Comparison of multi-phase contrast-enhanced T1-weighted volumetric interpolated breath-hold examination and fat-suppressed T2-weighted combined with diffusion-weighted magnetic resonance imaging in anal fistula evaluation
Introduction
Anal fistula represents a prevalent anorectal condition with a global incidence of 1–2 cases per 10,000, significantly impairing patient quality of life due to symptoms such as anal discomfort, pruritus, discharge of pus or blood, and occasionally, defecation difficulties (1). Surgical intervention remains the main treatment for anal fistula. However, the risk of postoperative recurrence and potential damage to the anal sphincter are concerns, often attributed to inadequate preoperative evaluation (2-4). Precise preoperative determination of the fistula’s characteristics, including the location, number of internal openings, presence of secondary tracts, and abscesses, is crucial for tailoring surgical approaches, thereby enhancing patient outcomes and minimizing disease recurrence (5).
Magnetic resonance imaging (MRI) has been reported to reduce the recurrence of anal fistula by up to 75% (6,7). Traditional MRI sequences for this condition typically encompass T2-weighted imaging (T2WI), fat-suppressed T2WI (FS-T2WI), and fat-suppressed T1-weighted imaging (FS-T1WI). Nonetheless, these conventional MRI sequences exhibit limitations. The secretion-filled nature of anal fistulas and surrounding perianal structures yield intense signals on T2WI, compromising image contrast and elevating the risk of misdiagnosis. Although fat signals suppression improves contrast of the fistula in FS-T2WI images, it detrimentally affects the delineation of perianal anatomy. Moreover, the FS-T2WI sequence demonstrates reduced sensitivity in detecting inactive or postoperative fistulas characterized by minimal secretion or scar formation, respectively.
Growing evidence indicates that the integration of diffusion-weighted imaging (DWI) with contrast-enhanced (CE) T1W1 improves the visibility of fistulas, serving as a pivotal modality for identifying acute inflammation (8-10). Meanwhile, the diagnostic accuracy of CE-FS-T1WI surpasses that of that of FS-T2WI sequences in characterizing the internal opening of anal fistulas (11). However, the relatively thick slices of two-dimensional (2D) imaging pose challenges in accurately depicting the internal opening and intricate secondary fistulas (11). The advent of 3.0T MRI technology, offering isotropic three-dimensional (3D) data acquisition with elevated spatial resolution, presents a promising avenue for the detection of anal fistula lesions (12,13). For routine diagnostic purposes, single time point 2D gradient echo (GRE) post-contrast T1 fat-suppressed imaging may be sufficient and more efficient. However, multi-phase 3D imaging is generally worth the extra time and effort when detailed anatomical and functional information is needed, particularly for evaluating the intricate details of fistulas and planning surgical interventions. The enhanced spatial resolution and dynamic information obtained from multi-phase imaging allow for precise evaluation of the path, extent, and relationship of fistulas to surrounding tissues, which is crucial for effective surgical planning, even if it takes extra time (13-15). Meanwhile, the efficacy of combining multi-phase CE fat-suppressed (FS) T1WI with 3D GRE sequence volumetric interpolated breath-hold examination (VIBE) in potentially influencing the incidence of anal fistulas or affecting postoperative recurrence remains to be elucidated. Therefore, this study aimed to explore the utility of multi-phase contrast-enhanced fat-suppressed T1-weighted imaging using three-dimensional gradient echo sequence volumetric interpolated breath-hold examination (CE-FS-T1-3D-VIBE) in evaluating anal fistula postoperative prognosis, and its comparative effectiveness with combined FS-T2WI-DWI sequences. We present this article in accordance with the STROBE reporting checklist (available at https://qims.amegroups.com/article/view/10.21037/qims-24-490/rc).
