Quantitative observation based on magnetic resonance imaging: the effects of marathon running on lumbar vertebrae, paraspinal muscles, and lumbar disc components
Introduction
Marathon running has flourished globally and become a highly popular sporting event. According to research, marathon running not only reduces the risk of arteriosclerosis but also decreases the likelihood of developing chronic cardiovascular diseases and mental health disorders (1,2). Despite its multifaceted benefits for the human body, marathon running may also increase the potential risk of lumbar spine injury, and can cause intervertebral disc (IVD) degeneration, herniated discs, and lumbar osteoporosis (3).
To gain a deeper understanding of the connection between marathon running and lumbar health, and to provide scientific guidance for athletes’ training plans and prevent lumbar injury, it is particularly important to quantitatively analyze the lumbar spines of marathon runners using magnetic resonance (MR). This study examined the specific effects of various influencing factors on the lumbar spine, including key indicators such as the IVD water content and proton density fat fraction (PDFF) of vertebral bodies and paraspinal muscles (PMs).
Lumbar injury and IVD degeneration are prevalent spinal health issues that profoundly affect people’s quality of life and efficiency at work. Notably, IVD degeneration is not limited to the elderly population, and there has been a gradual increase in its incidence in younger populations in recent years (4). The condition of the IVDs significantly affects spinal health and the incidence of low back pain, and excessive loads can accelerate degenerative changes in the discs (5). During marathon running, the lumbar IVDs endure tremendous compression and torsional forces, which may adversely affect the health of the discs. Further, fat infiltration in vertebral bodies and PMs is an independent and cumulative risk factor for lumbar degeneration (6,7). The perfusion of vertebral blood vessels is the primary pathway for nutrient delivery to the IVDs. The PMs are closely connected to the lumbar spine both spatially and functionally. As crucial muscle groups for maintaining trunk stability, they have a high degree of involvement in marathon running. However, current research on the effects of marathon running on PMs is limited.
With the aid of chemically shifted water-fat magnetic resonance imaging (MRI) technology, precise data on the biochemical components of bone marrow and skeletal muscle in the body can be non-invasively obtained. Among them, Q-Dixon technology employs a sagittal T*2-corrected 6-echo Dixon-volumetric interpolated breath-hold examination (VIBE) sequence. After T2 correction, water and fat images can be generated, and the fat fraction (FF) map can be automatically reconstructed (8). This technology can accurately measure the FF of bone marrow adipose tissue (BMAT) of vertebral bodies, and the PDFF in PMs (9,10). MRI technology has also been shown to be an effective means of measuring lumbar spine characteristics, including assessing morphological changes in the lumbar IVDs, such as the height and width, making it an essential tool for identifying, grading, and classifying the degree of IVD degeneration (11).
The primary objective of this study was to quantitatively observe the effects of marathon running on the lumbar spine structure using MRI technology. This study evaluated various aspects of quantitative MRI parameters such as lumbar vertebral density, PM mass, and lumbar IVD degeneration. Through this research, we hope to extend understandings of the effects of marathon running on lumbar health. Our findings may provide scientific guidance for athletes’ training plans and the effective prevention of lumbar injury. We present this article in accordance with the STROBE reporting checklist (available at https://qims.amegroups.com/article/view/10.21037/qims-24-1053/rc).
Methods
Study participants
This study was approved by the Ethics Committee of The Affiliated Hospital of Hangzhou Normal University (No. 2021[E2]-KS-040) and was conducted in accordance with the Declaration of Helsinki (as revised in 2013). Informed consent was obtained from all individual participants. From April 2021 to September 2021, 54 amateur marathon runners were randomly recruited from an amateur marathon runners club in Hangzhou as the marathon runner group. Amateur marathon runners were defined as those who had not undergone formal training and did not professionally engage in marathon running (12,13). Additionally, 30 healthy volunteers were recruited from the community as the control group. Both the marathon and control groups comprised males exclusively [to exclude the influence of sex hormones (14)]. All the participants were sedentary office knowledge workers. A questionnaire was used to collect data on the age, height, and weight of all participants.
To be eligible for inclusion in this study, the participants in the marathon runner group had to meet the following inclusion criteria: (I) be aged 20–55 years; (II) have no history of low back pain, discomfort, musculoskeletal symptoms, lumbar trauma or surgery, or lumbar disc herniation; (III) have no absolute contraindications for MRI; (IV) have more than 1 year of experience in amateur marathon running, have regularly engaged in long-distance running, and have a running frequency of more than 3 times per week at a distance of more than 10 km per run, or a monthly running distance exceeding 100 km; (V) have previously participated in a formal full marathon race.
To be eligible for inclusion in this study, the participants in the control group had to meet the following inclusion criteria: (I) be aged 20–55 years; (II) have no history of low back pain, discomfort, musculoskeletal symptoms, or lumbar trauma or surgery; and (III) have never participated in marathon sports, and have a weekly exercise (including but not limited to running) duration of less than 150 minutes in the past 3 years.
Participants were excluded from the study if they met any of the following exclusion criteria: (I) had lumbar trauma pain or lumbar disc herniation; (II) had contraindications for MRI scanning; (III) had images of suboptimal quality; (IV) have interference factors such as obesity, hyperlipidemia, alcoholism, and/or have history of the long-term use of drugs affecting bone metabolism (e.g., glucocorticoids and immunosuppressive drugs) (14,15).
