Medical imaging diagnosis of orthopedic prosthesis-associated infections: a narrative review
Introduction
In recent years, patients have derived significant benefits from orthopedic prostheses. However, orthopedic prosthesis-associated infections (OPAI) represent one of the most serious complications of this intervention, particularly in the case of open fractures. Reports indicate that 14.8% of total hip arthroplasties in the United States require surgical intervention each year due to infections (1). Multiple surgical debridements, internal fixation procedures, or prosthesis removal are often necessary, resulting in complications associated with surgical failure, increased healthcare costs, and patient disability. As a result, OPAI pose an urgent challenge for orthopedic surgeons (2-4). Regarding the application of imaging in orthopedic internal fixation systems, primarily involving digital navigation in the early stages and imaging evaluation in later stages, there currently exists no universally recognized optimal detection method or diagnostic standard, despite the availability of various diagnostic techniques. Although diagnostic imaging is indispensable for the management of OPAI, the currently published guidelines do not sufficiently cover the diagnosis of OPAI by medical imaging and there are no detailed diagnostic criteria for this disease. Several published guidelines relating to OPAI are summarized in Table 1 (5-12).
Table 1
Society | PJI | FRI | Otherwise |
---|---|---|---|
IDSA | 2013 diagnosis and management of prosthetic joint infection: clinical practice guidelines by the Infectious Diseases Society of America (5) | ||
EBJIS | 2021 the EBJIS definition of periprosthetic joint infection (6) | 2019 consensus document for the diagnosis of peripheral bone infection in adults: a joint paper by the EANM, EBJIS, and ESR (with ESCMID endorsement) (7) | |
AAOS | 2021 management of periprosthetic infection after reverse shoulder arthroplasty (8) | 2013 the new AAOS-ADA clinical practice guideline on prevention of orthopedic implant infection in patients undergoing dental procedures (9) | |
Chinese Orthopedic Association | 2021 Chinese expert consensus on diagnosis and treatment of infection after fracture fixation (3) | ||
ICM | 2019 definition of periprosthetic joint infection, 2018 Philadelphia Consensus Update (10) | ||
MSIS | 2011 New definition for periprosthetic joint infection: from the Workgroup of the Musculoskeletal Infection Society (11) | ||
EANM | 2016 nuclear medicine imaging of posttraumatic osteomyelitis (12) |
PJI, prosthetic joint infection; FRI, fracture-related infection; IDSA, Infectious Diseases Society of America; EBJIS, European Society for Bone and Joint Infections; AAOS, American Academy of Orthopedic Surgeons; ICM, International Consensus Conference; MSIS, Musculoskeletal Infections Association; EANM, European Association of Nuclear Medicine; ADA, American Dental Association; ESR, European Society or Radiology; ESCMID, European Society of Microbiology and Infectious Diseases.
Ultrasound (US) can be employed to identify potential warning signs of infection following arthroplasty; however, its efficacy diminishes when detecting deep-seated lesions (13). X-ray examination is beneficial to clarify the general scope of bone infection, and although indispensable, it lacks specificity (14). Computed tomography (CT) examinations are valuable for assessing the condition of bone tissue in detail, particularly in identifying necrotic bone within a lesion. Conversely, magnetic resonance imaging (MRI) provides a clearer depiction of the extent of infection and aids in delineating the boundaries for debridement, effectively detecting abnormal signals associated with bone infections. However, the diagnostic accuracy of both MRI and CT can be significantly compromised by artifacts produced by implants (15). In recent years, nuclear imaging has become increasingly employed for diagnosing orthopedic infections. This trend is based on the observation that metabolic changes often precede morphological alterations, and functional imaging modalities typically reveal pathological changes more promptly than conventional imaging techniques (16). Thus, this study aimed to: (I) identify the potential imaging tools available to diagnose OPAI (including X-ray, US, CT, MRI, and nuclear medicine); (II) summarize the mechanisms and features by which each imaging modality can or cannot identify infection (Tables 2,3). We present this article in accordance with the Narrative Review reporting checklist (available at https://qims.amegroups.com/article/view/10.21037/qims-24-403/rc).
Table 2
Radiographic imaging | Diagnostic criteria | Values and characteristics | Limitations and disadvantages |
---|---|---|---|
Ultrasound | Soft tissue thickening and congestion | Unaffected by metal artifacts; no ionizing radiation; low cost; can be used to guide injections and biopsies; good soft tissue assessment | Highly dependent on operator assessment; poor assessment of deep lesions |
X-ray radiography | Radiolucency, osteolysis, displacement | Low cost; higher spatial resolution; large imaging field of view | Ionizing radiation; insufficient sensitivity to soft tissue lesions; poor detection of complications such as osteolysis |
Computed tomography | Abnormal soft tissue density, periprosthetic osteolysis without distensible periosteal reaction, enlarged iliac lymph nodes | Prosthesis alignment to line change situation; non-invasive; osteolysis shows well; three-dimensional imaging | Susceptibility to metallic artifacts; ionizing radiation |
Magnetic resonance imaging | On T2-weighted images, it appears as high signal intensity and does not enhance with gadolinium | Extremely sensitive to soft tissue, including neurovascular structures; no ionizing radiation; ability to image any cross-section; three-dimensional imaging; multi-sequence | Susceptible to metal artifacts; time-consuming |
Table 3
Nuclear medicine imaging | Advantages | Limitations |
---|---|---|
68Ga-citrate | High-resolution imaging; assessment of metabolic activity; distinguish between infectious and non-infectious lesions | Requires delayed imaging (48 h), low specificity, limited spatial resolution |
18F-fluorodeoxyglucose | High resolution, high efficiency, simple operation, no need for direct handling of blood products | Chronic infections are poorly diagnosed |
Radiolabeled leukocytes | High specificity in the peripheral skeleton | Poor central skeletal specificity, susceptibility to leukocyte status in vivo |
99mTc-MDP three-phase bone scan/99mTc-HMPAO-labeled WBC scan | Preferred imaging for orthopedic implant infections | Time-consuming, technically difficult, limited availability, low spatial resolution |
99mTc HMPAO leukocyte/99mTc sulfur colloid bone (phytate) marrow scintigraphy | Less affected by leukocyte status in the body | Lack of sulfur colloids in some countries (inositol hexaphosphate substituted) |
99mTc-labeled bisphosphonates | High sensitivity; rapid imaging; wide availability | Low specificity in post-traumatic patients and post-surgical patients |
Methods
The data was obtained from PubMed and Web of Science Core Collection on 1 February 2024. The study includes original articles and reviews written and published in English between 2014 and 2024 (Table 4). The retrieval strategy employed was as follows: ((((Orthopedics[Title/Abstract]) OR (bone[Title/Abstract])) AND ((Infections[Title/Abstract]) OR (Infection[Title/Abstract]))) AND ((((artificial joints[Title/Abstract]) OR (fracture endoprostheses[Title/Abstract])) OR (implanted[Title/Abstract])) OR (Prosthesis[Title/Abstract]))) AND ((((((((Medical Imaging[Title/Abstract]) OR (CT[Title/Abstract])) OR (MRI[Title/Abstract])) OR (PET[Title/Abstract])) OR (SPECT[Title/Abstract])) OR (Medical Imaging diagnosis[Title/Abstract])) OR (ultrasound[Title/Abstract])) OR (Nuclear Medicine[Title/Abstract])).