Methods
Patients
This retrospective study was conducted in accordance with the Declaration of Helsinki (as revised in 2013). The study was approved by the Ethics Committee of the People’s Hospital of Liuyang (No. 2023-013). Since the MRI examinations require the intravenous injection of the contrast agent gadopentetate monoglucoside (Gd-DTPA), patients signed an informed consent form for the contrast injection prior to the MRI scan, and also provided consent for any surgeries or related procedures beforehand. This is a retrospective study, and an application to waive the requirement for informed consent for the retrospective collection of data was approved by the medical ethics committee after review. We initially retrieved 203 cases of anal fistula from the picture archiving and communication systems (PACS, Diagnostic imaging workstation; Neusoft Corporation, Shenyang, China). Following the application of inclusion and exclusion criteria, 168 patients with confirmed diagnoses of anal fistula were recruited in the Radiology Department of the People’s Hospital of Liuyang between November 2021 and November 2023, and all cases were assessed using MRI. The inclusion criterion was clinically diagnosed anal fistula that was subjected to MRI examination within seven working days before surgery. The clinical objective was to “cure while preserving anal function”. Treatment options were selected or combined based on the specific condition of the patient and can be categorized into surgeries that damage the sphincter and those that preserve sphincter function. The former category included procedures such as fistulectomy, fistulotomy, and the use of cutting setons, whereas the latter included the ligation of the intersphincteric fistula tract (LIFT) procedure and the endorectal advancement flap, among others. The exclusion criteria were as follows: patients with Crohn’s disease (CD) resulting in fistula, hidradenitis suppurativa, septic sweater, atypical infected lesions with anal fistula formation [in human immunodeficiency virus (HIV), tuberculosis (TB), and actinomycosis], immunocompromised patients (e.g., neutropenic patients undergoing chemotherapy to treat solid-organ malignancies, or patients with hematological malignancies) (Figure 1).
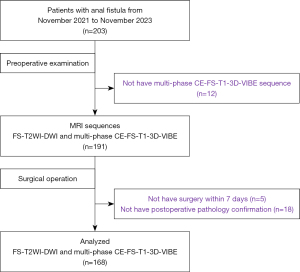
MRI techniques
A Siemens 3.0T magnetic resonance scanner (Skyra 3.0T superconducting type; Siemens AG, Erlangen, Germany) was used in all patients to obtain a high signal-to-noise ratio (SNR) and small field of view (FOV). An 18-channel body coil was used, and no special bowel preparation was required before the examination. The patient was placed in a supine position with the head advanced, and the pubic symphysis was used as the center of the positioning. In order to accurately locate the center of the magnetic field in the anal canal region, pelvic sagittal imaging was performed first to identify the location of the anal canal structures and initially determine the lesions, and then small FOV thin-layer oblique axial and oblique coronal imaging of the anal canal region was performed perpendicular and parallel to the anal canal. The scanning sequence included T2WI sagittal, FS-T2WI oblique coronal, T1WI oblique axial, and FS-T2WI oblique axial, followed by DWI oblique axial scanning. DWI was obtained using an echo planar single-shot spin echo sequence with b values of 50 and 800 s/mm2. The apparent diffusion coefficient (ADC) values were automatically calculated using a mono-exponential fitting algorithm, generating the corresponding ADC images. Finally, the acquisition of 1+5 phase FS-T1-3D-VIBE transverse axial enhancement scanning sequence was completed. The enhancement scanning was performed using the multi-phase enhancement 3D GRE VIBE acquisition technique sequence which was performed firstly by pre-scanning, and then the first period scanning was acquired after injecting the contrast agent for 20 seconds via the elbow vein using a high-pressure syringe, the second period after 55 seconds, the third period after 100 seconds, the fourth period after 145 seconds, and the fifth period after 215 seconds, with a temporal resolution of 20 seconds, involving a 1+5 phase enhanced FS-T1-3D-VIBE oblique axial sequence that took a total of 255 seconds. The contrast agent, Gd-DTPA, was administered using a high-pressure syringe at an injection rate of 2.5 mL/s and a dose of 0.1 mg/kg, followed by a 20 mL saline flush.