MRI procedures
MRI was performed using a 1.5 Tesla scanner (MAGNETOM Aera, Siemens Healthineers, Erlangen, Germany) equipped with an 8-channel body phased-array coil and image acquisition system. Participants were positioned in a supine position, head first, maintaining the normal physiological curvature of the lumbar spine. All MRI scans were conducted after 6:00 pm to minimize the influence of diurnal variations in IVD water content.
Initially, axial and sagittal T2-weighted MRI images were acquired for lumbar spine localization, using the following scanning parameters for the: (I) T2WI fat-sat sequence in the sagittal plane: repetition time (TR): 2,790 ms; time to echo (TE): 818 ms; slice thickness: 4 mm; slice gap: 0.2 mm; field of view (FOV): 320 mm × 320 mm; and flip angle: 150°; and (II) conventional T2WI sequence in the axial plane: TR: 4,510 ms; TE: 100 ms; slice thickness: 5.0 mm; slice gap: 1.0 mm; and FOV: 200 mm × 200 mm. Additionally, the PDFF of the lumbar vertebrae and PMs were measured using the DIXON-VIBE sequence with the following parameters: for vertebrae in the sagittal plane: TR: 15.73 ms; TE: 2.38/4.76/7.14/9.52/11.90/14.28 ms; slice thickness: 3 mm; slice gap: 1.5 mm; FOV: 280 mm × 280 mm; and flip angle: 4°; and for the PMs in the axial plane: TR: 15.60 ms, TE: 2.38/4.76/7.14/9.52/11.90/14.28 ms, slice thickness: 5.0 mm; slice gap: 1 mm; FOV: 420 mm × 420 mm; and flip angle: 4°. Finally, the T2* times of the lumbar IVDs were measured using a sagittal T2* mapping sequence using the following scanning parameters: TR: 454 ms; TE: 4.35/11.83/19.31/26.79/34.27 ms; slice thickness: 4 mm; slice gap: 0 mm; FOV: 280 mm × 280 mm; flip angle: 25°.
Image analysis
The scanned image data of the sequences were uploaded to the post-processing workstation (Syngo MMWP, E11), the vertebral bodies, IVDs, and PMs are manually segmented. The PMs examined included the psoas major, multifidus, and erector spinae. Given the anatomical proximity and functional similarity between the multifidus and erector spinae, these two muscles were combined into one group for further investigation in this study. Using the sagittal T2* mapping sequence, multiple columns of images with different echo times were obtained and reconstructed by exponential fitting using the post-processing workstation, and pseudo-color maps were then obtained. For the delineation of the lumbar vertebral body FF, the BMFF sequence’s midline sagittal plane was selected to manually draw the region of interest (ROI), avoiding the cortical bone and vertebral basal venous foramina, and measuring the vertebral body BMFF value (Figure 1). When delineating the IVD ROI, we correlated the T2 mapping anatomical images with the T2 mapping pseudo-color images on the post-processing workstation. On the sagittal maximum intensity projection image, we subdivided the IVD into five approximately equidistant ROIs. Specifically, the annulus fibrosus (AF) was subdivided into the anterior annulus fibrosus (AAF) and posterior annulus fibrosus (PAF), while the nucleus pulposus (NP) was further divided into the anterior nucleus pulposus (ANP), central nucleus pulposus (CNP), and posterior nucleus pulposus (PNP) regions. This allowed us to obtain the T2* time for each ROI of each IVD sub-region (Figure 2).
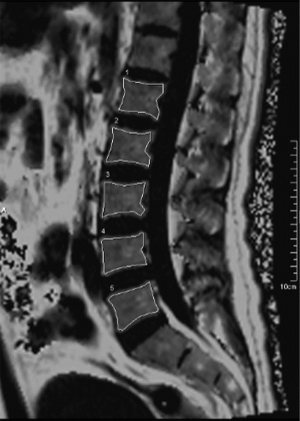
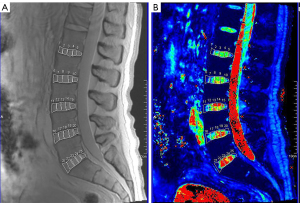
Based on the sagittal T2WI fat-sat sequence localization line, the middle level of the corresponding vertebral body was located, and manual segmentation was used to delineate the muscle areas of the bilateral psoas major and erector spinae at the L2, L3, L4, and L5 levels on the axial slices. We did not include the L1 vertebral level in the segmentation because of the incomplete display of the structures at the attachment of the superior border of the psoas major muscle at the L1 level in all participants. During the delineation process, special attention was paid to avoid confusion with subcutaneous fat and the boundary between muscle and fat. After determining the ROI, the cross-sectional area (CSA) of these muscles was measured and recorded using Syngo MMWP, and the average value of both sides was taken, for which the unit of measurement was square centimeters (cm2) (Figure 3).
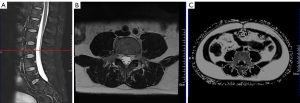
Reliability analysis
The lumbar vertebral body, IVDs, and bilateral PM ROI areas were outlined independently by two radiologists for the musculoskeletal diagnosis. An inter-rater reliability analysis was conducted to assess the consistency between the two readers. To ensure reliability, the ROIs were all measured independently, in a double-blind situation, at different times. And both radiologists used Syngo MMWP to manually outline the ROIs.