Table 4
Items | Specification |
---|---|
Date of search | 1 February 2024 |
Databases and other sources searched | PubMed and Web of Science Core Collection |
Search terms used | ((((Orthopedics[Title/Abstract]) OR (bone[Title/Abstract])) AND ((Infections[Title/Abstract]) OR (Infection[Title/Abstract]))) AND ((((artificial joints[Title/Abstract]) OR (fracture endoprostheses[Title/Abstract])) OR (implanted[Title/Abstract])) OR (Prosthesis[Title/Abstract]))) AND ((((((((Medical Imaging[Title/Abstract]) OR (CT[Title/Abstract])) OR (MRI[Title/Abstract])) OR (PET[Title/Abstract])) OR (SPECT[Title/Abstract])) OR (Medical Imaging diagnosis[Title/Abstract])) OR (ultrasound[Title/Abstract])) OR (Nuclear Medicine[Title/Abstract])) |
Timeframe | 2014–2024 |
Inclusion and exclusion criteria | Inclusion criteria: review or research articles in English |
Exclusion criteria: duplicates, full text not available, not related to medical imaging | |
Selection process | After remove of duplicates, two independent authors conducted a comprehensive review of the titles and abstracts of the remaining literature to assess their eligibility for inclusion. Consensus was obtained from the advice o f a senior physician |
A total of 259 articles were retrieved from PubMed, and 225 articles were obtained from the Web of Science Core Collection. After importing these articles into EndNote (Clarivate, Philadelphia, PN, USA) and using the software to remove duplicates, two independent authors conducted a comprehensive review of the titles and abstracts of the remaining literature to assess their eligibility for inclusion. Following this initial screening, the authors meticulously analyzed the abstracts and examined the full texts to confirm the final inclusion of 55 articles.
Main types of orthopedic prostheses
Existing orthopedic prostheses primarily consist of artificial joints, fracture endoprostheses, and other biological or chemical materials implanted in the human body, including bone cement and artificial bones (17-19). Among these, artificial joints and fracture fixation devices are two of the most common types of orthopedic prostheses. Overall, the clinical application of orthopedic implants is extensive and has significantly contributed to the advancement of the field of orthopedics.
Ultrasonography
US is a diagnostic technique that employs sound waves, which are reflected at the boundaries between tissues of differing densities, to generate real-time images. This non-invasive imaging modality offers visualization of the body without the use of ionizing radiation, making it a preferred option for a diverse range of patients (20). US can effectively identify potential warning signs of infection following arthroplasty, such as prosthesis instability or fractures. Research indicates that US has good diagnostic accuracy in detecting fractures in both the upper and lower extremities (21). Additionally, it is capable of identifying loosening of the prosthesis and assessing prosthesis movement. A previous study showed that US could detect periprosthetic complications, yet its effectiveness in identifying infections has remained a subject of debate (22). Furthermore, traditional diagnostic US is highly dependent on the operator’s skill and is less effective for evaluating deep lesions.
In addition to conventional US imaging techniques, ultrasonic lysis also contributes to the diagnosis of OPAI. This method utilizes cavitation to detach the biofilm surrounding the prosthesis, effectively releasing microorganisms and significantly enhancing the culture detection rate. Notably, this process preserves the activity of pathogens. Recent advancements have led to the continuous research and development of embedded ultrasonic systems (23). These systems can simultaneously monitor prosthesis loosening and temperature changes to aid in diagnosing infections in orthopedic patients. The technology requires only two implanted components: a piezoelectric transducer and a coil that generates a pulse-echo response through a three-coil inductive link. This streamlined approach to smart implants minimizes alterations to established implant designs, facilitating mass-market adoption and suggesting widespread future use.
Differentiating between prosthetic material infections and other complications is crucial for making appropriate management decisions. US imaging plays a valuable role in this process by providing characteristic features that aid in distinguishing infection from other conditions.
- Prosthetic material infections: US imaging can reveal specific signs indicating the presence of an infection in the prosthetic material. These signs may include the presence of pus or purulent material, fluid loculations, increased vascularity, and signs of implant malposition (24).
- Seroma and hematoma: US can assist in differentiating seroma and hematoma from prosthetic material infections by evaluating the fluid collection characteristics and the absence of infectious signs, such as pus. Seromas typically appear as anechoic or hypoechoic fluid collections, whereas hematomas exhibit echogenicity due to clot formation (25).
- Allergic reactions: US can exclude infection when assessing complications related to allergic reactions. It helps to identify soft tissue edema, inflammation, and increased blood flow, which are indicative of an immune response rather than an infectious process (24).
- Mechanical issues and aseptic loosening: US imaging can visualize mechanical issues and aseptic loosening of prosthetic material. It can reveal signs such as implant migration, periprosthetic fluid, or soft tissue abnormalities related to prosthesis instability. These findings are not typically associated with infection (26).
- It is noteworthy that US-guided puncture diagnosis of soft tissue abscess is also a commonly used and effective technique. It offers several benefits and applications that improve accuracy, reduce complications, and aid in drainage procedures. Incorporating US guidance into clinical practice can enhance patient outcomes by optimizing diagnosis, treatment planning, and follow-up care.
Radiographic imaging
X-ray radiography
X-ray imaging is the preferred method for evaluating potential complications in symptomatic patients with OPAI (24). It offers sufficient spatial resolution for various diagnostic applications, and its relatively low cost is a significant advantage. X-ray imaging allows for clear visualization of prosthesis loosening and displacement, as well as the detection of early or late postoperative complications, including heterotopic ossification, prosthesis dislocation, and fractures. As a standard imaging technique, X-ray is widely utilized for postoperative joint replacement evaluation and routine follow-up (26,27). In patients with OPAI, X-ray films frequently reveal radiolucency, osteolysis, displacement, or a combination of these features (28). Dynamic series X-ray examinations, also referred to as stress or functional X-rays, involve capturing images while the patient performs specific movements or weight-bearing activities (29). These examinations offer valuable insights into the stability, alignment, and functionality of orthopedic implants. Dynamic series X-ray examinations hold significant diagnostic value for the assessment and treatment of OPAI. When considering the necessity of repeated X-ray examinations, it is crucial to weigh the benefits of imaging against the potential risks of radiation exposure. Decisions regarding X-ray examinations, including dynamic series X-rays, should be made based on clinical necessity and tailored to the individual patient’s circumstances. However, conventional X-ray plain films lack sufficient sensitivity for detecting soft tissue lesions, resulting in a low detection rate for complications such as osteolysis. Recently, the incorporation of artificial intelligence (AI) has enhanced diagnostic accuracy. For instance, Oka and colleagues developed the VGG16 AI system, which achieves high-precision diagnosis by utilizing dual-plane X-ray images, even with a relatively small dataset (30).
