study focused on the effects of myocardial infiltration on the left atrium, further statistical analyses included LA strain as a variable under investigation adjustment for well-established prognostic markers. Statistical analyses were primarily conducted using SPSS 26 (IBM Corporation, Armonk, NY, USA), whereas C-indexes were calculated using R programming language (The R Foundation for Statistical Computing, Vienna, Austria). All P values were two-sided with a significance level of <0.05.
Results
Study population characteristics
A total of 131 patients were included. The clinical characteristics and CMR parameters are summarized in Table 1. Male sex predominated (61%), and the median age at diagnosis was 59 years (IQR, 51–65 years). Subendocardial and transmural LGE was observed in 121 patients (92%).
Table 1
Variables | All participants (N=131) | Primary endpoints | P value | |
---|---|---|---|---|
Negative (n=94) | Positive (n=37) | |||
Age (years) | 59 [51–65] | 57 [51–65] | 60 [53–65] | 0.464 |
Male | 80 [61] | 57 [61] | 23 [62] | 0.872 |
NYHA functional class ≥ II | 93 [71] | 25 [68] | 68 [75] | 0.588 |
dFLC (mg/L) | 202 [101–390] | 210 [92–372] | 195 [108–426] | 0.383 |
NT-proBNP (pg/mL) | 2,024 [759–3,642] | 1,377 [670–2,745] | 3,702 [2,365–6,253] | <0.001 |
Cardiac troponin I (ng/L) | 36 [17–60] | 32 [17–53] | 43 [23–81] | 0.056 |
eGFR (mL/min/1.73 m2) | 87 [72–102] | 88 [74–103] | 84 [65–98] | 0.177 |
LGE pattern | 0.214 | |||
None | 10 [8] | 8 [8] | 2 [5] | |
Subendocardial | 52 [40] | 41 [44] | 11 [30] | |
Transmural | 69 [52] | 45 [48] | 24 [65] | |
Native T1 (ms) | 1,465 [1,419–1,523] | 1,461 [1,424–1,517] | 1,480 [1,406–1,536] | 0.517 |
ECV fraction | 46±8 | 44±8 | 49±10 | 0.018 |
T2 (ms) | 43 [41–46] | 43 [41–45] | 44 [43–46] | 0.399 |
LV EF (%) | 59±13 | 60±13 | 54±13 | 0.01 |
LV end-diastolic volume index (mL/m2) | 73 [61–87] | 72 [61–85] | 77 [60–89] | 0.935 |
LV end-systolic volume index (mL/m2) | 29 [22–40] | 28 [21–38] | 31.9 [23.5–44.2] | 0.102 |
LV mass index (g/m2) | 66 [54–84] | 66 [54–84] | 68 [54–83] | 0.759 |
LV radial strain (%) | 26 [18–34] | 27 [20–35] | 22 [16–27] | 0.013 |
LV circumferential strain (%) | −16 [−19 to −13] | −17 [−20 to −14] | −15 [−17 to −12] | 0.018 |
LV longitudinal strain (%) | −13 [−17 to −9] | −16 [−18–−10] | −10 [−15 to −7] | 0.002 |
Left atrium area (cm2) | 65 [47–87] | 65 [46–87] | 65 [49–89] | 0.896 |
LA reservoir strain (%) | 20 [10–35] | 23 [12–39] | 14 [7–21] | <0.001 |
LA conduit strain (%) | 9 [5–17] | 11 [6–21] | 7 [4–11] | 0.003 |
LA booster strain (%) | 9 [4–17] | 12 [6–21] | 5 [3–12] | <0.001 |
Values are expressed as number [percentage], mean ± standard deviation, or median [interquartile range]. AL-CA, light-chain cardiac amyloidosis; dFLC, difference between involved and uninvolved free light chains; ECV, extracellular volume; EF, ejection fraction; eGFR, estimated glomerular filtration rate; LA, left atrial; LGE, late gadolinium enhancement; LV, left ventricular; NT-proBNP, N-terminal pro–B-type natriuretic peptide; NYHA, New York Heart Association.
