Evaluation of the SwiftScan mode in bone single-photon emission computed tomography (SPECT) imaging: effect on imaging quality and a semi-quantitative analysis
Introduction
Whole-body bone scintigraphy is currently one of the most commonly used examinations for detecting bone metastasis in the clinic, and it plays a crucial role in the clinical staging and treatment planning of patients with malignant tumors, especially those with a high prevalence of bone metastases, including breast, lung, prostate, and thyroid cancers, which account for 80% of bone metastasis (1). Following the detection of abnormal concentration foci through conventional bone plane scanning, single-photon emission computed tomography/computed tomography (SPECT/CT) imaging is often (but not always) performed. The incorporation of quantitative parameters, such as the standardized uptake value (SUV), provided by SPECT/CT enhances the accuracy of the quantitative identification and bolsters the reliability of the clinical diagnosis (2-4). Moreover, it facilitates the quantitative assessment of treatment response (5), offering a comparable objective basis. Bone scintigraphy is a widely available method, and plays a crucial role in bone metastasis detection in cancer patients, as it is inexpensive, and the method is simple. Thus, quantitative SPECT/CT may be helpful in detecting bone metastasis.
Currently, several advanced technologies have been integrated into SPECT/CT imaging, including attenuation correction based on CT data, scattering correction, and resolution recovery (6). Additionally, new acquisition methods have been developed to improve the image quality and quantitative accuracy of SPECT/CT (7). These advancements include the following three acquisition modes of SPECT: step-and-shoot (SS), continuous (continue), and step-and-shoot continuous (SSC). The latest SSC acquisition mode, which is known as SwiftScan (GE Healthcare, Tirat Hacarmel, Israel) and uses as the SPECT acquisition mode, has been introduced to SPECT imaging. In the traditional SS mode, projection data are collected only when the detector is stationary. Conversely, in the SSC mode, data are collected both when the detector is stationary and when it is in motion, moving from one position to the next. Research has shown that the SwiftScan SPECT acquisition mode significantly increases the photon count, reduces the acquisition time, and improves the quality of bone and lung tomographic images (8,9). Therefore, the SwiftScan SPECT acquisition mode has potential technical advantages in enhancing image quality and improving the quantitative accuracy of bone scintigraphy.
In this study, we aimed to investigate the effect of the SwiftScan acquisition mode on the quality of 99mTc-stannous methylene diphosphonate (99mTc-MDP) bone SPECT/CT images based on phantom and clinical patient data. We retrospectively compared the SwiftScan, SwiftScan-50%, and traditional SS modes of acquisition visually and semi-quantitatively. We present this article in accordance with the STROBE reporting checklist (available at https://qims.amegroups.com/article/view/10.21037/qims-24-627/rc).
Methods
Phantom preparation
The National Electrical Manufacturers Association (NEMA) International Electrotechnical Commission (IEC) phantom was used in the phantom study. The phantom has six spheres with diameters of 10, 13, 17, 22, 28, and 37 mm, respectively. The spheres were filled with 133.20 KBq/mL 99mTc-MDP at a sphere-to-background activity ratio of 10:1.
Phantom SPECT/CT imaging protocol
A SPECT/CT system (GE Discovery NM/CT 870, GE Healthcare, Tirat Hacarmel, Israel) equipped with a low-energy, high-resolution, and high-sensitivity collimator was used. The following parameters were used in the SPECT scan: energy peak: 140 keV ±10% energy window; matrix size: 128×128; zoom: 1.5; SwiftScan mode; scan speed: 5 s/frame, and 3°/360°. Next, the CT images were obtained using the following parameters: tube voltage: 140 kV; tube current: 300 mA; table speed: 8.75 mm/rot; slice thickness: 5 mm; pitch: 0.875:1; and matrix: 512×512. All the tomographic images were analyzed using the following three acquisition modes: SSC (SwiftScan); SS; and SS C-50%, which simulates a 50% reduction in the acquisition time by applying the Poisson re-sampling method to achieve a 50% reduction in counts (10,11).
All the SPECT tomographic images were reconstructed using the ordered subsets expectation maximization algorithm with four iterations/10 subsets. Additionally, attenuation correction (based on the CT data), scattering correction, and sensitivity recovery correction were performed. A post-filer (Butterworth with the frequency of 0.48 and an order of 10) was applied.