CT
CT is a valuable diagnostic tool for OPAI, as it allows for the identification of severe soft tissue abnormalities surrounding the prosthesis, periprosthetic osteolysis without expansive periosteal reaction, and iliac lymph node enlargement (31). Advances in CT technology have enabled the visualization of both bone structures and soft tissues around the prosthesis. CT examinations provide a high level of detail, facilitating the assessment of prosthesis alignment changes (29). Compared to X-ray imaging, CT scans offer greater accuracy in detecting osteolysis and enable better evaluation of prosthesis dislocation and wear. However, in diagnosing fracture-related infections (FRI), the accuracy of CT is relatively low (32). Nonetheless, CT scans excel in determining the degree of fracture healing and the location and integrity of the prosthesis in FRI cases.
CT examinations utilize ionizing radiation, which can pose potential harm to patients due to the high radiation dose involved. Additionally, CT imaging is susceptible to metal artifacts when evaluating complications following joint replacement surgeries. Metal artifacts can cause disruptions in CT images, resulting in the appearance of hardening artifacts. These artifacts impair the ability to clearly visualize the soft tissue surrounding the prosthesis and can significantly degrade image quality (33).
MRI
MRI is a non-invasive diagnostic technique that utilizes a powerful magnetic field, magnetic field gradients, and radio waves (34). This three-dimensional imaging tool offers several diagnostic advantages, including its ability to generate images without exposing the patient to ionizing radiation. Furthermore, MRI is capable of providing detailed visualization of any anatomical cross-section and exhibits high sensitivity in examining soft tissues. As a result, it allows for the accurate assessment of osteolysis, early detection of bone and muscle infections, and precise evaluation of the extent and severity of infection (29). Consequently, MRI is a valuable tool in the identification of orthopedic prosthesis failure, particularly in cases involving periprosthetic soft tissue abnormalities and bone marrow edema (35).
The use of MRI in the evaluation of orthopedic OPAI raises concerns due to the interaction between the magnetic field and the metal components of the prosthesis. However, research indicates that significant displacement of the prosthesis during the imaging process is infrequent. Moreover, when compared to CT, MRI is more costly, time-consuming, and offers limited value in assessing small periprosthetic osteolytic lesions and prosthesis positioning. Additionally, MRI does not provide guidance for joint punctures or tissue biopsies. Furthermore, the introduction of metal materials alters the B0 magnetic field strength and the Lamor frequency of the surrounding nuclear spins, resulting in distortion of MRI images due to metal artifact interference. Common manifestations of this interference include local loss of signal around the prosthesis and image deformation (36).
Several factors have been identified to contribute to image distortion, including the local frequency offset, type of sequence, scan thickness, and receiver bandwidth. By modifying MRI parameters and contrast parameters, it is possible to reduce artifacts and obtain high-quality diagnostic images. Furthermore, the intensity of the magnetic field is directly proportional to the degree of artifacts. In the diagnosis of OPAI, 1.5T nuclear magnetic resonance equipment is often preferred over 3.0T. Adjusting acquisition parameters can significantly enhance image quality, and the choice of pulse sequence also plays a crucial role. The commonly utilized sequences for diagnosing orthopedic implants via MRI include spin echo (SE), fast spin echo (FSE) imaging techniques, short tau inversion recovery (STIR), and the fat suppression technique known as the Dixon method (37,38).
Studies have shown that MRI can impact implants, and caution is necessary when using this imaging technique (39,40). The interaction between implants and the MRI magnetic field can cause magnetic field induction, potentially resulting in movement or forces acting on metal implants in strong magnetic fields, which may lead to patient discomfort or prosthesis displacement. Therefore, prior to performing an MRI, healthcare providers typically assess the presence of metal implants that may interact with the magnetic field to determine the suitability of the examination. Furthermore, metal implants can cause heating effects during MRI scans, as they may absorb radiofrequency energy and generate heat, potentially causing tissue overheating and burns. To mitigate heating effects, modern MRI machines utilize low specific absorption rate (SAR) sequences and implement safety measures to reduce the risk of thermal injuries.
In recent years, there have been advancements in techniques aimed at reducing susceptibility artifacts in single-point imaging, such as the utilization of images generated solely through phase encoding. Another technique, known as projection reconstruction, allows for the acquisition of gradient echo images with ultrashort echo times to minimize intra-primary dephasing. Pre-polarized MRI involves generating images at low b0 fields to reduce susceptibility effects. View angle tilting (VAT) imaging corrects for geometric displacements caused by b0 inhomogeneity. Furthermore, the development of metal artifact reduction sequences (MARS) has also been introduced (40).
Nuclear medicine
During routine radiographic examinations, indicators of orthopedic OPAI such as peripheral osteolysis, edge loosening, and periarticular calcification are frequently observed; however, these signs typically manifest at later stages of the infection. Metabolic changes often precede morphological alterations, and functional imaging modalities are adept at detecting pathological changes earlier than conventional imaging methods. Unlike anatomical imaging, nuclear imaging is a functional technique that remains unaffected by metal artifacts and can thus facilitate diagnosis before morphological tissue changes occur. Consequently, nuclear imaging technology is gaining recognition as a valuable diagnostic tool for identifying prosthesis infections. Nonetheless, there exists a notable gap in comprehensive research summarizing the application of nuclear imaging in this context.
Ga-citrate-based nuclear imaging
Ga-citrate scintigraphy utilizing gallium-67 (67Ga) has been historically used for the detection of infections, including prosthetic hip infections. Additionally, 68Ga is commonly utilized as a positron-emitting radiopharmaceutical for functional imaging in various applications (41). Positron emission tomography (PET) imaging using 68Ga-labeled tracers has gained popularity in recent years for infection imaging due to its higher resolution and quantification capabilities. In a study by Salomäki et al. (42), it was demonstrated that 68Ga-citrate could effectively differentiate between bone infections and the normal process of bone healing following surgery. Tseng et al. (43) also suggested that 68Ga-citrate PET/CT could be used to distinguish between infectious and non-infectious diseases after arthroplasty. However, the application of 68Ga-citrate scintigraphy for diagnosing OPAI has diminished in recent years due to various limitations, including the requirement for delayed imaging (48 hours), low specificity, and restricted spatial resolution. As a result of these drawbacks and the emergence of more advantageous compounds and techniques, the use of 68Ga-citrate as a standalone diagnostic tool for orthopedic prosthesis infections has become less common.
Radiolabeled leukocytes
Nuclide imaging using indium-111 (111In)-labeled leukocytes has demonstrated a high level of diagnostic accuracy, with a reported sensitivity of more than 95%. However, due to the more favorable imaging properties of 99mTc-labeled leukocytes (99mTc-WBC) following successful labeling with hexamethylpropylene amine oxime (HMPAO), they have replaced 111In-labeled leukocytes in most clinical applications (44). A study found that the sensitivity of the 99mTc-HMPAO-labeled white blood cell (WBC) scan was similar to that of the 99mTc-MDP three-phase bone scan (TBS) for detecting bone infections (45). Moreover, in post-traumatic and postoperative cases, particularly in the surrounding bones, the specificity is also higher, reaching up to 90% (45). However, the interpretation of leukocyte images is complicated by the accumulation of radiolabeled leukocytes not only at the infection site but also in the hematopoietically active bone marrow. The extensive and localized expansion of the hematopoietically active bone marrow makes it challenging to determine whether the leukocyte accumulation indicates infection or involvement of the periprosthetic region. As a result, the specificity of this imaging technique is poor in the central skeleton and in patients with leukopenia.