Statistical analysis
The data were analyzed using SPSS 26.0 software (IBM Corp., Armonk, NY, USA). Inter-observer agreement was assessed using the intra-class correlation coefficient (ICC) with a 95% confidence interval (CI). The Shapiro-Wilk test was used for all statistics with a sample size of less than 50, and the Kolmogorov-Smirnov test was used for all statistics with a sample size of more than 50. The normally distributed quantitative data are expressed as the mean ± standard deviation (), and the non-normally distributed quantitative data are expressed as the median (first quartile, third quartile). The disc T2 relaxation time, PM CSA, and FF of the PMs and vertebral bodies were compared between groups using a two-way analysis of variance, or generalized linear mixed models (for data that did not meet the normality and homogeneity of variance criteria). Pairwise comparisons were performed using the Bonferroni correction. A Spearman correlation analysis was conducted to evaluate the correlations between the effects of exercise intensity, IVD T2 time, lumbar vertebral body FF, paravertebral muscle FF and CSA. Correlations were classified as follows: r >0.6: extremely strong; r =0.6–0.4: moderate; r<0.4 weak/no correlation. A P value of <0.05 was considered statistically significant.
Results
Participants
In total, 54 amateur marathon runners and 30 healthy participants were enrolled in this study. The general characteristics of the two groups were compared, and there were no statistically significant differences between the marathon runner group and control group in terms of age, height, body mass, and body mass index (all P>0.05) (Table 1).
Table 1
Characteristics | Amateur marathon runners (n=54) | Healthy volunteers (n=30) | P value |
---|---|---|---|
Age (years) | 41.7±7.2 | 37.7±5.3 | 0.198 |
Height (cm) | 171.8±4.6 | 173.0±4.6 | 0.241 |
Weight (kg) | 68.6±7.2 | 70.7±4.9 | 0.171 |
BMI (kg/m2) | 23.3±2.1 | 23.6±1.2 | 0.344 |
Daily sedentary time (hour) | 5.3±2.2 | 4.9±1.8 | 0.461 |
Running history (years) | 4.7±3.1 | – | – |
The monthly running distance (km) | 172.6±76.4 | – | – |
The data are expressed as the mean ± standard deviation. BMI, body mass index.
The effect of marathon running on the vertebral body FF
Statistically significant differences were observed between the marathon runner group and control group in terms of the vertebral body FF at the L1, L2, L3, L4, and L5 vertebrae (all P<0.05). Specifically, the vertebral body FF of the marathon runners was significantly lower than that of the control group (Table 2).
Table 2
Lumber vertebra | Marathon runner group PDFF | Control group PDFF | P value |
---|---|---|---|
L1 | 33.44±5.19 | 36.60±5.66 | 0.016* |
L2 | 34.54±5.48 | 38.39±6.07 | 0.003* |
L3 | 36.62±5.80 | 40.26±5.91 | 0.006* |
L4 | 37.37±5.96 | 41.00±5.73 | 0.006* |
L5 | 37.99±6.07 | 41.82±6.05 | 0.004* |
*, P<0.05. PDFF, proton density fat fraction.
The effect of marathon running on the T2 relaxation times of IVDs
An analysis of the entire segment, including the AF and NP, was conducted. Compared to the control group, the marathon runner group had significantly increased mean T2 relaxation times in all segments of the AF (22.26±4.95 vs. 20.53±4.16 ms; P=0.021) and the NP (48.32±14.70 vs. 44.88±14.45 ms; P=0.018). When analyzing the different segments of the AF and NP, statistically significant differences were observed in the marathon runner group compared to the control group in the L3/4 AF region (21.95±4.53 vs. 19.60±3.30 ms; P=0.027), and the L4/5 NP region (51.80±15.95 vs. 44.36±15.50 ms; P=0.022). An analysis of the various sub-regions of the IVDs showed that there were also significant differences between the marathon runner group and the control group in terms of the T2 times in the ANP (41.28±14.36 vs. 38.04±13.12 ms; P=0.008), CNP (54.67±17.85 vs. 50.24±17.46 ms; P=0.003), and PAF (25.80±7.67 vs. 22.63±6.88 ms; P<0.001) (Figure 4).

The effect of marathon running on the CSA of PMs
The CSA of the psoas major muscle was more reduced at the L3 (8.25±2.17 vs. 9.70±1.81 cm2, P=0.002), L4 (11.82±2.65 vs. 13.62±1.97 cm2, P<0.001), and L5 (12.59±2.47 vs. 14.25±2.38 cm2, P<0.001) levels in the marathon runner group than the control group. The marathon runner group also had a smaller erector spinae CSA than the control group at the L2 (16.59±4.87 vs. 20.13±3.71 cm2, P<0.001) and L3 (18.04±4.63 vs. 21.65±3.38 cm2, P<0.001) levels (Figure 5).
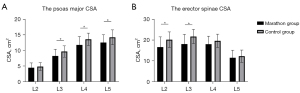
The effect of marathon running on the FF of PMs
The PDFF of the psoas major muscle at the L2, L3, L4, and L5 levels was significantly lower in the marathon runner group than the control group (P<0.001). There was no significant difference in the PDFF of the erector spinae muscle between the two groups (Table 3).
Table 3
Lumbar level | Psoas major PDFF | Erector spinae PDFF | |||||
---|---|---|---|---|---|---|---|
Marathon runner group | Control group | P value | Marathon runner group | Control group | P value | ||
L2 | 4.84 (3.68, 6.31) | 6.83 (5.72, 9.09) | <0.001* | 3.83 (3.08, 5.08) | 4.36 (3.71, 5.63) | 0.269 | |
L3 | 3.91 (3.23, 4.97) | 6.76 (5.33, 7.72) | <0.001* | 4.75 (3.87, 6.26) | 5.71 (4.72, 6.75) | 0.110 | |
L4 | 4.26 (3.16, 5.00) | 6.11 (5.45, 6.92) | <0.001* | 5.58 (4.49, 7.05) | 6.56 (5.63, 7.75) | 0.214 | |
L5 | 4.35 (3.50, 5.64) | 6.67 (5.62, 8.58) | <0.001* | 8.67 (6.72, 10.82) | 10.07 (8.17, 11.04) | 0.052 |
*, P<0.05. PDFF, proton density fat fraction.