Examination sequence
The examination sequence and parameters are detailed in Table 1. The sequences are as follows:
Table 1
Type | T2WI | T2WI | T1WI | T2WI | DWI | T1-3D-VIBE (1+5) |
---|---|---|---|---|---|---|
Acquisition plane | Sagittal | FS + oblique coronal | Oblique axial | FS + oblique axial | Oblique axial | FS + oblique axial |
TR (ms) | 6,000 | 5,800 | 700 | 9,090 | 4,300 | 3.23 |
TE (ms) | 112 | 86 | 12 | 85 | 59 | 1.22 |
Flip angle (°) | 160 | 160 | 160 | 160 | – | 9 |
Bandwidth (Hz/pixel) | 200 | 200 | 170 | 200 | 1566 | 520 |
Slice thickness/gap (mm) | 4/0.4 | 4/0.4 | 3/0.3 | 3/0.3 | 3/0.3 | 1.5/0.03 |
Number of slices | 23 | 23 | 25 | 25 | 25 | 104 |
Acquisition matrix | 320×320 | 320×256 | 320×240 | 320×256 | 114×114 | 320×240 |
Voxel size (mm) | 0.6×0.6×3.0 | 0.6×0.6×3.0 | 0.75×0.75×4.0 | 0.75×0.75×3.0 | 1.05×1.05×3.0 | 1.2×1.2×1.5 |
Field of view (mm) | 200 | 260 | 240 | 240 | 240 | 380 |
Acquisition time (min-sec) | 1 min 56 s | 1 min 45 s | 1 min 35 s | 1 min 59 s | 1 min 59 s | 4 min 15 s |
MRI, magnetic resonance imaging; T2WI, T2-weighted imaging; T1WI, T1-weighted imaging; DWI, diffusion-weighted imaging; T1-3D-VIBE, T1-weighted imaging using three-dimensional volumetric interpolated breath-hold examination; FS, fat-suppressed; TR, repetition time; TE, echo time.
T2WI sagittal sequence repetition time (TR): 6,000 ms and echo time (TE): 112 ms, FS-T2WI oblique coronal sequence: TR 5,800 ms and TE 86 ms, T1WI oblique axial sequence: TR 700 ms and TE 12 ms, FS-T2WI oblique axial sequence: TR 9,090 ms and TE 85 ms, DWI oblique axial sequence: TR 4,300 ms and TE 59 ms. The slice thickness for all these sequences was 3–4 mm. Additionally, the T1-3D-VIBE (1+5) oblique axial sequence had a TR of 3.23 ms, a TE of 1.22 ms, and a slice thickness of 1.5 mm.
MRI evaluation
The MRI sequences of FS-T2WI-DWI and multi-phase CE-FS-T1-3D-VIBE were evaluated independently by two senior radiologists with more than 10 years of diagnostic experience in anal fistula in MRI. The radiologists were blinded to the clinical history, surgical results, blood tests, endoscopy findings, and follow-up MRI examinations. All MRI evaluations were conducted on the same PACS observatory using a standardized form specifically created for this study, which included detailed information described later. The sequences were retrieved and adjusted by another physician. Initially, only the FS-T2WI-DWI oblique axial sequences were assessed, followed by the assessment of only the multiphase CE-FS-T1-3D-VIBE sequences four weeks later. Both radiologists used the same procedure for their evaluations, with each assessment completed within one week to minimize reading bias. If any discrepancies were encountered in the results, a final judgement was made by a physician with more than 20 years of extensive diagnostic experience in MRI. The time needed for evaluation ranged from 5 to 20 minutes, depending on the complexity of each case. The fistula classification (Parks’ classification), location and number of internal openings, number of primary tracts, secondary tracts, accompanying abscesses, and horseshoe extensions were observed or measured on FS-T2WI-DWI and multi-phase CE-FS-T1-3D-VIBE sequences (16). Tubular lesions less than 10 mm in diameter were classified as fistula tracts, whereas fluid collections or extensions of the fistula greater than 10 mm in diameter were identified as abscesses (17,18). The position of the internal opening was described using the “anal clock” method. The internal opening clarity was scored by 1–4 points (19): 1, the display of no obvious internal opening or fistula; 2, the display of a vaguely visible probable internal opening or fistula; 3, the display of a basically clear visible internal opening or fistula; 4, a clear internal opening or fistula display, a clear relationship of the lesion to the surrounding sphincter or an increased display with clear detail. The external opening was not considered an imaging observation as it can be directly observed during surgery. The clinician performed the procedure with a thorough evaluation, including: MRI and other relevant examination information, visualization, palpation, and methylene blue dye exploration, and recorded the results of the procedure.