Additionally, both 111In-WBC and 99mTc-WBC have certain drawbacks, including the labor-intensive preparation process, the requirement for specialized equipment, and the handling of potentially infectious blood. Consequently, there is a need to develop radiopharmaceuticals that can offer comparable clinical performance to radiolabeled leukocytes (46).
Radiolabeled specific antigranulocyte monoclonal antibodies and nonspecific IgG
Radiolabeled antigranulocyte antibodies, such as 99mTc-anti-CD15 immunoglobulin M (IgM) and 99mTc-anti-NCA-95 IgG, have been developed for in vivo labeling of leukocytes and have shown to be effective for the diagnosis of osteoarticular infectious and inflammatory diseases. These antibodies accumulate in infected and inflamed areas primarily through non-specific extravasation, which is a result of increased vascular permeability and specific targeting of infiltrating granulocytes. The blood clearance of intact immunoglobulin G (IgG) antibodies is relatively slow, whereas antibody fragments [Fab' or F(ab')2] and IgM antibodies are cleared more rapidly. A meta-analysis conducted by Pakos et al. (46) reported a sensitivity and specificity of 83% and 80%, respectively, for anti-neutrophil scintigraphy in diagnosing osteoarticular infectious and inflammatory diseases. However, this method is not as effective in patients with neutropenia, and the use of antibiotics can reduce its clinical efficacy (47). The overall sensitivity of radiolabeled antibodies for detecting infections is approximately 80–90%. Although peripheral bone infections can be adequately visualized, the sensitivity decreases when the infection is closer to the spine. This is mainly due to the physiological uptake of normal bone marrow and limited infiltration of WBC, which poses a similar diagnostic challenge to those of 111In-WBC and 99mTc-WBC imaging (48). Radiolabeled nonspecific human IgG also accumulates in infectious and inflammatory areas through non-specific extravasation facilitated by enhanced vascular permeability (49).
99mTc-labeled bisphosphonates
99mTc-labeled bisphosphonates, such as methylene diphosphonate (MDP) and hydroxymethylene diphosphonate (HDP), are widely used radiopharmaceuticals for imaging osteoblast activity. In cases of orthopedic prosthesis infections, there is an increase in osteoblast activity, and the detection of infection using 99mTc-MDP demonstrates high sensitivity (90%) (50). Generally, the TBS with 99mTc-MDP is highly sensitive in detecting bone infection. However, its specificity is influenced by specific circumstances. The specificity is high when the bone is not affected by other pathological conditions (approximately 90%), but it decreases significantly in post-traumatic patients and post-surgical patients (around 35%). Notably, recent advancements in AI have greatly enhanced the efficiency and accuracy of prosthetic joint infection diagnosis, as reported by Nie et al. in 2023 (51).
Reference criteria for differentiating between loosening and infection after total medullary arthroplasty using bone triphasic imaging have been outlined (52). Normal findings include: (I) no radioactive uptake observed at the bone-prosthesis interface; (II) low radioactive uptake noted around the femoral neck-prosthesis; (III) low radioactive uptake observed around the femoral neck and localized to the femoral head-prosthesis; (IV) low radioactive uptake detected around the femoral neck and prosthesis shank. In cases of loosening, the following findings may be present: (I) high radioactive uptake at points of impingement (e.g., large and small rotors, prosthesis ends); (II) radiation uptake identified around the femoral head-prosthesis and femoral neck-prosthesis; (III) radiation uptake around the femoral neck and localized to the prosthetic stem. For cases of infection, single-photon emission computed tomography (SPECT) imaging reveals excessive bone-prosthesis radioactivity uptake as well as radioactivity uptake in the surrounding soft tissues.
99mTc-MDP TBS/99mTc-HMPAO-labeled WBC scan
The TBS technique using 99mTc-MDP involves three distinct phases that provide valuable information about the vascularity, soft tissue abnormalities, and bone metabolism in the area of interest. Blood flow phase: in this phase, the perfusion of the radiotracer is assessed, providing information about the vascularity of the area of interest. Blood pool phase: this phase involves delayed imaging after tracer injection, focusing on the dynamic vascular phase and identifying soft tissue abnormalities. Bone phase: this phase is performed several hours after the injection when the radiotracer accumulates in areas of active bone metabolism, helping identify abnormalities in bone structure or function.
Palestro et al. (53) reported satisfactory results from the combined analysis of radiolabeled leukocytes and bone marrow imaging, achieving over 95% accuracy in diagnosing OPAI. Currently, most authors consider combined leukocyte and bone marrow imaging as the preferred modality for evaluating suspected orthopedic prosthesis infections due to its diagnostic efficacy. Complementing this approach, the combination of 99mTc-MDP TBS and 99mTc-HMPAO-labeled WBC scan (WS) further improves diagnostic accuracy. Kim et al. (54) found that the combination of TBS and WS demonstrated better diagnostic accuracy (91.7%, 11/12) compared to WS alone (81.0%). This is attributed to the fact that MDP uptake reflects perfusion status and osteoblast activity, whereas leukocyte accumulation reflects leukocyte activity. These two imaging methods complement each other, enhancing diagnostic accuracy. For OPAI diagnosis, a gold standard is achieved with sensitivity ranging from 55% to 100% and specificity ranging from 94% to 100%. However, this method is hindered by time-consuming procedures, technical difficulties, limited availability, and sub-optimal spatial resolution.
99mTc HMPAO leukocyte/99mTc sulfur colloid bone (phytate) marrow scintigraphy
Combining leukocyte scintigraphy (LS)/99mTc sulfur colloid bone marrow scintigraphy (BMS) achieves an accuracy of over 90%. This combined imaging approach is founded on the accumulation of both WBC and sulfur colloids in the bone marrow. WBC accumulate in infections, whereas sulfur colloids do not. Therefore, when there is activity observed in the leukocyte image and no corresponding activity in the bone marrow image of the sulfur colloid, the combined analysis of radiolabeled LS/BMS confirms infection (positive result), and vice versa indicates no infection (negative result).
Noronha et al. (55) discovered that 99mTc phytate and 99mTc sulfur colloid have distinct biodistributions. 99mTc phytate is a scandium complex of inositol hexaphosphate that forms colloids in the body by chelating with the divalent ion Ca2+ in the blood, much like free ligands. It tends to accumulate in dense bone rather than bone marrow. Therefore, although its role as a bone marrow imaging agent is limited, it holds significant value in diagnosing OPAI. The combined therapy of radiolabeled leukocyte/BMS demonstrated sensitivity, specificity, positive predictive value (PPV), negative predictive value (NPV), and accuracy rates of 100%, 83%, 83%, 100%, and 91%, respectively, for prosthetic infection diagnosis. In countries where 99mTc sulfur colloid is lacking, 99mTc phytate is often used as a suitable alternative. Thus, it becomes crucial to study the differences between these two contrast agents. Jung et al. (56) reported comparable diagnostic performance in a combined study of 99mTc HMPAO LS/99mTc phytate BMS to other studies utilizing sulfur colloids. Consequently, the combined 99mTc HMPAO LS/99mTc phytate BMS holds promise as an acceptable alternative to combined radiolabeled LS/99mTc sulfur colloid BMS in regions or countries with limited access to 99mTc sulfur colloid.