There was a strong correlation between LA strain and cardiac structural and functional parameters (Table 2). The strongest correlation was found between LA reservoir strain and LV systolic function (EF, strain).
Table 2
Variables | LA reservoir strain | LA conduit strain | LA booster strain | |||||
---|---|---|---|---|---|---|---|---|
r value | P value | r value | P value | r value | P value | |||
LV EF (%) | 0.54 | <0.001 | 0.47 | <0.001 | 0.52 | <0.001 | ||
LV end-diastolic volume index (mL/m2) | −0.04 | 0.624 | 0.03 | 0.75 | −0.05 | 0.572 | ||
LV end-systolic volume index (mL/m2) | −0.38 | <0.001 | −0.29 | 0.001 | −0.38 | <0.001 | ||
LV mass index (g/m2) | −0.37 | <0.001 | −0.39 | <0.001 | −0.29 | 0.001 | ||
LV radial strain (%) | 0.65 | <0.001 | 0.61 | <0.001 | 0.6 | <0.001 | ||
LV circumferential strain (%) | −0.68 | <0.001 | −0.65 | <0.001 | −0.6 | <0.001 | ||
LV longitudinal strain (%) | −0.47 | <0.001 | −0.47 | <0.001 | −0.41 | <0.001 | ||
LA area (cm2) | −0.44 | <0.001 | −0.28 | 0.001 | −0.47 | <0.001 |
EF, ejection fraction; LA, left atrial; LV, left ventricular.
Association of LA strain with outcome
During a median follow-up of 28 months (IQR, 10–50 months), 37 patients (28%) reached the primary endpoints, and 52 patients (40%) reached the secondary endpoints. The non-survivors tended to have higher NT-proBNP levels, higher ECV, lower LV EF, and worse LV strain. Furthermore, they had worse LA strain, with LA reservoir strain and LA booster strain showing the most significant differences from the survivors.
Serum biomarker NT-proBNP, ECV, LV EF, LV strain, and LA strain were univariably associated with the primary endpoints (all P values <0.05), while NT-proBNP and LA booster strain were univariably associated with the secondary endpoints (all P values <0.05), as shown in Table 3. After adjustments for age and sex, LA strain remained significantly prognostic for the primary endpoints, while LA booster strain remained significantly prognostic for the secondary endpoints (Table S1). Kaplan-Meier curves demonstrated that patients with an NT-proBNP level of ≥2,890 pg/mL, ECV ≥0.5, LV EF ≤63%, LV longitudinal strain ≥17%, LA reservoir strain ≤28%, or LA booster strain ≤7% had significantly shorter survival (Figure 3). Representative case examples of patients with different outcomes are shown in Figure 4.