Statistical analysis
SPSS 25.0 software (IBM Corp., Armonk, NY, USA) was used for data analysis and descriptive statistics were shown as mean ± standard deviation. The agreement between the two radiologists in the intra- and inter-observer definition of internal opening scores was assessed by weighted Kappa statistic. κ values were interpreted as follows: poor (κ<0.1), slight (0.1≤κ<0.2), fair (0.2≤κ<0.4), moderate (0.4≤κ<0.6), good (0.6≤κ<0.8), and excellent (0.8≤κ≤1) (20). The clarity scores of internal opening fistulae using the FS-T2WI+DWI and multi-phase CE-FS-T1-3D-VIBE sequences were analyzed by χ2 test. The accuracy of location and number of internal orifices, primary fistula, secondary fistula, anal fistula classification, abscess, and horseshoe fistula in the FS-T2WI + DWI and multi-phase CE-FS-T1-3D-VIBE sequences were analyzed by χ2 test. A P value <0.05 was considered a statistically significant difference in the one-tailed test.
Results
A cohort of 168 patients with anal fistula were analyzed in this study, comprising 149 males (88.7%) and 19 females (11.3%), with a mean age of 38.0 years (range, 14–70 years).
Surgical findings revealed a total of 227 internal openings: a single internal opening in 129 cases (76.79%), two internal openings in 29 cases (17.26%), and more than two internal openings in 10 cases (5.95%). Additionally, there were 227 primary fistulas and 99 secondary fistulas, including 27 horseshoe fistulas and 127 concurrent abscesses. Classification according to the Parks criteria yielded 99 cases (58.93%) of the intersphincteric type, 53 cases (31.55%) of transsphincteric type, 12 cases (7.14%) of the suprasphincteric type, and 4 cases (2.38%) of extrasphincteric type.
Inter-observer agreement on the clarity scores for the internal opening and fistulae of anal fistulas was substantial, with Kappa values indicating good consistency (FS-T2WI + DWI for internal opening clarity, κ=0.788; multi-phase CE-FS-T1-3D-VIBE for internal opening clarity, κ=0.785; FS-T2WI+DWI for fistula clarity, κ=0.779; multi-phase CE-FS-T1-3D-VIBE for fistula clarity, κ=0.784).
The clarity of the internal opening and primary fistula is critical for surgical planning. Comparative analysis of the FS-T2WI-DWI and multi-phase CE-FS-T1-3D-VIBE sequences for diagnosing internal clarity (Table 2, Figure 2) demonstrated a significant superiority of the multi-phase CE-FS-T1-3D-VIBE sequence in delineating internal clarity (P=0.013). Similarly, when comparing the two sequences for primary fistula tract clarity (Table 3, Figure 3), the multi-phase CE-FS-T1-3D-VIBE sequence again showed significant superiority (P<0.001).
Table 2
Score | Multi-phase CE-FS-T1-3D-VIBE | FS-T2WI-DWI | χ2 | P value |
---|---|---|---|---|
1 | 6 | 8 | ||
2 | 19 | 30 | ||
3 | 63 | 73 | ||
4 | 80 | 57 | ||
Total | 168 | 168 | 6.225 | 0.013 |
FS-T2WI-DWI, fat-suppressed T2-weighted imaging combined with diffusion-weighted imaging; CE-FS-T1-3D-VIBE, contrast-enhanced fat-suppressed T1-weighted imaging using three-dimensional volumetric interpolated breath-hold examination.