Clinical study subjects
A total of 69 patients who underwent 99mTc-MDP local bone SPECT/CT examination at the Department of Nuclear Medicine between June and December 2023 were retrospectively enrolled in the study (Figure 1). These patients underwent evaluations for confirmed or suspected bone metastasis. To be eligible for inclusion in this study, the patients had to meet the following inclusion criteria: (I) show the presence of focal radioactive accumulation on whole-body bone planar scans; and (II) have undergone local bone SPECT/CT scans. Patients who were unable to undergo image assessment or who had poor image quality were excluded from the study.
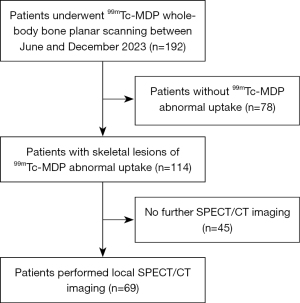
This study was conducted in accordance with the Declaration of Helsinki (as revised in 2013). The study was approved by the Ethics Committee of Central Hospital of Dalian University of Technology (approval No. YN2004-110-01), and all patients provided written informed consent.
Bone 99mTc-MDP SPECT/CT imaging
Each patient received an intravenous injection of 740–925 MBq of 99mTc-MDP. Whole-body bone planar scan and single field-of-view SPECT/CT imaging were performed 2–4 hours after the injection. Anterior and posterior views of planar whole-body scan (matrix: 256×1,024, and speed: 10 cm/min) were obtained in the supine position. SPECT/CT images were immediately acquired after the whole-body scan. The SPECT/CT image patient acquisition conditions and the reconstruction in the clinical study were the same as those in the phantom study.
Image analysis
Phantom image analysis
On the CT images, a spherical tool was used to outline a sphere along the inner wall of the hot sphere that matched the diameter of the sphere. The volumes of interest (VOIs) for the outlined target area were compared with the actual volumes of the corresponding hot spheres to ensure an error of <10%. The same VOIs were copied from the background area next to the hot spheres, and the radioactive concentration values (KBq/mL), average radioactive counts, and standard deviations (SDs) of the hot spheres and background were automatically measured. All the VOIs were drawn three times to evaluate the variations, and the results are presented as the mean ± SD. The coefficient of variation (COV) of the background and the signal-to-background ratio (SBR) of the spheres were determined. The COV was defined as the ratio of the background SD to the mean KBq/mL, while the SBR was defined as the maximum KBq/mL quotient of spheres to background mean KBq/mL.
Visual assessment of clinical study
The SPECT/CT images were double-blindly interpreted by two expert nuclear medicine radiologists, and a 5-point Likert scale (on which 1 point represented unacceptable image quality, 2 points represented unsatisfactory image quality, 3 points represented acceptable image quality, 4 points represented good image quality, and 5 points represented excellent image quality) was used for the visual evaluation based on the clarity, smoothness, and lesion detection performance of the images in the three acquisition modes (12).
Semi-quantitative analysis of clinical study
The VOIs of the 99mTc-MDP bone abnormal radioactive concentration foci in the SPECT images obtained using the three acquisition modes was automatically delineated using Q.VMI software (GE Healthcare), with a delineation threshold set at 40% SUVmax. Concurrently, the VOIs of equivalent volumes were outlined in the contralateral psoas major region (representing the normal background) to enable the quantification of the SUVmax and SUVmean within the lesion VOIs, as well as the calculation of the SUVmean and SD within the background VOIs. Subsequently, the COV and bone lesion SBR were determined. The COV was defined as the ratio of the background SD to the SUVmean, while the SBR was defined as the quotient of the lesion SUVmax to the background SUVmean.
Statistical analysis
The statistical analysis was conducted using SPSS 22.0 (IBM Co., New York, USA). The quantitative data with a normal distribution are expressed as the mean ± SD. While the quantitative data with a non-normal distribution are expressed as the median (P25, P75). A one-way analysis of variance test was employed in both the phantom and clinical studies, and the least significant difference test was applied for multiple comparisons. The intraclass correlation coefficient (ICC) was used to assess the consistency of the visual scores between the two expert nuclear medicine radiologists. The Chi-square test was used to evaluate differences in the visual scores. The Friedman test was used to assess differences in the semi-quantitative parameters COVs, SUVs, and SBRs in the clinical study. The receiver operating characteristics (ROC) curve and DeLong test were used to compare the diagnostic performance of malignant bone lesions between the different acquisition mode groups. A diagnosis of skeletal metastases was based on a pathologic or clinical diagnosis. A P value <0.05 (two-tailed) was considered statistically significant.