Table 3
Variables | Primary endpoints | Secondary endpoints | |||||||||
---|---|---|---|---|---|---|---|---|---|---|---|
Univariate analysis | Multivariable forward stepwise selection | Univariate analysis | Multivariable forward stepwise selection | ||||||||
HR (95% CI) | P value | HR (95% CI) | P value | HR (95% CI) | P value | HR (95% CI) | P value | ||||
Age (years) | 1.02 (0.98–1.05) | 0.37 | – | – | 0.99 (0.96–1.02) | 0.597 | – | – | |||
Sex | 0.99 (0.51–1.94) | 0.997 | – | – | 0.75 (0.41–1.37) | 0.348 | – | – | |||
NYHA functional class ≥ II | 0.99 (0.5–1.98) | 0.991 | – | – | 0.97 (0.53–1.79) | 0.923 | – | – | |||
Ln (dFLC) | 1.25 (0.92–1.70) | 0.157 | – | – | 1.12 (0.90–1.41) | 0.311 | – | – | |||
Ln (NT-proBNP) | 2.02 (1.49–2.74) | <0.001 | 1.53 (1.04–2.24) | 0.029 | 1.38 (1.04–1.81) | 0.023 | – | ||||
–Ln (cardiac troponin I) | 1.50 (0.92–2.43) | 0.102 | – | – | 1.06 (0.71–1.57) | 0.779 | – | – | |||
Ln eGFR | 0.40 (0.15–1.04) | 0.059 | – | – | 0.62 (0.24–1.64) | 0.333 | – | – | |||
LGE pattern | 1.69 (0.95–3.00) | 0.073 | – | – | 1.32 (0.83–2.09) | 0.240 | – | ||||
Ln (native T1) | 0.85 (0.03–23.80) | 0.921 | – | – | 2.68 (0.43–16.80) | 0.294 | – | – | |||
ECV fraction | 1.06 (1.02–1.11) | 0.005 | – | – | 1.03 (0.99–1.07) | 0.147 | – | – | |||
Ln T2 | 1.66 (0.02–162.22) | 0.829 | – | – | 7.29 (0.23–28.26) | 0.259 | – | – | |||
LV EF (%) | 0.97 (0.94–0.99) | 0.01 | – | – | 0.99 (0.96–1.01) | 0.262 | – | – | |||
Ln LV end-diastolic volume index | 0.62 (0.16–2.44) | 0.498 | – | – | 0.62 (0.18–2.20) | 0.463 | – | – | |||
Ln LV end-systolic volume index | 1.69 (0.82–3.49) | 0.155 | – | – | 1.08 (0.56–2.08) | 0.820 | – | – | |||
Ln LV mass index | 1.04 (0.36–3.05) | 0.942 | – | – | 0.75 (0.29–1.99) | 0.567 | – | – | |||
Ln LV radial strain | 0.44 (0.22–0.89) | 0.023 | – | – | 0.42 (0.29–1.50) | 0.474 | – | – | |||
Ln LV circumferential strain | 0.36 (0.14–0.96) | 0.041 | – | – | 0.71 (0.29–1.76) | 0.460 | – | – | |||
Ln LV longitudinal strain | 0.31 (0.14–0.71) | 0.006 | – | – | 0.74 (0.36–1.54) | 0.742 | – | – | |||
Ln LA area | 1.03 (0.48–2.22) | 0.937 | – | – | 1.11 (0.53–2.32) | 0.781 | – | – | |||
Ln LA reservoir strain | 0.49 (0.36–0.67) | <0.001 | – | – | 0.70 (0.48–1.02) | 0.062 | – | – | |||
Ln LA conduit strain | 0.42 (0.28–0.63) | <0.001 | – | – | 0.77 (0.55–1.09) | 0.140 | – | – | |||
Ln LA booster strain | 0.52 (0.36–0.74) | <0.001 | 0.64 (0.42–0.96) | 0.031 | 0.68 (0.49–0.93) | 0.015 | 0.68 (0.49–0.93) | 0.015 |
Statistically significant predictors of outcome were entered into a multivariable Cox proportional hazards analysis with forward stepwise selection. dFLC, difference between involved and uninvolved free light chains; ECV, extracellular volume; EF, ejection fraction; eGFR, estimated glomerular filtration rate; LA, left atrial; LGE, late gadolinium enhancement; LV, left ventricular; NT-proBNP, N-terminal pro–B-type natriuretic peptide; NYHA, New York Heart Association; HR, hazard ratio; CI, confidence interval.
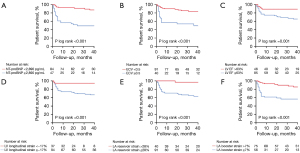
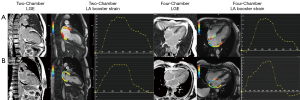
Prognostic value of LA strain
In the multivariable-adjusted competing risk models (Table 3), reduced LA booster strain remained associated with a higher risk of all-cause mortality [hazard ratio (HR) 0.64, 95% confidence interval (CI): 0.42–0.96; P=0.031] and the secondary endpoint (HR 0.68, 95% CI: 0.49–0.93; P=0.015) suggesting that LA booster strain is the most discriminating CMR parameter. Among the metrics of cardiac function, LA reservoir strain (C-index 0.72; 95% CI: 0.64–0.80) and LA booster strain (C-index 0.71; 95% CI: 0.64–0.80) showed superior accuracy in predicting the risk of mortality (Table 4).