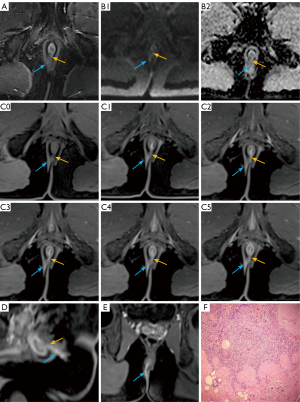
Table 3
Score | Multi-phase CE-FS-T1-3D-VIBE | FS-T2WI-DWI | χ2 | P value |
---|---|---|---|---|
1 | 5 | 5 | ||
2 | 8 | 21 | ||
3 | 48 | 79 | ||
4 | 107 | 63 | ||
Total | 168 | 168 | 16.576 | <0.001 |
FS-T2WI-DWI, fat-suppressed T2-weighted imaging combined with diffusion-weighted imaging; CE-FS-T1-3D-VIBE, contrast-enhanced fat-suppressed T1-weighted imaging using three-dimensional volumetric interpolated breath-hold examination.
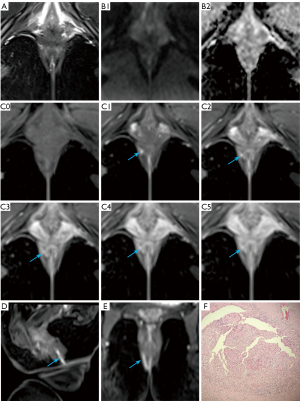
Further analysis was conducted to ascertain the multi-phase CE-FS-T1-3D-VIBE sequence’s efficacy in evaluating the internal position, number of internal openings, primary fistula tracts, secondary tracts, and fistula classification (Table 4). The findings indicated that the multi-phase CE-FS-T1-3D-VIBE sequence provided superior visualization of the internal opening location [86.31% vs. 77.38%, 95% confidence interval (CI): 0.307–0.959, P=0.034, Figure 4] and clarity of secondary tracts (88.69% vs. 80.95%, 95% CI: 0.293–1.001, P=0.048, Figure 5) compared to the FS-T2WI-DWI sequence.
Table 4
Characteristics | Correct, n (%) | Incorrect, n (%) | χ2 | P value |
---|---|---|---|---|
Internal position | 4.507 | 0.034 | ||
FS-T2WI-DWI | 130 (77.38) | 38 (22.62) | ||
Multi-phase CE-FS-T1-3D-VIBE | 145 (86.31) | 23 (13.69) | ||
Number of internal opening | 2.211 | 0.137 | ||
FS-T2WI-DWI | 148 (88.10) | 20 (11.90) | ||
Multi-phase CE-FS-T1-3D-VIBE | 156 (92.86) | 12 (7.14) | ||
Primary tract | 1.403 | 0.236 | ||
FS-T2WI-DWI | 151 (89.88) | 17 (10.12) | ||
Multi-phase CE-FS-T1-3D-VIBE | 157 (93.45) | 11 (6.55) | ||
Secondary tracts | 3.907 | 0.048 | ||
FS-T2WI-DWI | 136 (80.95) | 32 (19.05) | ||
Multi-phase CE-FS-T1-3D-VIBE | 149 (88.69) | 19 (11.31) | ||
Fistula classification | 3.262 | 0.071 | ||
FS-T2WI-DWI | 150 (89.29) | 18 (10.71) | ||
Multi-phase CE-FS-T1-3D-VIBE | 159 (94.64) | 9 (5.36) | ||
Abscesses | 1.549 | 0.213 | ||
FS-T2WI-DWI | 157 (93.45) | 11 (6.55) | ||
Multi-phase CE-FS-T1-3D-VIBE | 162 (96.43) | 6 (3.57) | ||
Horseshoe fistulas | 0.336 | 0.562 | ||
FS-T2WI-DWI | 166 (98.81) | 2 (1.19) | ||
Multi-phase CE-FS-T1-3D-VIBE | 167 (99.40) | 1 (0.60) |
FS-T2WI-DWI, fat-suppressed T2-weighted imaging combined with diffusion-weighted imaging; CE-FS-T1-3D-VIBE, contrast-enhanced fat-suppressed T1-weighted imaging using three-dimensional volumetric interpolated breath-hold examination.