18F-fluorodeoxyglucose (FDG) PET/CT
Metabolic changes often occur before morphological changes, and functional imaging modalities can detect pathological changes earlier than conventional imaging techniques. Therefore, using PET/CT to identify initial signs of bone infection shows promise. Research has demonstrated that PET/CT can effectively differentiate between bone infection and normal physiological activity. Additionally, the combination of simultaneous hybrid imaging with MRI and PET has the potential to detect early changes in infected tissue and improve the accuracy of assessing the extent of infection in the bone or soft tissues using morphological imaging.
FDG enters cells through glucose transporters, specifically glut-1 and glut-3. Hexokinase then phosphorylates 18F-FDG within the cells, resulting in the formation of FDG-6-phosphate. This phosphorylated deoxyglucose cannot undergo further metabolism, causing 18F-FDG to accumulate in activated lymphocytes, neutrophils, and macrophages, with minimal decrease over time. These characteristics make 18F-FDG PET a suitable imaging technique for assessing various inflammatory and infectious diseases, offering promising prospects in the evaluation and diagnosis of infection and inflammation (57). In recent literature, 18F-FDG PET imaging has been proposed for the detection of OPAI (58). It is suggested that 18F-FDG PET/CT holds great potential in diagnosing and assessing the effectiveness of OPAI treatment. This relatively new method of evaluating periprosthetic infections offers significant advantages over combined leukocyte and bone marrow imaging, including improved resolution, time efficiency, use of a single radiotracer injection, simple operation, swift test completion (within 2 hours), and elimination of the need for direct manipulation of blood products, thereby reducing the risk of contamination by harmful pathogens.
Chatziioannou et al. (59) demonstrated the efficacy of 18F-FDG PET/CT in evaluating the response to anti-infective treatment in prosthetic joint infections, using animal models. Additionally, Shemesh et al. (60) reported that 18F-FDG PET/CT can accurately identify infections and non-infectious causes of non-healing, soft tissue infections, and chronic osteomyelitis following arthroplasty, with a specificity of up to 100%. Kumar et al. (61) investigated the use of 18F-FDG PET/CT for diagnosing infections after hip arthroplasty and reported sensitivity and specificity of 93.7% and 92.3%, respectively. Basu et al. (62) conducted a PET imaging analysis of 221 patients with arthroplasty, showing improved sensitivity (33.3%) and specificity (88.5%) compared to leukapheresis. However, not all studies have concluded that PET imaging surpasses leukocyte imaging; Yue et al. (63) stated that 18F-FDG PET/CT cannot replace leukocyte imaging. To enhance specificity and resolution in 18F-FDG PET/CT imaging, in recent years, there have been efforts to label leukocytes in vitro with 18F-FDG. These labeled leukocytes have been validated for detecting periprosthetic joint infections, demonstrating high specificity, with sensitivity ranging from 87.0% to 93.3%, and specificity ranging from 82.0% to 97.4% (64,65). However, this method has the drawback of being time-consuming and not suitable for diagnosing chronic infections (66,67).
In addition to its successful use in diagnosing periprosthetic joint infection (PJI), qualitative assessment of 18F-FDG PET/CT scanning has also been shown to be effective in diagnosing FRI, with a diagnostic accuracy of 0.83 [95% confidence interval (CI): 0.77–0.89] and an area under the curve (AUC) of 0.84 (95% CI: 0.78–0.91) (68). Notably, the NPV of 0.91 was shown to be significantly higher than that of most other imaging methods, making 18F-FDG PET/CT an excellent tool for patients with chronic or low-grade infections (69). Furthermore, combining qualitative assessment with standardized uptake value (SUV) measurements improves diagnostic accuracy even further, yielding a diagnostic accuracy of 0.86 and an AUC of 0.89 (95% CI: 0.84–0.95). This signifies that the inclusion of SUV measurements enhances diagnostic accuracy, albeit to a relatively small extent. In summary, although 18F-FDG PET/CT is a highly sensitive nuclear imaging technique, it lacks the necessary specificity to clearly differentiate between various conditions in suspected FRI cases (70) (Figures 1-3).
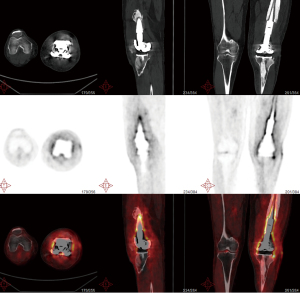
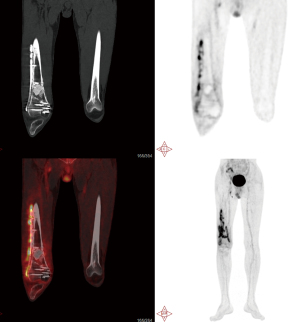
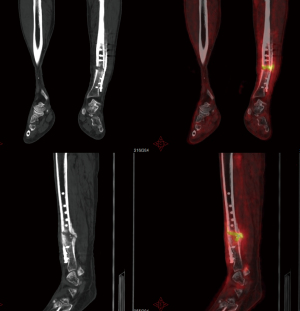
Initial diagnosis and follow-up of infections
The initial diagnosis of orthopedic prosthesis infection requires a comprehensive evaluation that includes the medical history, physical examination, laboratory tests, and imaging studies. For imaging, the initial approach predominantly involves X-ray examinations, such as standard radiographs in multiple views, to assess the implant’s position, stability, and surrounding bone integrity. Additionally, nuclear imaging techniques, including three-phase bone scintigraphy or PET/CT, are utilized to evaluate inflammatory activity around the prosthesis, with positive results indicating the presence of infection. Subsequent management should be tailored to the individual patient’s circumstances. Throughout the treatment process, imaging studies play a crucial role in monitoring the resolution of inflammation and assessing treatment efficacy. Persistent symptoms or ambiguous imaging results may necessitate further diagnostic evaluations, including joint fluid cultures or tissue biopsies. Timely identification, diagnosis, and treatment of OPAI are essential to prevent complications and improve patient recovery outcomes.
Limitations and future directions
The current diagnosis of OPAI lacks standardized definitions for radiological features and the interpretation of radiological findings relies on subjective judgments due to the absence of quantitative criteria. Additionally, the absence of uniform diagnostic criteria hampers comparisons between studies. Inconsistencies in the use of terms such as “radiolucency”, “osteolysis”, “pseudotumor”, and “adverse tissue reaction” further complicate the conduct of meta-analyses. Most studies that we included were retrospective, with a paucity of prospective research on the radiological diagnosis of OPAI. Therefore, the need to establish large-scale, multicenter research protocols is urgent. Despite these limitations, there are promising developments in various technological fronts. In the realm of ultrasonographic diagnosis, the utilization of embedded US systems and post-cultivation US examination has extended beyond mere imaging capabilities, with new applications being explored. Embedded US systems refer to the integration of US technology into medical devices or implants, allowing for real-time imaging capabilities during procedures or continuous monitoring after implantation. Post-cultivation US examination involves using US imaging to assess cultivated tissue or cell cultures for various purposes. Post-cultivation US examination involves using US imaging techniques to evaluate cultivated tissue or cell cultures. US examination helps to evaluate the morphology, viability, and functionality of cultured cells, ensuring their suitability for downstream applications. US also enables non-destructive monitoring of cellular behavior and response to various stimuli, aiding in research and development of therapeutic strategies. In the field of radiographic diagnosis, advancements in CT and MRI artifact removal techniques, radiomics, and AI could significantly enhance the accuracy of OPAI diagnosis. Nuclear medicine has also witnessed notable progress, with the widespread adoption of SPECT/CT fusion imaging and the emergence of 18F-FDG PET/CT, which have demonstrated the crucial role of nuclear medicine diagnostic techniques and their unique metabolic information in the diagnosis of OPAI. Consequently, these developments have become hot topics of research in this field. Furthermore, the development of microorganism-specific diagnostic and therapeutic probes has introduced a novel approach toward enhancing the theranostics of OPAI. Nevertheless, relying on a single imaging modality may be insufficient for achieving a comprehensive diagnosis of OPAI. In cases where suspicion arises, the use of complementary imaging techniques should be considered to improve diagnostic accuracy and reliability.