Results
Phantom results
The results of the phantom study are summarized in Figures 2,3. We observed that the quality of images obtained in the SwiftScan mode, including the spheres, display clarity, and shapes, was better than that of images obtained in the SwiftScan-50% and SS modes. The background noise of the SwiftScan and SwiftScan-50% modes was lower than the SS who had the highest COVs (P<0.05). For 37–13 mm spheres, the mean count of the SwiftScan mode was significantly higher than the mean counts of the SwiftScan-50% and SS modes (P<0.05). According to the corresponding semi-quantitative parameters analysis, the SwiftScan mode had the highest radioactive concentration and SBR, followed by the SwiftScan-50% mode, while the SS mode had the lowest concentration (P<0.05) for spheres of 37–17 mm.
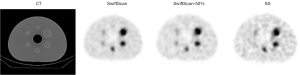
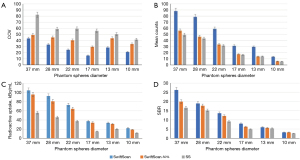
Patient characteristics
The differences in the actual acquisition time between the different acquisition modes are detailed in Table 1. In total, 69 patients were included in the study, of whom, 30 were male and 39 were female. The patients had a median age of 67 years (range, 18–82 years). Table 2 sets out the basic characteristics of all the patients. In total, the 69 patients had a total of 101 skeletal lesions, exhibiting abnormal 99mTc-MDP uptake. Among these skeletal lesions, 49 were benign (e.g., degenerative or fractures) and 52 were malignant (Table 1). A diagnosis of the skeletal metastasis was based on a pathologic or clinical diagnosis.
Table 1
Group | Mode | S/step (s) | Total time |
---|---|---|---|
SwiftScan | Step + move | 5 | 5 min + 3 min 03 s |
SwiftScan-50% | 1/2 step + move | 2.5 | 2 min 30 s + 3 min 03 s |
SS | Step | 5 | 5 min |
SPECT, single-photon emission computed tomography; SwiftScan, step-and-shoot continuous acquisition mode; SwiftScan-50%, simulates a 50% reduction in acquisition time; SS, step-and-shoot.
Table 2
Variable | Patients | Benign skeletal lesions | Malignant skeletal lesions |
---|---|---|---|
Total number of patients | 69 | 49 | 52 |
Gender | |||
Male | 30 | 24 | 21 |
Female | 39 | 25 | 31 |
Age | |||
>67 years | 27 | 19 | 30 |
≤67 years | 42 | 30 | 22 |
Primary tumor | |||
Breast cancer | 15 | 13 | 11 |
Lung cancer | 26 | 18 | 22 |
Prostate cancer | 14 | 9 | 10 |
Thyroid cancer | 8 | 7 | 3 |
Others | 6 | 2 | 6 |
Location of skeletal lesions | |||
Thoracic cage | 12 | 14 | 10 |
Spine bone | 50 | 22 | 28 |
Pelvic bone | 39 | 13 | 14 |
Visual assessment clinical results
The visual scoring of the bone SPECT/CT images in the three different acquisition modes by two expert nuclear medicine radiologists was highly consistent [ICC: 0.816, 95% confidence interval (CI): 0.769–0.853, P<0.001]. The average image quality scores for the SwiftScan, SwiftScan-50%, and SS modes were 4.37±0.62, 3.45±0.55, and 3.18±0.68, respectively (P<0.001). Additionally, there were significantly higher visual scores in the swiftscan-50% than SS (P<0.05). The proportions of scores ≥4 in the three modes were 83/101, 33/101, and 31/101, respectively (P<0.001). Notably, no significant difference in the proportion of scores ≥4 was observed between the SwiftScan-50% and SS modes (P=0.762) (Figure 4). However, there was a statistically significant difference in the proportion of scores of 3 to 4 between the two modes with values of 59/101 and 44/101, respectively (P<0.05).