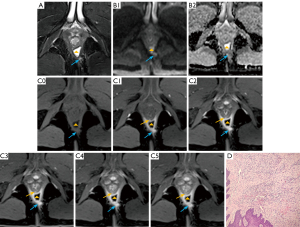
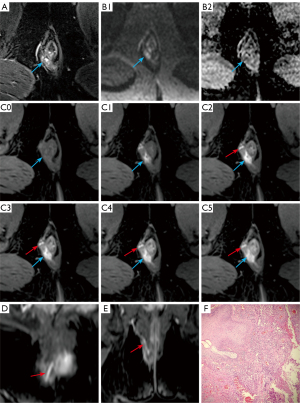
Although the multi-phase CE-FS-T1-3D-VIBE sequence exhibited higher accuracy in depicting the number of internal openings, primary tract, and fistula classification than the FS-T2WI-DWI sequence, these differences did not reach statistical significance.
For the assessment of abscesses, the diagnostic accuracies of the multi-phase CE-FS-T1-3D-VIBE and FS-T2WI-DWI MRI sequences were recorded at 96.43% and 93.45%, respectively. Regarding the detection of horseshoe fistulas, accuracies were noted at 99.40% for the multi-phase CE-FS-T1-3D-VIBE sequence and 98.81% for the FS-T2WI-DWI sequence (Table 4, Figure 5). Statistical analysis revealed no significant differences in the diagnostic performance of the two sequences for both abscesses and horseshoe fistulas, indicating comparable diagnostic efficacy.
In the evaluation of the anal canal mucosa during the early arterial phase (55–75 s), multi-phase CE-FS-T1-3D-VIBE sequence afforded a clear visualization. The appearance of the mucosa varies with anatomical location; specifically, within the mid-anal canal at the level of the dentate line, the mucosa presents as irregular, sparse, and flattened, adopting an “X” shape in the imaging (Figure 6).
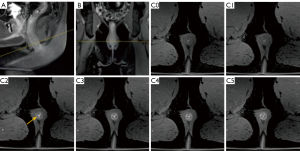
Discussion
In the present study, the multi-phase CE-FS-T1-3D-VIBE sequence demonstrated notable advantages over the FS-T2WI-DWI sequence in the evaluation of anal fistulas. Specifically, the multi-phase CE-FS-T1-3D-VIBE sequence, characterized by its shorter phase resolution time, reduced slice thickness, and enhanced micro structural visualization, significantly improved the delineation of internal openings, main fistula tracts, and secondary tracts. This enhancement in image quality is pivotal for surgical planning and prognostication in anal fistula management.
Traditional MRI sequences, including T1WI, have been instrumental in visualizing the anatomy of the sphincter complex, levator ani muscle, and ischiorectal fossa. However, the differentiation between fistulas, abscesses, and normal musculature remains challenging due to the low to moderate signal intensity of these pathological entities. The introduction of FS-T2WI and DWI has improved the contrast of active fistulas against adjacent tissues (17,21). DWI, in particular, has emerged as a valuable tool for the diagnosis of anal fistulas, offering enhanced signal intensity and contrast. Furthermore, the application of DWI in patients with renal insufficiency or contrast allergies underscores its utility in increasing diagnostic confidence (9,22).
Despite these advancements, DWI is not without limitations, particularly in the evaluation of perianal regions where artifacts from the air-soft tissue interface can compromise image quality (23,24). It may produce false positives due to conditions such as abscesses that cause restricted diffusion, or false negatives if lesions are too small or do not restrict diffusion significantly. Differentiating scar tissue from recurrent disease can be challenging with DWI, as both may display similar diffusion characteristics. Conversely, CE with FS T1WI has shown efficacy in clearly delineating primary and secondary fistulas, as well as their relationship to the anal sphincter complex, thus becoming a preferred method for MRI evaluation of anal fistulas (11). Nevertheless, the slice thickness inherent to this technique may obscure fine details of internal openings and secondary tracts, potentially overlooking minute lesions (5).
The advent of 3.0 T MRI with isotropic 3D data acquisition offers a solution to these limitations, providing high spatial resolution crucial for detecting anal fistula lesions. The VIBE sequence, in particular, stands out for its ability to produce T1-weighted images with high contrast and spatial resolution within a relatively short acquisition time. This capability is further enhanced by the sequence’s high SNR and contrast-to-noise ratio (CNR), facilitating the accurate diagnosis of fistulas with a small FOV and layer thicknesses ranging between 1–2 mm (17,25,26).