Correlations
The AF was weakly negatively correlated with the monthly running distance at the L4/5 (r=–0.342, P=0.011) and L5/S1 (r=–0.304, P=0.026) levels. The PDFF of the psoas major muscle was weakly and negatively correlated with the monthly running distance at the L3 level (r=–0.396, P=0.003) (Figure 6).
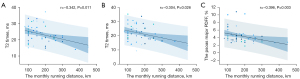
Reproducibility measurement
In the quantitative ROI assessment using MRI, the ICCs and 95% CIs for the lumbar vertebral PDFF, IVD T2 time, PM CSA, and PM PDFF were 0.977 (0.942, 0.989), 0.848 (0.810, 0.880), 0.939 (0.876, 0.969), and 0.833 (0.698, 0.910), respectively. These results indicate good reliability among observers.
Discussion
This study conducted a comparative analysis of the differences in MRI signals of lumbar vertebrae and paravertebral muscles between an amateur marathon runner group and a control group. The participants were all sedentary office knowledge workers. None of the participants undertook physical labor in their work, and the participants in the marathon runner group did not engage in any strength training other than that related to their usual running habits. The results showed that the marathon runner group had a smaller PM CSA and lower fat content in the psoas major muscle and lumbar vertebrae than the control group. Notably, the T2 times of the IVDs, especially the ANP, CNP and PAF, were significantly higher in the amateur marathon runner group than the control group, which suggests that marathon running has a positive effect on the psoas major muscles, IVDs, and vertebrae.
In relation to the vertebral fat content, we found that the fat content of each lumbar vertebra of the amateur marathon runners was lower than that of the healthy participants.
BMAT only accounts for about 10% of total body fat; however, t this varies widely among individuals (16). Physiologically, the human body is primarily composed of red bone marrow at birth, but as humans age, the red bone marrow gradually transforms into yellow bone marrow, which eventually accounts for up to 70% of all bone marrow tissue in adulthood (17). However, under pathological conditions, such as osteoporosis, aging, type 2 diabetes, and radiotherapy, the content of BMAT tends to increase further (18-21). Study (22) has shown that there may be an inverse relationship between BMAT and bone mineral density, which could be related to the pathogenesis of osteoporosis.
Compared to those engaged in weight-bearing exercises, individuals who perform non-weight-bearing exercises (e.g., cycling and swimming) have a lower bone mass (23-25). Research has shown that participants who engaged in just two hours of physical activity per week had a lower level of BMAT FF than those in the control group, and this effect was more pronounced in spinal BMAT (26). Repeated mechanical loads on the spine (27), such as those experienced during activities like playing soccer, can result in a lower bone marrow FF and higher bone mineral density. This effect is more evident in weight-bearing areas like the lumbar spine and lower limbs. The present study further confirmed that marathon running, as a sustained and moderately intense aerobic weight-bearing exercise, can effectively reduce the proportion of BMAT. This type of exercise also facilitates the transport of newly formed red blood cells from the bone marrow into the bloodstream, meeting the body’s demand for oxygen during exercise. This supports the findings of Belavy et al. (28), who reported that the vertebral FF was more reduced in a long-distance runner group than a control group. Additionally, similar results were observed in a prospective study conducted on patients with non-specific low back pain, where a 6-month exercise intervention (28) led to a decrease in lumbar BMAT, particularly among males.
In relation to changes in the IVDs, previous studies have investigated alterations in IVDs after marathon running using qualitative methods (29). Conversely, the present study employed a more precise quantitative T2 mapping technique. The application of this technology enabled us to detect early biochemical changes in IVDs that are difficult to discern using the Pfirrmann grading system, providing a more objective evaluation (30). Our study found that the increase in T2 times was more significant in the ANP, CNP, and PAF of the amateur marathon runners than the healthy participants.
The IVD consists of an AF and an inner NP. From the outside to the inside, the water and glycosaminoglycan content gradually increases, while type-I collagen gradually transforms into type-II collagen. During IVD degeneration, the water content decreases, and the collagen tissue transitions from a more randomly oriented network structure to a more uniform structure. Microstructural elements are lost, and the polarity of the NP collagen increases (31). Previous animal models have revealed a strong correlation between the T2 relaxation time and proteoglycan levels, type-II collagen content, and histological degeneration scores (32,33). Dynamic compressive loads during exercise alter the specific expression of key matrix genes and catabolic genes in the IVDs, depending on the frequency and magnitude of the stimulus (34). Marathon running can promote the hydration and glycosaminoglycan levels of the IVDs, thereby helping to delay the degenerative process. Additionally, similar to previous studies (35), we found variations in the response of IVDs to mechanical stimuli in different anatomical regions.