Table 4
Variable | Concordance index (95% CI) | △ vs. LV EF | P value |
---|---|---|---|
LV EF | 0.60 (0.59–0.77) | – | – |
LV radial strain | 0.55 (0.51–0.58) | 0.05 (0.02–0.26) | 0.109 |
LV circumferential strain | 0.54 (0.50–0.58) | 0.06 (0.03–0.27) | 0.085 |
LV longitudinal strain | 0.65 (0.56–0.75) | 0.05 (0.02–0.21) | 0.276 |
LA reservoir strain | 0.72 (0.64–0.80) | 0.12 (0.02–0.21) | 0.011 |
LA conduit strain | 0.67 (0.58–0.78) | 0.07 (0.01–0.19) | 0.064 |
LA booster strain | 0.71 (0.64–0.80) | 0.11 (0.03–0.21) | 0.010 |
EF, ejection fraction; LA, left atrial; LV, left ventricular; CI, confidence interval.
The LA strain’s predictive value was further evaluated when well-established prognostic markers were added. As shown in Table 5, LA booster strain was a significant prognostic factor for mortality after corrections were made for NT-proBNP (HR 0.65, 95% CI: 0.43–0.97; P=0.034); LA strain was a significant prognostic factor for mortality after corrections were made for myocardial amyloid burden (LGE pattern and ECV fraction) and LV function (LV EF and LV strain) (all P values <0.05). Moreover, LA booster strain was a significant prognostic factor for secondary endpoints after corrections were made for myocardial amyloid burden and LV function (all P values <0.05). The addition of LA booster strain to known clinical predictors (NT-proBNP and ECV) improved the discrimination (NT-proBNP + ECV: C-index =0.680; NT-proBNP + ECV + LA booster strain: C-index =0.742) and the goodness of fit (log-likelihood ratio test P<0.001). These results indicate that LA booster strain was an incremental predictor of the outcome, even after adjustments were made for other known clinical predictors.
Table 5
Adjustment for variables | LA reservoir strain | LA conduit strain | LA booster strain | |||||
---|---|---|---|---|---|---|---|---|
HR (95% CI) | P | HR (95% CI) | P | HR (95% CI) | P | |||
Primary endpoints | ||||||||
Adjusted for NT-proBNP | 0.64 (0.38–1.09) | 0.098 | 0.73 (0.47–1.14) | 0.163 | 0.65 (0.43–0.97) | 0.034 | ||
Adjusted for LGE pattern | 0.40 (0.24–0.64) | <0.001 | 0.52 (0.34–0.80) | 0.003 | 0.49 (0.34–0.69) | <0.001 | ||
Adjusted for ECV fraction | 0.44 (0.26–0.75) | 0.002 | 0.59 (0.38–0.93) | 0.023 | 0.50 (0.33–0.76) | 0.001 | ||
Adjusted for LV EF | 0.43 (0.26–0.71) | 0.001 | 0.57 (0.37–0.88) | 0.011 | 0.51 (0.36–0.74) | <0.001 | ||
Adjusted for LV radial strain | 0.43 (0.25–0.72) | 0.002 | 0.57 (0.35–0.93) | 0.023 | 0.51 (0.35–0.74) | <0.001 | ||
Adjusted for LV circumferential strain | 0.40 (0.23–0.69) | 0.001 | 0.54 (0.33–0.88) | 0.013 | 0.50 (0.35–0.72) | <0.001 | ||
Adjusted for LV longitudinal strain | 0.50 (0.31–0.80) | 0.004 | 0.63 (0.41–0.99) | 0.042 | 0.55 (0.38–0.79) | 0.001 | ||
Secondary endpoints | ||||||||
Adjusted for NT-proBNP | 0.88 (0.53–1.43) | 0.594 | 0.95 (0.62–1.34) | 0.821 | 0.77 (0.52–1.14) | 0.187 | ||
Adjusted for LGE pattern | 0.72 (0.45–1.14) | 0.156 | 0.82 (0.54–1.22) | 0.333 | 0.68 (0.47–0.98) | 0.036 | ||
Adjusted for ECV fraction | 0.73 (0.44–1.21) | 0.224 | 0.96 (0.56–1.33) | 0.491 | 0.67 (0.45–0.99) | 0.042 | ||
Adjusted for LV EF | 0.68 (0.41–1.13) | 0.139 | 0.81 (0.53–1.23) | 0.320 | 0.66 (0.45–0.96) | 0.030 | ||