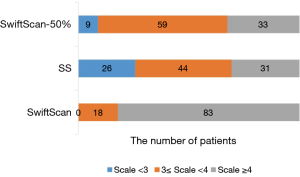
Semi-quantitative analysis clinical results
As Table 3 shows, the COV of the SwiftScan mode [38.46 (28.75, 47.58)] was significantly lower than that of the SwiftScan-50% mode [49.18 (37.25, 62.33)] and SS mode [53.85 (44.01, 66.67)] (P<0.001). Moreover, the COV of the SS mode was the highest (P<0.001). Similarly, in the clinical case SPECT/CT images, the image noise levels of the SwiftScan and SwiftScan-50% modes were better than those of the SS mode.
Table 3
Acquisition mode | Background SUVmean | Background SD | COV | Lesion SUVmax | Lesion SUVmean | Lesion SBR |
---|---|---|---|---|---|---|
SwiftScan | 0.45 (0.31, 0.63) | 0.16 (0.11, 0.23) | 38.46 (28.75, 47.58) | 15.80 (11.25, 25.15) | 9.26 (6.45, 14.00) | 37.50 (23.80, 78.07) |
SwiftScan-50% | 0.44 (0.29, 0.57) | 0.21 (0.14, 0.29) | 49.18 (37.25, 62.33) | 14.60 (10.80, 21.45) | 8.70 (6.18, 11.75) | 32.90 (21.09, 59.55) |
SS | 0.33 (0.23, 0.46) | 0.19 (0.12, 0.24) | 53.85 (44.01, 66.67) | 9.35 (6.23, 13.85) | 5.14 (3.34, 7.56) | 28.30 (18.72, 47.40) |
P value | <0.001 | <0.001 | <0.001 | <0.001 | <0.001 | <0.001 |
Data are expressed as the median (P25, P75). SBR is defined as lesion SUVmax/background SUVmean; COV is defined as background SD/SUVmean. SPECT, single-photon emission computed tomography; SUVmean, mean of the standard uptake value; SD, standard deviation; COV, coefficient of variation; SUVmax, maximum of the standard uptake value; SBR, signal-to-background ratio; SwiftScan, step-and-shoot continuous acquisition mode; SwiftScan-50%, simulates a 50% reduction in acquisition time; SS, step-and-shoot.
As Figure 5 and Table 3 show, the SUVmax, SUVmean, and SBR of the skeletal lesions were highest in the SwiftScan mode [15.8 (11.25, 25.15), 9.26 (6.45, 14.00), and 37.50 (23.80, 78.07), respectively], followed by the SwiftScan-50% mode [14.60 (10.80, 21.45), 8.70 (6.18, 11.75), and 32.90 (21.09, 59.55), respectively]. These values were all significantly higher than those of the SS mode [9.35 (6.23, 13.85), 5.14 (3.34, 7.56), and 28.30 (18.72, 47.40), respectively] (all P<0.001). Some typical case images are shown in Figure 6.
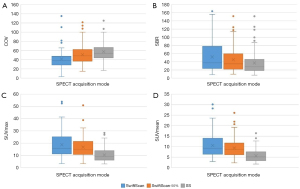
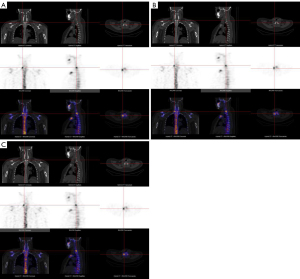
Diagnostic performance of different SPECT acquisition modes
Figure 7 shows the diagnostic efficacy of the SwiftScan, SwiftScan-50%, and SS modes in identifying malignant skeletal lesions using the SUVmax and SBR as variables. The areas under the curves for the SwiftScan, SwiftScan-50%, and SS modes were 0.92 (95% CI: 0.86–0.97), 0.911 (95% CI: 0.85–0.96) and 0.91(95% CI: 0.85–0.96) for SUVmax, and 0.82 (95% CI: 0.73–0.90), 0.825 (95% CI: 0.74–0.91) and 0.81 (95% CI: 0.73–0.90) for SBR, respectively. However there was no statically significant difference among the three groups in terms of the SUVmax (P=0.52 for SwiftScan vs. SwiftScan-50%, P=0.68 for SwiftScan vs. SS, P=0.96 for SwiftScan-50% vs. SS) or the SBR (P=0.87 for SwiftScan vs. SwiftScan-50%, P=0.81 for SwiftScan vs. SS, P=0.69 for SwiftScan-50% vs. SS). The optimal diagnostic cut-off values of the SUVmax for malignant skeletal lesions were 17.95 (sensitivity: 93.88%, specificity: 82.69%, and accuracy: 88.12%) for the SwiftScan mode, 14.75 (sensitivity: 85.71%, specificity: 82.69%, and accuracy: 84.16%) for the SwiftScan-50% mode, and 9.94 (sensitivity: 89.80%, specificity: 80.77%, and accuracy: 85.15%) for the SS mode. For the SBR, the optimal diagnostic cut-off values were 41.02 (sensitivity: 85.71%, specificity: 73.08%, and accuracy: 79.21%) for the SwiftScan mode, 38.22 (sensitivity: 87.76%, specificity: 71.15%, and accuracy: 79.21%) for the SwiftScan-50% mode, and 28.38 (sensitivity: 77.55%, specificity: 75.00%, and accuracy: 76.24%) for the SS mode.