Previous review on the effects of exercise on IVDs indicate that while dynamic axial loads experienced during slow- to-moderate movements contribute to positive adaptive adjustments in the discs, high-impact loads, explosive movements, and extreme exercises pose a potential threat to their health (3). Our study found a mild negative correlation between the monthly running volume and the AF of the L4/L5 and L5/S1 segments, and that excessive training intensity can also lead to disc damage. Previous research has shown that sports like basketball and volleyball can also benefit the adaptation of the IVDs (36). Specific types of exercise elicit positive physiological responses in the discs, and our study supports the view that marathon running can also lead to beneficial adaptations in the IVDs. Currently, studies are exploring whether therapeutic exercise training can improve the disc health of adults with chronic low back pain (37).
Our study also found a corresponding reduction in the muscle CSA of the PMs of the amateur marathon runners. The immediate energy source during muscle contraction is adenosine triphosphate (ATP), which is primarily obtained through the aerobic metabolism of glucose. When aerobic metabolism becomes insufficient, anaerobic metabolism occurs, consuming muscle glycogen and leading to muscle catabolism (38). Meanwhile, insufficient energy intake during running can lead to the activation of the adenosine 5'-monophosphate-activated protein kinase (AMPK) pathway, which in turn inhibits the mammalian target of rapamycin (mTOR) pathway involved in muscle cell synthesis (39,40).
Studies have shown that moderate-to-high intensity aerobic exercise results in a moderate decrease in muscle strength, especially after >30 minutes of aerobic training (41). Research has also shown that training strength and endurance simultaneously interferes with the development of strength, even at low intensities, compared to resistance training alone (42,43). In a cross-sectional study by Ferreira et al. (44), resistance exercise was shown to be effective in the control of sarcopenia, but no reduction in the prevalence of sarcopenia in the aerobic and sedentary groups was observed.
In the present study, the amateur marathon runners did not perform resistance strength training in addition to marathon running, which might have contributed to the reduction in the muscle CSA. In addition, we also found a reduction in the psoas major FF at various levels, and a slight negative correlation between the average psoas major FF at the L3 level and the monthly running distance, and the reduction of psoas major FF is beneficial to muscle strength (45). No reduction in the FF was found in the erector spinae muscles. This might be because the erector spinae muscles are more susceptible to fat infiltration (46). Conversely, the PMs play different roles in running; the psoas major and iliopsoas form the main muscles for hip flexion, and their involvement is crucial for rapid leg lifting and acceleration during running (47), while the erector spinae muscles mainly play a role in maintaining posture and upper body stabilization (48). However, the failure of the FF in the erector muscles to decrease could also be related to the reciprocal action of the psoas major and erector spinae muscle (49). As the CSA of the PMs decreases, the psoas major muscle exerts a compensatory mechanism to reduce the FF and stabilize the lumbar spine (50). Research has also revealed that the finding that high-intensity resistance training exclusively impacts intermuscular fat in the thigh muscles while sparing the erector spinae and psoas major might be attributed to the subjects’ older age and elevated baseline of intermuscular adipose tissue, resulting in a diminished muscle response to exercise (51).
Despite revealing the positive effect of marathon running on the lumbar spine and PMs, our study had some limitations. First, as a cross-sectional study, we cannot directly determine the causal relationship between marathon running and changes in the lumbar spine structure. To more accurately assess the effect of exercise on lumbar spine health, larger prospective studies need to be conducted to validate the current findings. Second, additional exercise types, intensities, and durations during training sessions among amateur athletes might have influenced the research outcomes. In future studies, we need to record and analyze these factors in more detail to control for any potential interference.
Prolonged high-intensity interval training leads to the hypertrophy of type-II muscle fibers, which is more conducive to the development of lower limb strength. Moderate-intensity continuous training recruits more type-I muscle fibers (52,53). Future studies should seek to explore whether the reduction in the muscle CSA we observed during marathon running was due to a reduction in type-I fibers or type-II fibers. Further, future research should also consider other factors that may affect the lumbar spine structure, such as age, gender, exercise intensity, and frequency. This will provide a more comprehensive understanding of the effect of exercise on lumbar spine health. By considering these factors, we can more accurately assess the lumbar spine health risks of different populations during marathon running and develop personalized exercise programs.
Conclusions
Our study showed that marathon running has a positive effect on the lumbar spine and psoas major muscles. By reducing vertebral fat content, improving disc hydration, and enhancing psoas major muscle strength, marathon running may offer an effective means for preventing and adjuvantly treating spinal health issues, such as lumbar degeneration. However, as muscle loss may occur during over-intense endurance running, resistance training and carbohydrate supplementation are essential. These findings have significant clinical implications for guiding the public on how to maintain spinal health through exercise. However, this study had a number of limitations, and relevant variables need to be further explored and controlled in future research to more accurately assess the effect of marathon running on lumbar spine health. Through continuous and in-depth research, we will be able to provide the public with more scientific and personalized exercise recommendations to promote the comprehensive development of spinal health.
Acknowledgments
Funding: This study was supported by
Footnote
Reporting Checklist: The authors have completed the STROBE reporting checklist. Available at https://qims.amegroups.com/article/view/10.21037/qims-24-1053/rc
Conflicts of Interest: All authors have completed the ICMJE uniform disclosure form (available at https://qims.amegroups.com/article/view/10.21037/qims-24-1053/coif). The authors have no conflicts of interest to declare.
Ethical Statement: The authors are accountable for all aspects of the work in ensuring that questions related to the accuracy or integrity of any part of the work are appropriately investigated and resolved. This study was conducted in accordance with the Declaration of Helsinki (as revised in 2013) and was approved by the Ethics Committee of The Affiliated Hospital of Hangzhou Normal University (No. 2021[E2]-KS-040). Informed consent was obtained from all individual participants.