Acknowledgments
Funding: This study was partially supported by
Footnote
Reporting Checklist: The authors have completed the Narrative Review reporting checklist. Available at https://qims.amegroups.com/article/view/10.21037/qims-24-403/rc
Conflicts of Interest: All authors have completed the ICMJE uniform disclosure form (available at https://qims.amegroups.com/article/view/10.21037/qims-24-403/coif). The authors have no conflicts of interest to declare.
Ethical Statement: The authors are accountable for all aspects of the work in ensuring that questions related to the accuracy or integrity of any part of the work are appropriately investigated and resolved.
Open Access Statement: This is an Open Access article distributed in accordance with the Creative Commons Attribution-NonCommercial-NoDerivs 4.0 International License (CC BY-NC-ND 4.0), which permits the non-commercial replication and distribution of the article with the strict proviso that no changes or edits are made and the original work is properly cited (including links to both the formal publication through the relevant DOI and the license). See: https://creativecommons.org/licenses/by-nc-nd/4.0/.
References
- Arciola CR, Campoccia D, Montanaro L. Implant infections: adhesion, biofilm formation and immune evasion. Nat Rev Microbiol 2018;16:397-409. [Crossref] [PubMed]
- Metsemakers WJ, Morgenstern M, McNally MA, Moriarty TF, McFadyen I, Scarborough M, Athanasou NA, Ochsner PE, Kuehl R, Raschke M, Borens O, Xie Z, Velkes S, Hungerer S, Kates SL, Zalavras C, Giannoudis PV, Richards RG, Verhofstad MHJ. Fracture-related infection: A consensus on definition from an international expert group. Injury 2018;49:505-10. [Crossref] [PubMed]
- Jiang N, Wang BW, Chai YM, Wu XB, Tang PF, Zhang YZ, Yu B. Chinese expert consensus on diagnosis and treatment of infection after fracture fixation. Injury 2019;50:1952-8. [Crossref] [PubMed]
- Li Y, Yang Y, Qing Y, Li R, Tang X, Guo D, Qin Y. Enhancing ZnO-NP Antibacterial and Osteogenesis Properties in Orthopedic Applications: A Review. Int J Nanomedicine 2020;15:6247-62. [Crossref] [PubMed]
- Osmon DR, Berbari EF, Berendt AR, Lew D, Zimmerli W, Steckelberg JM, Rao N, Hanssen A, Wilson WR. Diagnosis and management of prosthetic joint infection: clinical practice guidelines by the Infectious Diseases Society of America. Clin Infect Dis 2013;56:e1-e25. [Crossref] [PubMed]
- McNally M, Sousa R, Wouthuyzen-Bakker M, Chen AF, Soriano A, Vogely HC, Clauss M, Higuera CA, Trebše R. The EBJIS definition of periprosthetic joint infection. Bone Joint J 2021;103-B:18-25. [Crossref] [PubMed]
- Glaudemans AWJM, Jutte PC, Cataldo MA, Cassar-Pullicino V, Gheysens O, Borens O, Trampuz A, Wörtler K, Petrosillo N, Winkler H, Signore A, Sconfienza LM. Consensus document for the diagnosis of peripheral bone infection in adults: a joint paper by the EANM, EBJIS, and ESR (with ESCMID endorsement). Eur J Nucl Med Mol Imaging 2019;46:957-70. [Crossref] [PubMed]
- Lemmens L, Geelen H, Depypere M, De Munter P, Verhaegen F, Zimmerli W, Nijs S, Debeer P, Metsemakers WJ. Management of periprosthetic infection after reverse shoulder arthroplasty. J Shoulder Elbow Surg 2021;30:2514-22. [Crossref] [PubMed]
- Jevsevar DS, Abt E. The new AAOS-ADA clinical practice guideline on Prevention of Orthopaedic Implant Infection in Patients Undergoing Dental Procedures. J Am Acad Orthop Surg 2013;21:195-7. [Crossref] [PubMed]
- Parvizi J, Tan TL, Goswami K, Higuera C, Della Valle C, Chen AF, Shohat N. The 2018 Definition of Periprosthetic Hip and Knee Infection: An Evidence-Based and Validated Criteria. J Arthroplasty 2018;33:1309-1314.e2. [Crossref] [PubMed]
- Parvizi J, Zmistowski B, Berbari EF, Bauer TW, Springer BD, Della Valle CJ, Garvin KL, Mont MA, Wongworawat MD, Zalavras CG. New definition for periprosthetic joint infection: from the Workgroup of the Musculoskeletal Infection Society. Clin Orthop Relat Res 2011;469:2992-4. [Crossref] [PubMed]
- Govaert GAM, Glaudemans AWJM. Nuclear medicine imaging of posttraumatic osteomyelitis. Eur J Trauma Emerg Surg 2016;42:397-410. [Crossref] [PubMed]
- Erivan R, Villatte G, Eymond G, Mulliez A, Descamps S, Boisgard S. Usefulness of sonication for diagnosing infection in explanted orthopaedic implants. Orthop Traumatol Surg Res 2018;104:433-8. [Crossref] [PubMed]
- Murthy S, Fritz J. Metal Artifact Reduction MRI in the Diagnosis of Periprosthetic Hip Joint Infection. Radiology 2023;306:e220134. [Crossref] [PubMed]
- Lohmann CH, Rampal S, Lohrengel M, Singh G. Imaging in peri-prosthetic assessment: an orthopaedic perspective. EFORT Open Rev 2017;2:117-25. [Crossref] [PubMed]
- Verberne SJ, Temmerman OPP, Vuong BH, Raijmakers PG. Fluorodeoxyglucose positron emission tomography imaging for diagnosing periprosthetic hip infection: the importance of diagnostic criteria. Int Orthop 2018;42:2025-34. [Crossref] [PubMed]
- Anderson PA, Morgan SL, Krueger D, Zapalowski C, Tanner B, Jeray KJ, Krohn KD, Lane JP, Yeap SS, Shuhart CR, Shepherd J. Use of Bone Health Evaluation in Orthopedic Surgery: 2019 ISCD Official Position. J Clin Densitom 2019;22:517-43. [Crossref] [PubMed]
- Krappel FA, Harland U. Failure of osteosynthesis and prosthetic joint infection due to Mycobacterium tuberculosis following a subtrochanteric fracture: a case report and review of the literature. Arch Orthop Trauma Surg 2000;120:470-2. [Crossref] [PubMed]
- Liu Y, Liang H, Zhou X, Song W, Shao H, He Y, Yang Y, Guo L, Li P, Wei X, Duan W. Micro-Computed Tomography Analysis of Femoral Head Necrosis After Long-Term Internal Fixation for Femoral Neck Fracture. Orthop Surg 2022;14:1186-92. [Crossref] [PubMed]
- Shastri SK, Rudresh S, Anand R, Nagesh S, Seelamantula CS, Thittai AK. Axial super-resolution in ultrasound imaging with application to non-destructive evaluation. Ultrasonics 2020;108:106183. [Crossref] [PubMed]
- Rupp M, Bärtl S, Lang S, Walter N, Alt V. Fracture-related infections after intramedullary nailing : Diagnostics and treatment. Unfallchirurg 2022;125:50-8.