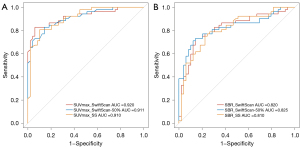
Discussion
Given the increasing clinical applications of SPECT/CT, the tracer injection activity and scanning time need to be reduced to improve patient compliance and reduce the radiation dose. The results of this study confirmed that the SwiftScan acquisition mode significantly improved image quality. Additionally, the image quality and semi-quantitative analysis of the short scan time SwiftScan-50% mode were comparable to those of the SS mode, but no change in diagnostic performance was observed.
Previous studies on different radiotracers have also supported the use of the SwiftScan acquisition mode, which has a reduced scanning time. For example, a study by Bailly et al. (7) on dopamine transporter [123I] FP-CIT (DaTSCAN) [Iodine-123-fluoropropyl (FP)-carbomethoxy-3β-(4-iodophenyltropane) (CIT)] showed that a 25% reduction in acquisition time using the SwiftScan mode did not compromise the image quality or semi-quantitative analysis. Similarly, Picone et al. (13) compared different acquisition modes for bone and lung perfusion SPECT, and found that image quality and semi-quantitative parameters (i.e., the COV) were similar between the SS and SSC-25% modes (with a 25% reduction in the examination time). This study verified the effect of the SwiftScan acquisition mode on improving the quality of phantom and bone SPECT images. Notably, the SwiftScan mode significantly improved the image scores of nearly half of the bone lesions (83 vs. 31 cases). The COVs of the phantom and clinical image backgrounds were significantly lower in SwiftScan mode, and the noise level of the images was significantly reduced (14). SwiftScan SPECT also includes blurring while the detector moves to the next acquisition position. Therefore, it is assumed that the COV of SwiftScan SPECT reflects the increase in count statistics, and the blurring effect resulting from the detector movement (14). Further attempts were made to compare the SwiftScan-50% scanning time reduction with that of the SS mode. The SwiftScan-50% mode significantly improved the proportion of image scores of 3–4 points (59 vs. 44 cases) without losing the COV of the SPECT image. The diagnostic information in the SPECT image cannot be improved in the SS mode; however, the SwiftScan mode may be able to reduce the bone SPECT acquisition time by 50% or reduce the injection activity, and may even provide clinical practice evidence for the quantitative analysis of dynamic SPECT/CT.
Currently, research on the effect of the SwiftScan mode on the SPECT semi-quantitative parameter SUV of lesions is limited. In a previous study (13), the SUVmean of 50 normal bone VOIs did not differ significantly between the SS and SwiftScan-25% groups with values of 6.8±3.3 (0.4–13.2) and 6.5±3.2 (0.2–12.8), respectively (P=0.6). However, this study revealed that the SwiftScan mode significantly improved the SUVmax and SUVmean of the SPECT/CT abnormal skeletal lesions. Further, even the SwiftScan-50% mode exhibited a higher SUVmax and SUVmean than the SS mode, resulting in a substantial improvement in the SBR of the lesions. This improvement can be primarily attributed to the increase in the radioactivity counts facilitated by SwiftScan mode, which was invalidated in our phantom study.