Open Access Statement: This is an Open Access article distributed in accordance with the Creative Commons Attribution-NonCommercial-NoDerivs 4.0 International License (CC BY-NC-ND 4.0), which permits the non-commercial replication and distribution of the article with the strict proviso that no changes or edits are made and the original work is properly cited (including links to both the formal publication through the relevant DOI and the license). See: https://creativecommons.org/licenses/by-nc-nd/4.0/.
References
- Bhuva AN, D'Silva A, Torlasco C, Jones S, Nadarajan N, Van Zalen J, Chaturvedi N, Lloyd G, Sharma S, Moon JC, Hughes AD, Manisty CH. Training for a First-Time Marathon Reverses Age-Related Aortic Stiffening. J Am Coll Cardiol 2020;75:60-71. [Crossref] [PubMed]
- Wirnitzer K, Boldt P, Wirnitzer G, Leitzmann C, Tanous D, Motevalli M, Rosemann T, Knechtle B. Health status of recreational runners over 10-km up to ultra-marathon distance based on data of the NURMI Study Step 2. Sci Rep 2022;12:10295. [Crossref] [PubMed]
- Belavý DL, Albracht K, Bruggemann GP, Vergroesen PP, van Dieën JH. Can Exercise Positively Influence the Intervertebral Disc? Sports Med 2016;46:473-85. [Crossref] [PubMed]
- Ambrosio L, Mazzuca G, Maguolo A, Russo F, Cannata F, Vadalà G, Maffeis C, Papalia R, Denaro V. The burden of low back pain in children and adolescents with overweight and obesity: from pathophysiology to prevention and treatment strategies. Ther Adv Musculoskelet Dis 2023;15:1759720X231188831.
- Iatridis JC, MacLean JJ, Roughley PJ, Alini M. Effects of mechanical loading on intervertebral disc metabolism in vivo. J Bone Joint Surg Am 2006;88:41-6. [Crossref] [PubMed]
- Krug R, Joseph GB, Han M, Fields A, Cheung J, Mundada M, Bailey J, Rochette A, Ballatori A, McCulloch CE, McCormick Z, O'Neill C, Link TM, Lotz J. Associations between vertebral body fat fraction and intervertebral disc biochemical composition as assessed by quantitative MRI. J Magn Reson Imaging 2019;50:1219-26. [Crossref] [PubMed]
- Jung M, Rospleszcz S, Löffler MT, Walter SS, Maurer E, Jungmann PM, Peters A, Nattenmüller J, Schlett CL, Bamberg F, Kiefer LS, Diallo TD. Association of lumbar vertebral bone marrow and paraspinal muscle fat composition with intervertebral disc degeneration: 3T quantitative MRI findings from the population-based KORA study. Eur Radiol 2023;33:1501-12. [Crossref] [PubMed]
- Li X, Xie Y, Lu R, Zhang Y, Li Q, Kober T, Hilbert T, Tao H, Chen S. Q-Dixon and GRAPPATINI T2 Mapping Parameters: A Whole Spinal Assessment of the Relationship Between Osteoporosis and Intervertebral Disc Degeneration. J Magn Reson Imaging 2022;55:1536-46. [Crossref] [PubMed]
- Karampinos DC, Yu H, Shimakawa A, Link TM, Majumdar S T. 1-corrected fat quantification using chemical shift-based water/fat separation: application to skeletal muscle. Magn Reson Med 2011;66:1312-26. [Crossref] [PubMed]
- Karampinos DC, Ruschke S, Dieckmeyer M, Diefenbach M, Franz D, Gersing AS, Krug R, Baum T. Quantitative MRI and spectroscopy of bone marrow. J Magn Reson Imaging 2018;47:332-53. [Crossref] [PubMed]
- Pfirrmann CW, Metzdorf A, Zanetti M, Hodler J, Boos N. Magnetic resonance classification of lumbar intervertebral disc degeneration. Spine (Phila Pa 1976) 2001;26:1873-8. [Crossref] [PubMed]
- Zhang Y, Shu D, Yao W, Ding J, Chen L, Lin X, Tian T, Liu J. MRI study of changes in knee bone marrow edema-like signal in asymptomatic amateur marathon runners before and after half-marathon running. Clin Imaging 2021;80:150-7. [Crossref] [PubMed]
- Cao H, Cui F, Yu H, Cui J, Ma F, Duan L. Normal bone marrow signal characteristics in whole-body diffusion-weighted images of amateur marathon runners. Quant Imaging Med Surg 2024;14:2321-33. [Crossref] [PubMed]