Adjusted for LV radial strain | 0.69 (0.40–1.17) | 0.165 | 0.81 (0.51–1.29) | 0.379 | 0.65 (0.44–0.96) | 0.030 | ||
Adjusted for LV circumferential strain | 0.68 (0.40–1.17) | 0.167 | 0.81 (0.50–1.31) | 0.387 | 0.65 (0.44–0.96) | 0.031 | ||
Adjusted for LV longitudinal strain | 0.74 (0.47–1.17) | 0.201 | 0.84 (0.55–1.28) | 0.410 | 0.68 (0.47–0.99) | 0.041 |
CMR, cardiac magnetic resonance; ECV, extracellular volume; EF, ejection fraction; LA, left atrial; LGE, late gadolinium enhancement; LV, left ventricular; NT-proBNP, N-terminal pro-B-type natriuretic peptide; HR, hazard ratio; CI, confidence interval.
Discussion
This study evaluated the prognostic impact of LA strain in AL-CA, with a specific focus on stage II patients. LA strain was strongly associated with outcome and demonstrated prognostic significance for risk stratification. Notably, LA booster strain was superior to metrics of LV function in predicting outcomes. Thus, LA strain measurement should be included as part of risk stratification in patients with stage II AL-CA.
According to the relevant research, an interaction exists between atrial activity and ventricular function; atrial changes precede ventricular changes and are considered to be an early sign of ventricular abnormality (16). LA strain has emerged as a novel noninvasive marker that can be measured on standard CMR cine images, facilitating its application in clinical practice. LA strain measures myocardial deformation throughout the cardiac cycle, and such pancycle assessment may provide a unique assessment of both LA and LV function.
CMR-derived ECV has unique advantages in determining the extent of myocardial amyloid burden (17). We confirmed that ECV fraction had prognostic value in predicting adverse outcomes in patients with stage II disease. Progressive amyloid deposits accumulate not only in the ventricles but also in the LA, resulting in significantly increased LA stiffness (18,19). LA stiffness has been recently identified as an independent prognostic marker and is associated with a reduction of LA strain. We found a close correlation between the impairment of the three phasic components of LA function and LV dysfunction. This indicates that amyloid infiltration progressively affects both LV and LA function in parallel. It has been demonstrated that LA function, particularly LA strain, can be used to reflect the degree of LV diastolic dysfunction and is more sensitive than LA size or volume (20-23). Diastolic filling mainly occurs during early diastole in healthy, compliant ventricles. However, in cardiac amyloidosis, characterized by small, noncompliant ventricles, there is increased dependence on late diastolic filling facilitated by atrial contraction (24). In addition, chronically elevated LV filling pressures have been shown to lead to eccentric LA hypertrophy and remodeling, which in turn results in reduced contractility (25). LA strains reflect the combined adverse impact of impaired atrial compliance and reduced booster pump function. LA booster strain, indicating decreased contractile ability, is likely the initial and most sensitive strain component to be affected.