The primary objective of quantitative SPECT/CT is to generate tomographic images with voxel values representative of activity concentrations, which can be converted into SUVs. SUVs can be measured using a variety of metrics. The SUVmax is more commonly used to represent the absorption intensity of a certain feature, as it relies on a single pixel value, which makes it more susceptible to image noise. Conversely, the SUVmean, which can be defined through target area delineation, is more heavily influenced by the outline threshold. In our study, a 40% SUVmax threshold was employed, and a strong correlation with the SUVmax was found. The SUVmax is mainly affected by image quality and noise level (15), but is not affected by acquisition time (16). Compared with the SS mode, the SUVmax and SUVmean measured by the SwiftScan mode SPECT were notably higher, which important, as SUVs are very helpful in the evaluation of the treatment response and prognostic assessment. Moreover, the increase in the SUVmax of skeletal lesions contributes to an improved SBR, which in turn can increase doctors’ confidence in making differential diagnoses. However, the ROC curve analysis results did not show an enhancement in the diagnostic performance of malignant skeletal lesions by the SwiftScan mode. The lesions included in this study were all clearly observable abnormal uptake lesions on planar images. Further, SwiftScan SPECT differential diagnosis is not used to screen suspicious lesions in this study, so there was no data to prove the higher differential diagnostic performance in the discovery of additional occult skeletal lesions.
However, the effect of the SwiftScan mode on image quality depends on the chosen acquisition time. Shiiba et al. (14) used a skeletal phantom and reported that the SSC mode showed significantly better radioactive counting and image quality than the SS mode in a short scanning time (<7 min). Further, the results for the SSC mode were quantitatively similar or better than those of the SS mode regardless of the acquisition time. These findings indicate that the SSC mode is particularly effective at improving image quality and ensuring quantitative accuracy in a short scanning time. This may be due to the fact that the radioactive counts detected during the rotation time of the detectors is added to the SS mode, and thus when the SS setting time is short, the increase in the total radioactive counts is significantly higher than that when the SS setting time is long. Therefore, this study adopted a 5 s/step and 3°/frame acquisition time, and the total acquisition time was about 8 min (including 300 s step + 180 s move), which is equivalent to adding 60% of the acquisition count statistics based on the step mode, higher acquisition counts show more obvious advantages in image quality.
This study had a number of limitations. Consistent with published literature, this study used the Poisson re-sampling method to simulate a 50% count reduction (7,10,13); however, the effects of variations in acquisition time and radioactivity concentration levels were not explored. Further randomized controlled studies based on clinical practice need to be conducted to elucidate the effects of changes in acquisition time on image quality and quantification.
Conclusions
Our study showed that the SwiftScan acquisition mode significantly improved the quality of the bone SPECT images. It is possible to reduce the scanning time by 50% without affecting the image quality and the semi-quantitative analysis results, compared to those obtained using the traditional SS mode. These findings provide valuable insights that may inform the clinical use of the SwiftScan mode in the qualitative and quantitative diagnosis of bone SPECT imaging.
Acknowledgments
Funding: None.
Footnote
Reporting Checklist: The authors have completed the STROBE reporting checklist. Available at https://qims.amegroups.com/article/view/10.21037/qims-24-627/rc
Conflicts of Interest: All authors have completed the ICMJE uniform disclosure form (available at https://qims.amegroups.com/article/view/10.21037/qims-24-627/coif). The authors have no conflicts of interest to declare.
Ethical Statement: The authors are accountable for all aspects of the work in ensuring that questions related to the accuracy or integrity of any part of the work are appropriately investigated and resolved. This study was conducted in accordance with the Declaration of Helsinki (as revised in 2013). The study was approved by the Ethics Committee of Central Hospital of Dalian University of Technology (approval No. YN2004-110-01), and all patients provided written informed consent.
Open Access Statement: This is an Open Access article distributed in accordance with the Creative Commons Attribution-NonCommercial-NoDerivs 4.0 International License (CC BY-NC-ND 4.0), which permits the non-commercial replication and distribution of the article with the strict proviso that no changes or edits are made and the original work is properly cited (including links to both the formal publication through the relevant DOI and the license). See: https://creativecommons.org/licenses/by-nc-nd/4.0/.