- Gibbon WW, Long G, Barron DA, O'Connor PJ. Complications of orthopedic implants: sonographic evaluation. J Clin Ultrasound 2002;30:288-99. [Crossref] [PubMed]
- Hall TAG, Cegla F, van Arkel RJ. Simple Smart Implants: Simultaneous Monitoring of Loosening and Temperature in Orthopaedics With an Embedded Ultrasound Transducer. IEEE Trans Biomed Circuits Syst 2021;15:102-10. [Crossref] [PubMed]
- Arvieux C, Common H. New diagnostic tools for prosthetic joint infection. Orthop Traumatol Surg Res 2019;105:S23-30. [Crossref] [PubMed]
- Long E, Maselli A, Barron S, Morgenstern M, Comer CD, Chow K, Cauley R, Lee BT. Applications of Ultrasound in the Postoperative Period: A Review. J Reconstr Microsurg 2022;38:245-53. [Crossref] [PubMed]
- Fernández-Fernández R, Oñorbe-San Francisco F, Gil-Garay E. Long-Term Outcomes of a Titanium-Encased Ceramic Liner Total Hip Arthroplasty (15 to 21Year Results). J Arthroplasty 2021;36:3697-702. [Crossref] [PubMed]
- Jiang Y, Wang SN, Wu HT, Qin HJ, Ren ML, Lin JC, Yu B. Aspirin alleviates orthopedic implant-associated infection. Int J Mol Med 2019;44:1281-8. [Crossref] [PubMed]
- Uzair U, Johnson C, Beladi-Behbahani S, Rajamanthrilage AC, Raval YS, Benza D, Ranasinghe M, Schober G, Tzeng TJ, Anker JN. Conformal Coating of Orthopedic Plates with X-ray Scintillators and pH Indicators for X-ray Excited Luminescence Chemical Imaging through Tissue. ACS Appl Mater Interfaces 2020;12:52343-53. [Crossref] [PubMed]
- Kingsbury SR, Smith LK, Czoski Murray CJ, Pinedo-Villanueva R, Judge A, West R, Smith C, Wright JM, Arden NK, Thomas CM, Kolovos S, Shuweihdi F, Garriga C, Bitanihirwe BKY, Hill K, Matu J, Stone M, Conaghan PG. Safety of disinvestment in mid- to late-term follow-up post primary hip and knee replacement: the UK SAFE evidence synthesis and recommendations. Southampton (UK): National Institute for Health and Care Research; 2022.
- Oka K, Shiode R, Yoshii Y, Tanaka H, Iwahashi T, Murase T. Artificial intelligence to diagnosis distal radius fracture using biplane plain X-rays. J Orthop Surg Res 2021;16:694. [Crossref] [PubMed]
- Lima AL, Oliveira PR, Carvalho VC, Saconi ES, Cabrita HB, Rodrigues MB. Periprosthetic joint infections. Interdiscip Perspect Infect Dis 2013;2013:542796. [Crossref] [PubMed]
- Ronin D, Boyer J, Alban N, Natoli RM, Johnson A, Kjellerup BV. Current and novel diagnostics for orthopedic implant biofilm infections: a review. APMIS 2022;130:59-81. [Crossref] [PubMed]
- Kohyama S, Yoshii Y, Okamoto Y, Nakajima T. Advances in Bone Joint Imaging-Metal Artifact Reduction. Diagnostics (Basel) 2022;12:3079. [Crossref] [PubMed]
- Cyteval C, Bourdon A. Imaging orthopedic implant infections. Diagn Interv Imaging 2012;93:547-57. [Crossref] [PubMed]
- Huang C, Chen Y, Ding H, Huang Z, Zhang C, Li W, Liu X, Tu Z, Zhang W, Fang X. Metal Artifact Reduction Sequences MRI: A Useful Reference for Preoperative Diagnosis and Debridement Planning of Periprosthetic Joint Infection. J Clin Med 2022;11:4371.
- Koff MF, Burge AJ, Koch KM, Potter HG. Imaging near orthopedic hardware. J Magn Reson Imaging 2017;46:24-39. [Crossref] [PubMed]
- Zanetti M. The Expanding Role of MRI in the Evaluation of Periprosthetic Hip Joint Infection. Radiology 2020;296:109-10. [Crossref] [PubMed]
- Meyers SP, Wiener SN. Magnetic resonance imaging features of fractures using the short tau inversion recovery (STIR) sequence: correlation with radiographic findings. Skeletal Radiol 1991;20:499-507. [Crossref] [PubMed]
- Shellock FG, Rosen MS, Webb A, Kimberly WT, Rajan S, Nacev AN, Crues JV. Managing Patients With Unlabeled Passive Implants on MR Systems Operating Below 1.5 T. J Magn Reson Imaging 2024;59:1514-22.
- Abel F, Tan ET, Chazen JL, Lebl DR, Sneag DB. MRI after Lumbar Spine Decompression and Fusion Surgery: Technical Considerations, Expected Findings, and Complications. Radiology 2023;308:e222732. [Crossref] [PubMed]
- Banerjee SR, Pomper MG. Clinical applications of Gallium-68. Appl Radiat Isot 2013;76:2-13.
- Salomäki SP, Kemppainen J, Hohenthal U, Luoto P, Eskola O, Nuutila P, Seppänen M, Pirilä L, Oksi J, Roivainen A. Head-to-Head Comparison of (68)Ga-Citrate and (18)F-FDG PET/CT for Detection of Infectious Foci in Patients with Staphylococcus aureus Bacteraemia. Contrast Media Mol Imaging 2017;2017:3179607.
- Tseng JR, Chang YH, Yang LY, Wu CT, Chen SY, Wan CH, Hsiao IT, Yen TC. Potential usefulness of 68Ga-citrate PET/CT in detecting infected lower limb prostheses. EJNMMI Res 2019;9:2. [Crossref] [PubMed]
- Kampen WU, Westphal F, Van den Wyngaert T, Strobel K, Kuwert T, Van der Bruggen W, Gnanasegaran G, Jens JH, Paycha F. SPECT/CT in Postoperative Foot and Ankle Pain. Semin Nucl Med 2018;48:454-68. [Crossref] [PubMed]
- Basu S, Kwee TC, Saboury B, Garino JP, Nelson CL, Zhuang H, Parsons M, Chen W, Kumar R, Salavati A, Werner TJ, Alavi A. FDG PET for diagnosing infection in hip and knee prostheses: prospective study in 221 prostheses and subgroup comparison with combined (111)In-labeled leukocyte/(99m)Tc-sulfur colloid bone marrow imaging in 88 prostheses. Clin Nucl Med 2014;39:609-15.