Benjamin et al. (12) reported that LA booster strain and indexed minimum and maximum LA volumes were significantly associated with the composite outcome of death or heart failure hospitalization among patients with cardiac amyloidosis. In line with this research, our study demonstrated that LA booster strain is a strong independent predictor of clinical outcome. Hence, our findings highlighted the capability and effectiveness of LA strain for long-term risk stratification in patients with AL-CA. The superior prognostic value of LA booster strain validated its strong sensitivity for prognosis. The statistical selection algorithm identified booster strain as a significant independent risk factor, indicating that LA strain can provide additional prognostic information in AL-CA beyond amyloid deposition assessed through contrast-enhanced imaging marker and LV function assessed through EF and strain analysis. Moreover, incorporating LA booster strain into established clinical risk markers significantly enhanced both discrimination and goodness of fit. CMR-derived LA strain could identify underdiagnosed patients with poor prognosis and substantially improve patient selection for the intensification of medical therapy in stage II AL-CA.
Limitations
Several limitations of this research should be acknowledged. This study was conducted at a single center with a relatively small sample size. A larger patient population is necessary to validate or refine the current findings, as it would allow for more robust parametric modeling. Further work is needed to confirm the hypothesis linking CMR-derived LA strain to the prognosis of patients with AL. Due to the novelty of LA strain, no reference data from healthy control participants are available. The cutoff of LA strain, obtained from receiver operating characteristic analysis of the 131 participants, may not represent the ground truth, which should ideally be derived from large populations.
Conclusions
Our findings highlight the potential clinical value of LA strain for identifying underdiagnosed patients with poor prognosis. LA booster strain is a highly promising parameter with independent prognostic value in patients with stage II AL-CA and is superior to metrics of LV function.
Acknowledgments
Funding: This study was supported by
Footnote
Reporting Checklist: The authors have completed the STROBE reporting checklist. Available at https://qims.amegroups.com/article/view/10.21037/qims-24-1025/rc
Conflicts of Interest: All authors have completed the ICMJE uniform disclosure form (available at https://qims.amegroups.com/article/view/10.21037/qims-24-1025/coif). The authors have no conflicts of interest to declare.
Ethical Statement: The authors are accountable for all aspects of the work in ensuring that questions related to the accuracy or integrity of any part of the work are appropriately investigated and resolved. This study was conducted in accordance with the Declaration of Helsinki (as revised in 2013) and was approved by the Institutional Ethics Committee for Human Research at Peking Union Medical College Hospital (Beijing, China) (IRB protocol No. JS1470). All participants provided written informed consent before enrollment.
Open Access Statement: This is an Open Access article distributed in accordance with the Creative Commons Attribution-NonCommercial-NoDerivs 4.0 International License (CC BY-NC-ND 4.0), which permits the non-commercial replication and distribution of the article with the strict proviso that no changes or edits are made and the original work is properly cited (including links to both the formal publication through the relevant DOI and the license). See: https://creativecommons.org/licenses/by-nc-nd/4.0/.