References
- Van den Wyngaert T, Strobel K, Kampen WU, Kuwert T, van der Bruggen W, Mohan HK, Gnanasegaran G, Delgado-Bolton R, Weber WA, Beheshti M, Langsteger W, Giammarile F, Mottaghy FM, Paycha F, Bone EANMJoint Committee and the Oncology Committee. The EANM practice guidelines for bone scintigraphy. Eur J Nucl Med Mol Imaging 2016;43:1723-38. [Crossref] [PubMed]
- Tabotta F, Jreige M, Schaefer N, Becce F, Prior JO, Nicod Lalonde M. Quantitative bone SPECT/CT: high specificity for identification of prostate cancer bone metastases. BMC Musculoskelet Disord 2019;20:619. [Crossref] [PubMed]
- Gherghe M, Mutuleanu MD, Stanciu AE, Irimescu I, Lazar A, Bacinschi X, Anghel RM. Quantitative Analysis of SPECT-CT Data in Metastatic Breast Cancer Patients-The Clinical Significance. Cancers (Basel) 2022;14:273. [Crossref] [PubMed]
- Ikeda T, Kitajima K, Tsuchitani T, Takahashi Y, Hama Y, Kotura N. Effectiveness of quantitative bone SPECT/CT for bone metastasis diagnosis. Hell J Nucl Med 2022;25:253-9. [Crossref] [PubMed]
- Gherghe M, Mutuleanu MD, Stanciu AE, Irimescu I, Lazar AM, Toma RV, Trifanescu OG, Anghel RM. Quantitative Assessment of Treatment Response in Metastatic Breast Cancer Patients by SPECT-CT Bone Imaging-Getting Closer to PET-CT. Cancers (Basel) 2023;15:696. [Crossref] [PubMed]
- Ljungberg M, Pretorius PH. SPECT/CT: an update on technological developments and clinical applications. Br J Radiol 2018;91:20160402. [Crossref] [PubMed]
- Bailly M, Le Rouzic G, Metrard G, Ribeiro MJ. Faster Acquisition for Dopamine Transporter Imaging Using Swiftscan Step and Shoot Continuous SPECT Without Impairing Visual and Semiquantitative Analysis. Front Med (Lausanne) 2020;7:235. [Crossref] [PubMed]
- Thibault F, Bailly M, Le Rouzic G, Metrard G. Clinical evaluation of General Electric new Swiftscan solution in bone scintigraphy on NaI-camera: A head to head comparison with Siemens Symbia. PLoS One 2019;14:e0222490. [Crossref] [PubMed]
- Shibutani T, Onoguchi M, Yoneyama H, Konishi T, Nakajima K. Performance of SwiftScan planar and SPECT technology using low-energy high-resolution and sensitivity collimator compared with Siemens SPECT system. Nucl Med Commun 2021;42:732-7. [Crossref] [PubMed]
- Salkica N, Begic A, Zubovic S, Ceric S, Basic A, Sehic A, Julardzija F, Tinjak E. Impact of Reduced Acquisition Time on Bone Single-photon Emission Computed Tomography Images in Oncology Patients. Acta Inform Med 2022;30:36-40. [Crossref] [PubMed]
- Kim IH, Lee SJ, An YS, Choi SY, Yoon JK. Simulating dose reduction for myocardial perfusion SPECT using a Poisson resampling method. Nucl Med Mol Imaging 2021;55:245-52. [Crossref] [PubMed]
- Sibille L, Chambert B, Alonso S, Barrau C, D'Estanque E, Al Tabaa Y, Collombier L, Demattei C, Kotzki PO, Boudousq V. Impact of the Adaptive Statistical Iterative Reconstruction Technique on Radiation Dose and Image Quality in Bone SPECT/CT. J Nucl Med 2016;57:1091-5. [Crossref] [PubMed]
- Picone V, Makris N, Boutevin F, Roy S, Playe M, Soussan M. Clinical validation of time reduction strategy in continuous step-and-shoot mode during SPECT acquisition. EJNMMI Phys 2021;8:10. [Crossref] [PubMed]
- Shiiba T, Sekikawa Y, Tateoka S, Shinohara N, Inoue Y, Kuroiwa Y, Tanaka T, Kihara Y, Imamura T. Verification of the effect of acquisition time for SwiftScan on quantitative bone single-photon emission computed tomography using an anthropomorphic phantom. EJNMMI Phys 2022;9:48. [Crossref] [PubMed]
- O'Connor MK, Morrow MMB, Hunt KN, Boughey JC, Wahner-Roedler DL, Conners AL, Rhodes DJ, Hruska CB. Comparison of Tc-99m maraciclatide and Tc-99m sestamibi molecular breast imaging in patients with suspected breast cancer. EJNMMI Res 2017;7:5. [Crossref] [PubMed]
- Strandberg S, Hashemi A, Axelsson J, Riklund K. Optimization of PET reconstruction algorithm, SUV thresholding algorithm and PET acquisition time in clinical 11C-acetate PET/CT. PLoS One 2018;13:e0209169. [Crossref] [PubMed]