- Pakos EE, Trikalinos TA, Fotopoulos AD, Ioannidis JP. Prosthesis infection: diagnosis after total joint arthroplasty with antigranulocyte scintigraphy with 99mTc-labeled monoclonal antibodies--a meta-analysis. Radiology 2007;242:101-8. [Crossref] [PubMed]
- Al Mohajer M, Darouiche RO. The expanding horizon of prosthetic joint infections. J Appl Biomater Funct Mater 2014;12:1-12. [Crossref] [PubMed]
- Signore A, Jamar F, Israel O, Buscombe J, Martin-Comin J, Lazzeri E. Clinical indications, image acquisition and data interpretation for white blood cells and anti-granulocyte monoclonal antibody scintigraphy: an EANM procedural guideline. Eur J Nucl Med Mol Imaging 2018;45:1816-31.
- Meller J, Liersch T, Oezerden MM, Sahlmann CO, Meller B. Targeting NCA-95 and other granulocyte antigens and receptors with radiolabeled monoclonal antibodies (Mabs). Q J Nucl Med Mol Imaging 2010;54:582-98.
- Wong KK, Piert M. Dynamic bone imaging with 99mTc-labeled diphosphonates and 18F-NaF: mechanisms and applications. J Nucl Med 2013;54:590-9. [Crossref] [PubMed]
- Nie L, Sun Z, Shan F, Li C, Ding X, Shen C. An artificial intelligence framework for the diagnosis of prosthetic joint infection based on (99m)Tc-MDP dynamic bone scintigraphy. Eur Radiol 2023;33:6794-803. [Crossref] [PubMed]
- Guo H, Xu C, Chen J. Risk factors for periprosthetic joint infection after primary artificial hip and knee joint replacements. J Infect Dev Ctries 2020;14:565-71. [Crossref] [PubMed]
- Palestro CJ, Kim CK, Swyer AJ, Capozzi JD, Solomon RW, Goldsmith SJ. Total-hip arthroplasty: periprosthetic indium-111-labeled leukocyte activity and complementary technetium-99m-sulfur colloid imaging in suspected infection. J Nucl Med 1990;31:1950-5.
- Kim C, Lee SJ, Kim JY, Hwang KT, Choi YY. Comparative Analysis of (99m)Tc-MDP Three-Phase Bone Scan with SPECT/CT and (99m)Tc-HMPAO-Labeled WBC SPECT/CT in the Differential Diagnosis of Clinically Suspicious Post-traumatic Osteomyelitis. Nucl Med Mol Imaging 2017;51:40-8.
- Noronha OP, Sewatkar AB. Comparison of three RE agents-99mTc-phytate, 99mTc-sulfur colloid and 99mTc-Sb2S3 colloid in the rodent species. Int J Rad Appl Instrum B 1986;13:67-73. [Crossref] [PubMed]
- Jung KP, Park JS, Lee AY, Choi SJ, Lee SM, Bae SK. The Clinical Usefulness of (99m)Tc HMPAO Leukocyte/(99m)Tc Phytate Bone Marrow Scintigraphy for Diagnosis of Prosthetic Knee Infection: A Preliminary Study. Nucl Med Mol Imaging 2012;46:247-53.
- Glaudemans AW, Signore A. FDG-PET/CT in infections: the imaging method of choice? Eur J Nucl Med Mol Imaging 2010;37:1986-91. [Crossref] [PubMed]
- Wang Y, Sun Z, Liang X, Shen C. Inguinal draining-lymph node in 18F-FDG PET/CT images could be a new indicator for the diagnosis of fracture-related infection in the lower extremities. Front Immunol 2023;14:1206682. [Crossref] [PubMed]
- Chatziioannou AF. PET scanners dedicated to molecular imaging of small animal models. Mol Imaging Biol 2002;4:47-63. [Crossref] [PubMed]
- Shemesh S, Kosashvili Y, Groshar D, Bernstine H, Sidon E, Cohen N, Luria T, Velkes S. The value of 18-FDG PET/CT in the diagnosis and management of implant-related infections of the tibia: a case series. Injury 2015;46:1377-82. [Crossref] [PubMed]
- Kumar R, Dhanpathi H, Basu S, Rubello D, Fanti S, Alavi A. Oncologic PET tracers beyond [(18)F]FDG and the novel quantitative approaches in PET imaging. Q J Nucl Med Mol Imaging 2008;52:50-65.
- Basu S, Kwee TC, Surti S, Akin EA, Yoo D, Alavi A. Fundamentals of PET and PET/CT imaging. Ann N Y Acad Sci 2011;1228:1-18. [Crossref] [PubMed]
- Yue B, Tang T. The use of nuclear imaging for the diagnosis of periprosthetic infection after knee and hip arthroplasties. Nucl Med Commun 2015;36:305-11. [Crossref] [PubMed]
- Aksoy SY, Asa S, Ozhan M, Ocak M, Sager MS, Erkan ME, Halac M, Kabasakal L, Sönmezoglu K, Kanmaz B. FDG and FDG-labelled leucocyte PET/CT in the imaging of prosthetic joint infection. Eur J Nucl Med Mol Imaging 2014;41:556-64. [Crossref] [PubMed]
- Rini JN, Bhargava KK, Tronco GG, Singer C, Caprioli R, Marwin SE, Richardson HL, Nichols KJ, Pugliese PV, Palestro CJ. PET with FDG-labeled leukocytes versus scintigraphy with 111In-oxine-labeled leukocytes for detection of infection. Radiology 2006;238:978-87. [Crossref] [PubMed]
- Palestro CJ, Love C, Miller TT. Diagnostic imaging tests and microbial infections. Cell Microbiol 2007;9:2323-33. [Crossref] [PubMed]
- Bhattacharya A, Kochhar R, Sharma S, Mittal BR. Infection of pancreatic pseudocyst demonstrated on PET/CT using 18F-fluorodeoxyglucose-labeled autologous leucocytes. Clin Nucl Med 2012;37:618-9. [Crossref] [PubMed]
- Lemans JVC, Hobbelink MGG, IJpma FFA, Plate JDJ, van den Kieboom J, Bosch P, Leenen LPH, Kruyt MC, Glaudemans AWJM, Govaert GAM. The diagnostic accuracy of (18)F-FDG PET/CT in diagnosing fracture-related infections. Eur J Nucl Med Mol Imaging 2019;46:999-1008.
- Govaert GA, IJpma FF, McNally M, McNally E, Reininga IH, Glaudemans AW. Accuracy of diagnostic imaging modalities for peripheral post-traumatic osteomyelitis - a systematic review of the recent literature. Eur J Nucl Med Mol Imaging 2017;44:1393-407.
- Zhang Q, Dong J, Shen Y, Yun C, Zhou D, Liu F. Comparative diagnostic accuracy of respective nuclear imaging for suspected fracture-related infection: a systematic review and Bayesian network meta-analysis. Arch Orthop Trauma Surg 2021;141:1115-30.