References
- Gertz MA. Immunoglobulin light chain amyloidosis: 2022 update on diagnosis, prognosis, and treatment. Am J Hematol 2022;97:818-29. [Crossref] [PubMed]
- Gertz MA, Dispenzieri A. Systemic Amyloidosis Recognition, Prognosis, and Therapy: A Systematic Review. JAMA 2020;324:79-89. [Crossref] [PubMed]
- Chen Z, Shi A, Wang Z, Chen Y, Lin Y, Su M, Dong H, Laptseva N, Hu Y, Flammer AJ, Duru F, Jin W, Chen L. Prognostic value of plasma big endothelin-1 in patients with light chain cardiac amyloidosis. Heart 2024;110:1124-32. [Crossref] [PubMed]
- Clerc OF, Datar Y, Cuddy SAM, Bianchi G, Taylor A, Benz DC, Robertson M, Kijewski MF, Jerosch-Herold M, Kwong RY, Ruberg FL, Liao R, Di Carli MF, Falk RH, Dorbala S. Prognostic Value of Left Ventricular (18)F-Florbetapir Uptake in Systemic Light-Chain Amyloidosis. JACC Cardiovasc Imaging 2024;17:911-22. [Crossref] [PubMed]
- Guo Y, Li X, Wang Y. State of the Art: Quantitative Cardiac MRI in Cardiac Amyloidosis. J Magn Reson Imaging 2022;56:1287-301. [Crossref] [PubMed]
- Wan K, Sun J, Yang D, Liu H, Wang J, Cheng W, Zhang Q, Zeng Z, Zhang T, Greiser A, Jolly MP, Han Y, Chen Y. Left Ventricular Myocardial Deformation on Cine MR Images: Relationship to Severity of Disease and Prognosis in Light-Chain Amyloidosis. Radiology 2018;288:73-80. [Crossref] [PubMed]
- Rosca M, Lancellotti P, Popescu BA, Piérard LA. Left atrial function: pathophysiology, echocardiographic assessment, and clinical applications. Heart 2011;97:1982-9. [Crossref] [PubMed]
- Zhang R, Cui C, Wang S, Wang Y, Liu R, Liu L. Interaction effect of hypertension and obesity on left atrial phasic function: a three-dimensional echocardiography study. Quant Imaging Med Surg 2023;13:4463-74. [Crossref] [PubMed]
- Leng S, Ge H, He J, Kong L, Yang Y, Yan F, Xiu J, Shan P, Zhao S, Tan RS, Zhao X, Koh AS, Allen JC, Hausenloy DJ, Mintz GS, Zhong L, Pu J. Long-term Prognostic Value of Cardiac MRI Left Atrial Strain in ST-Segment Elevation Myocardial Infarction. Radiology 2020;296:299-309. [Crossref] [PubMed]
- Li Y, Xu Y, Tang S, Jiang X, Li W, Guo J, Yang F, Xu Z, Sun J, Han Y, Zhu Y, Chen Y. Left Atrial Function Predicts Outcome in Dilated Cardiomyopathy: Fast Long-Axis Strain Analysis Derived from MRI. Radiology 2022;302:72-81. [Crossref] [PubMed]
- Stojanovska J, Topaloglu N, Fujikura K, Khazai B, Ibrahim ES, Tsodikov A, Bhave NM, Kolias TJ. Decreased Left Atrial Reservoir Strain Is Associated with Adverse Outcomes in Restrictive Cardiomyopathy. J Clin Med 2022;11:4116. [Crossref] [PubMed]
- Benjamin MM, Arora P, Munir MS, Darki A, Liebo M, Yu M, Syed MA, Kinno M. Association of Left Atrial Hemodynamics by Magnetic Resonance Imaging With Long-Term Outcomes in Patients With Cardiac Amyloidosis. J Magn Reson Imaging 2023;57:1275-84. [Crossref] [PubMed]
- Comenzo RL, Reece D, Palladini G, Seldin D, Sanchorawala V, Landau H, Falk R, Wells K, Solomon A, Wechalekar A, Zonder J, Dispenzieri A, Gertz M, Streicher H, Skinner M, Kyle RA, Merlini G. Consensus guidelines for the conduct and reporting of clinical trials in systemic light-chain amyloidosis. Leukemia 2012;26:2317-25. [Crossref] [PubMed]