- Zhang Y, Zhou J, Zheng XZ, Ding JP, Shu DB, Chen LL, Lin XY, Tian T. Magnetic resonance imaging analysis on the effect of marathon on the dynamic changes of morphological characteristics of patellar tendon in amateur marathon runners after half marathon. Zhonghua Yi Xue Za Zhi 2022;102:636-41. [Crossref] [PubMed]
- Yao W, Zhang Y, Zhang L, Zhou J, Zhang Y, Zheng X, Ding J. MRI features of and factors related to ankle injuries in asymptomatic amateur marathon runners. Skeletal Radiol 2021;50:87-95. [Crossref] [PubMed]
- Beekman KM, Regenboog M, Nederveen AJ, Bravenboer N, den Heijer M, Bisschop PH, Hollak CE, Akkerman EM, Maas M. Gender- and Age-Associated Differences in Bone Marrow Adipose Tissue and Bone Marrow Fat Unsaturation Throughout the Skeleton, Quantified Using Chemical Shift Encoding-Based Water-Fat MRI. Front Endocrinol (Lausanne) 2022;13:815835. [Crossref] [PubMed]
- Lecka-Czernik B, Rosen CJ. Energy Excess, Glucose Utilization, and Skeletal Remodeling: New Insights. J Bone Miner Res 2015;30:1356-61. [Crossref] [PubMed]
- Whitney DG, Singh H, Miller F, Barbe MF, Slade JM, Pohlig RT, Modlesky CM. Cortical bone deficit and fat infiltration of bone marrow and skeletal muscle in ambulatory children with mild spastic cerebral palsy. Bone 2017;94:90-7. [Crossref] [PubMed]
- de Araújo IM, Salmon CE, Nahas AK, Nogueira-Barbosa MH, Elias J Jr, de Paula FJ. Marrow adipose tissue spectrum in obesity and type 2 diabetes mellitus. Eur J Endocrinol 2017;176:21-30. [Crossref] [PubMed]
- Bastos CM, Araújo IM, Nogueira-Barbosa MH, Salmon CEG, de Paula FJA, Troncon LEA. Reduced bone mass and preserved marrow adipose tissue in patients with inflammatory bowel diseases in long-term remission. Osteoporos Int 2017;28:2167-76. [Crossref] [PubMed]
- Suchacki KJ, Cawthorn WP. Molecular Interaction of Bone Marrow Adipose Tissue with Energy Metabolism. Curr Mol Biol Rep 2018;4:41-9. [Crossref] [PubMed]
- Shen W, Scherzer R, Gantz M, Chen J, Punyanitya M, Lewis CE, Grunfeld C. Relationship between MRI-measured bone marrow adipose tissue and hip and spine bone mineral density in African-American and Caucasian participants: the CARDIA study. J Clin Endocrinol Metab 2012;97:1337-46. [Crossref] [PubMed]
- Olmedillas H, González-Agüero A, Moreno LA, Casajus JA, Vicente-Rodríguez G. Cycling and bone health: a systematic review. BMC Med 2012;10:168. [Crossref] [PubMed]
- Rector RS, Rogers R, Ruebel M, Hinton PS. Participation in road cycling vs running is associated with lower bone mineral density in men. Metabolism 2008;57:226-32. [Crossref] [PubMed]
- Bezerra A, Freitas L, Maciel L, Fonseca H. Bone Tissue Responsiveness To Mechanical Loading-Possible Long-Term Implications of Swimming on Bone Health and Bone Development. Curr Osteoporos Rep 2022;20:453-68. [Crossref] [PubMed]
- Belavy DL, Quittner MJ, Ridgers ND, Shiekh A, Rantalainen T, Trudel G. Specific Modulation of Vertebral Marrow Adipose Tissue by Physical Activity. J Bone Miner Res 2018;33:651-7. [Crossref] [PubMed]
- Wang J, Yi P, Huang Y, Yu Q, Mei Y, Chen J, Feng Y, Zhang X. Quantitative evaluation of bone marrow fat content and unsaturated fatty index in young male soccer players using proton magnetic resonance spectroscopy (1H-MRS): a preliminary study. Quant Imaging Med Surg 2021;11:4275-86. [Crossref] [PubMed]
- Belavy DL, Miller CT, Owen PJ, Rantalainen T, Connell D, Hahne AJ, Ford JJ, Trudel G. Exercise may impact on lumbar vertebrae marrow adipose tissue: Randomised controlled trial. Bone 2022;157:116338. [Crossref] [PubMed]
- Horga LM, Henckel J, Fotiadou A, Di Laura A, Hirschmann AC, Lee R, Hart AJ. What happens to the lower lumbar spine after marathon running: a 3.0 T MRI study of 21 first-time marathoners. Skeletal Radiol 2022;51:971-80. [Crossref] [PubMed]
- Tamagawa S, Sakai D, Nojiri H, Sato M, Ishijima M, Watanabe M. Imaging Evaluation of Intervertebral Disc Degeneration and Painful Discs-Advances and Challenges in Quantitative MRI. Diagnostics (Basel) 2022.