- Messroghli DR, Moon JC, Ferreira VM, Grosse-Wortmann L, He T, Kellman P, Mascherbauer J, Nezafat R, Salerno M, Schelbert EB, Taylor AJ, Thompson R, Ugander M, van Heeswijk RB, Friedrich MG. Clinical recommendations for cardiovascular magnetic resonance mapping of T1, T2, T2* and extracellular volume: A consensus statement by the Society for Cardiovascular Magnetic Resonance (SCMR) endorsed by the European Association for Cardiovascular Imaging (EACVI). J Cardiovasc Magn Reson 2017;19:75. [Crossref] [PubMed]
- Fontana M, Pica S, Reant P, Abdel-Gadir A, Treibel TA, Banypersad SM, et al. Prognostic Value of Late Gadolinium Enhancement Cardiovascular Magnetic Resonance in Cardiac Amyloidosis. Circulation 2015;132:1570-9. [Crossref] [PubMed]
- Longobardo L, Zito C, Carerj S, Khandheria BK. Left atrium in heart failure with preserved ejection fraction: the importance of function before anatomy. Eur Heart J Cardiovasc Imaging 2017;18:730-1. [Crossref] [PubMed]
- Korosoglou G, Giusca S, André F, Aus dem Siepen F, Nunninger P, Kristen AV, Frey N. Diagnostic Work-Up of Cardiac Amyloidosis Using Cardiovascular Imaging: Current Standards and Practical Algorithms. Vasc Health Risk Manag 2021;17:661-73. [Crossref] [PubMed]
- Bandera F, Martone R, Chacko L, Ganesananthan S, Gilbertson JA, Ponticos M, et al. Clinical Importance of Left Atrial Infiltration in Cardiac Transthyretin Amyloidosis. JACC Cardiovasc Imaging 2022;15:17-29. [Crossref] [PubMed]
- Versteylen MO, Brons M, Teske AJ, Oerlemans MIFJ. Restrictive Atrial Dysfunction in Cardiac Amyloidosis: Differences between Immunoglobulin Light Chain and Transthyretin Cardiac Amyloidosis Patients. Biomedicines 2022;10:1768. [Crossref] [PubMed]
- Kim J, Yum B, Palumbo MC, Sultana R, Wright N, Das M, You C, Moskowitz CS, Levine RA, Devereux RB, Weinsaft JW. Left Atrial Strain Impairment Precedes Geometric Remodeling as a Marker of Post-Myocardial Infarction Diastolic Dysfunction. JACC Cardiovasc Imaging 2020;13:2099-113. [Crossref] [PubMed]
- Frydas A, Morris DA, Belyavskiy E, Radhakrishnan AK, Kropf M, Tadic M, Roessig L, Lam CSP, Shah SJ, Solomon SD, Pieske B, Pieske-Kraigher E. Left atrial strain as sensitive marker of left ventricular diastolic dysfunction in heart failure. ESC Heart Fail 2020;7:1956-65. [Crossref] [PubMed]
- Morris DA, Belyavskiy E, Aravind-Kumar R, Kropf M, Frydas A, Braunauer K, Marquez E, Krisper M, Lindhorst R, Osmanoglou E, Boldt LH, Blaschke F, Haverkamp W, Tschöpe C, Edelmann F, Pieske B, Pieske-Kraigher E. Potential Usefulness and Clinical Relevance of Adding Left Atrial Strain to Left Atrial Volume Index in the Detection of Left Ventricular Diastolic Dysfunction. JACC Cardiovasc Imaging 2018;11:1405-15. [Crossref] [PubMed]
- Thomas L, Marwick TH, Popescu BA, Donal E, Badano LP. Left Atrial Structure and Function, and Left Ventricular Diastolic Dysfunction: JACC State-of-the-Art Review. J Am Coll Cardiol 2019;73:1961-77. [Crossref] [PubMed]
- Fontana M, Patel RK, Martinez-Naharro A. Atrial Involvement in Cardiac Amyloidosis: Beyond Dilatation. JACC CardioOncol 2020;2:732-4. [Crossref] [PubMed]
- Pellicori P, Zhang J, Lukaschuk E, Joseph AC, Bourantas CV, Loh H, Bragadeesh T, Clark AL, Cleland JG. Left atrial function measured by cardiac magnetic resonance imaging in patients with heart failure: clinical associations and prognostic value. Eur Heart J 2015;36:733-42. [Crossref] [PubMed]