- Zeldin L, Mosley GE, Laudier D, Gallate ZS, Gansau J, Hoy RC, Poeran J, Iatridis JC. Spatial mapping of collagen content and structure in human intervertebral disk degeneration. JOR Spine 2020;3:e1129. [Crossref] [PubMed]
- Ishikawa T, Watanabe A, Kamoda H, Miyagi M, Inoue G, Takahashi K, Ohtori S. Evaluation of Lumbar Intervertebral Disc Degeneration Using T1ρ and T2 Magnetic Resonance Imaging in a Rabbit Disc Injury Model. Asian Spine J 2018;12:317-24. [Crossref] [PubMed]
- Bouhsina N, Decante C, Hardel JB, Rouleau D, Abadie J, Hamel A, Le Visage C, Lesoeur J, Guicheux J, Clouet J, Fusellier M. Comparison of MRI T1, T2, and T2* mapping with histology for assessment of intervertebral disc degeneration in an ovine model. Sci Rep 2022;12:5398. [Crossref] [PubMed]
- Ching CT, Chow DH, Yao FY, Holmes AD. Changes in nuclear composition following cyclic compression of the intervertebral disc in an in vivo rat-tail model. Med Eng Phys 2004;26:587-94. [Crossref] [PubMed]
- Fearing BV, Hernandez PA, Setton LA, Chahine NO. Mechanotransduction and cell biomechanics of the intervertebral disc. JOR Spine 2018;1:e1026. [Crossref] [PubMed]
- Owen PJ, Hangai M, Kaneoka K, Rantalainen T, Belavy DL. Mechanical loading influences the lumbar intervertebral disc. A cross-sectional study in 308 athletes and 71 controls. J Orthop Res 2021;39:989-97. [Crossref] [PubMed]
- Tagliaferri SD, Belavy DL, Bowe SJ, Clarkson MJ, Connell D, Craige EA, Gollan R, Main LC, Miller CT, Mitchell UH, Mundell NL, Neason C, Samanna CL, Scott D, Tait JL, Vincent GE, Owen PJ. Assessing safety and treatment efficacy of running on intervertebral discs (ASTEROID) in adults with chronic low back pain: protocol for a randomised controlled trial. BMJ Open Sport Exerc Med 2023;9:e001524. [Crossref] [PubMed]
- Westerblad H, Bruton JD, Katz A. Skeletal muscle: energy metabolism, fiber types, fatigue and adaptability. Exp Cell Res 2010;316:3093-9. [Crossref] [PubMed]
- Zhang CS, Hawley SA, Zong Y, Li M, Wang Z, Gray A, Ma T, Cui J, Feng JW, Zhu M, Wu YQ, Li TY, Ye Z, Lin SY, Yin H, Piao HL, Hardie DG, Lin SC. Fructose-1,6-bisphosphate and aldolase mediate glucose sensing by AMPK. Nature 2017;548:112-6. [Crossref] [PubMed]
- Schiaffino S, Reggiani C, Akimoto T, Blaauw B. Molecular Mechanisms of Skeletal Muscle Hypertrophy. J Neuromuscul Dis 2021;8:169-83. [Crossref] [PubMed]
- Markov A, Chaabene H, Hauser L, Behm S, Bloch W, Puta C, Granacher U. Acute Effects of Aerobic Exercise on Muscle Strength and Power in Trained Male Individuals: A Systematic Review with Meta-analysis. Sports Med 2022;52:1385-98. [Crossref] [PubMed]
- Hickson RC. Interference of strength development by simultaneously training for strength and endurance. Eur J Appl Physiol Occup Physiol 1980;45:255-63. [Crossref] [PubMed]
- Häkkinen K, Alen M, Kraemer WJ, Gorostiaga E, Izquierdo M, Rusko H, Mikkola J, Häkkinen A, Valkeinen H, Kaarakainen E, Romu S, Erola V, Ahtiainen J, Paavolainen L. Neuromuscular adaptations during concurrent strength and endurance training versus strength training. Eur J Appl Physiol 2003;89:42-52. [Crossref] [PubMed]
- Ferreira LF, de Oliveira AR, Schiefelbein ML, Garcia E, da Rosa LHT. Aerobic Training Does not Decrease the Prevalence of Sarcopenia in Older Women: Cross-Sectional Study. Ageing International 2023;48:563-74.
- Schweitzer L, Geisler C, Pourhassan M, Braun W, Glüer CC, Bosy-Westphal A, Müller MJ. What is the best reference site for a single MRI slice to assess whole-body skeletal muscle and adipose tissue volumes in healthy adults? Am J Clin Nutr 2015;102:58-65. [Crossref] [PubMed]
- Si F, Wang T, Zang L, Yuan S, Du P, Fan N, Wu Q, Wang A. Characteristics of paraspinal muscle fat infiltration in asymptomatic Chinese adults: a cross-sectional study. Quant Imaging Med Surg 2023;13:4526-39. [Crossref] [PubMed]
- Ema R, Sakaguchi M, Kawakami Y. Thigh and Psoas Major Muscularity and Its Relation to Running Mechanics in Sprinters. Med Sci Sports Exerc 2018;50:2085-91. [Crossref] [PubMed]
- Hodges PW, Danneels L. Changes in Structure and Function of the Back Muscles in Low Back Pain: Different Time Points, Observations, and Mechanisms. J Orthop Sports Phys Ther 2019;49:464-76. [Crossref] [PubMed]
- Özcan-Ekşi EE, Ekşi MŞ, Turgut VU, Canbolat Ç, Pamir MN. Reciprocal relationship between multifidus and psoas at L4-L5 level in women with low back pain. Br J Neurosurg 2021;35:220-8. [Crossref] [PubMed]
- Muellner M, Haffer H, Chiapparelli E, Dodo Y, Shue J, Tan ET, Zhu J, Pumberger M, Sama AA, Cammisa FP, Girardi FP, Hughes AP. Fat infiltration of the posterior paraspinal muscles is inversely associated with the fat infiltration of the psoas muscle: a potential compensatory mechanism in the lumbar spine. BMC Musculoskelet Disord 2023;24:846. [Crossref] [PubMed]
- Kircher K, Chaudry O, Nagel AM, Ghasemikaram M, Uder M, Jakob F, Kohl M, Kemmler W, Engelke K. Effects of high-intensity training on fatty infiltration in paraspinal muscles in elderly males with osteosarcopenia - the randomized controlled FrOST study. BMC Geriatr 2024;24:141. [Crossref] [PubMed]
- Chen Y, Feng X, Huang L, Wang K, Mi J. Comparative efficacy of concurrent training types on lower limb strength and muscular hypertrophy: A systematic review and network meta-analysis. J Exerc Sci Fit 2024;22:86-96. [Crossref] [PubMed]
- Fry AC. The role of resistance exercise intensity on muscle fibre adaptations. Sports Med 2004;34:663-79. [Crossref] [PubMed]