The anatomical origin of anal fistulas, typically from the dentate line, underscores the importance of accurately visualizing this region for surgical intervention. The multi-phase CE-FS-T1-3D-VIBE sequence’s ability to morphologically differentiate the anal canal epithelium, particularly above the dentate line, represents a significant advancement in the preoperative assessment of anal fistulas (16,27). This multi-phase contrast-enhanced MRI technique, by highlighting the early arterial phase, offers a clear visualization of the mucosal layer and its secretions, thereby facilitating a more precise determination of the internal opening’s position and number.
Accurately determining the location, number, and course of the internal orifice, fistula, and perianal abscess in anal fistula cases—particularly in complex anal fistulas—is crucial for selecting the appropriate surgical procedure, ensuring effective treatment, and reducing postoperative recurrence (6,28,29). The ability to improve soft tissue differentiation and visualize fine anatomical structures, along with the non-invasive and direct identification of internal orifices, fistulas, and abscess cavities, holds significant value in the assessment of anal fistulas. The multi-phase CE-FS-T1-3D-VIBE sequence, compared to conventional MRI sequences and DWI sequences, offers thinner slice thickness and clearer visualization of subtle structures. This results in enhanced clarity in identifying the internal orifice of the fistula and the main fistula. Additionally, the sequence allows for image reconstruction using multiplanar reformation (MPR) in all phases, providing a more detailed and multidirectional view of the lesion’s morphology and its anatomical relationship with the perianal structures. This technique can clearly reveal some internal orifices and small secondary fistulas that conventional sequences might miss, thereby significantly reducing diagnostic omissions and improving the accuracy of sphincter assessment, ultimately contributing to the effective treatment and reduced recurrence of anal fistulas after surgery.
Multi-phase scanning is used to dynamically display the structure of the anal canal, the pathology of anal fistulas, and the relationship between the two. Scanning only one or two phases would not adequately demonstrate the dynamic enhancement patterns of the anal canal structures and lesions. For example, multi-phase enhanced imaging during the early arterial phase can clearly show the prominently enhanced dentate line, whereas the enhancement of the internal anal sphincter is relatively delayed. Acute lesions may show continuous enhancement, whereas chronic lesions might exhibit progressive enhancement changes. Therefore, we believe that if feasible, conducting multi-phase enhanced scans to illustrate the relationships between acute and chronic lesions and the anatomy of the anus is necessary. Based on our team’s research, most anal fistula cases are clearly visible during the third or fourth phase. Therefore, to reduce examination time, we recommend conducting at least two phases of scans after injecting the contrast agent: the second phase (55–75 s), third phase (100–120 s), or fourth phase (145–165 s) (17,23,30).
Our findings indicate that the multi-phase CE-FS-T1-3D-VIBE sequence not only surpasses the FS-T2WI-DWI sequence in visualizing the clarity and location of internal openings and secondary tracts but also matches its efficacy in identifying the number of internal openings, main tracts, and fistula classification. Moreover, this sequence demonstrates comparable diagnostic performance in detecting abscesses and horseshoe fistulas, suggesting its broader applicability in anal fistula evaluation.
However, there are some limitations in this research. As a retrospective study, this research is susceptible to both selection and information biases, underscoring the need for well-designed prospective studies to corroborate these findings. Although surgical outcomes served as the reference standard, there is a possibility that some small fistulas and associated lesions were not detected during the surgical procedure. Furthermore, the brief four-week interval between datasets and the use of similar cohorts may have introduced recall bias, and there is potential for diagnostic bias in the lesion assessments made by the two observers.
Conclusions
The multi-phase CE-FS-T1-3D-VIBE sequence represents a superior diagnostic tool for the evaluation of anal fistulas, offering enhanced clarity and accuracy in identifying crucial anatomical features. This advancement holds significant potential for improving surgical outcomes and reducing recurrence rates in anal fistula treatment.
Acknowledgments
Funding: This study was supported by
Footnote
Reporting Checklist: The authors have completed the STROBE reporting checklist. Available at https://qims.amegroups.com/article/view/10.21037/qims-24-490/rc
Conflicts of Interest: All authors have completed the ICMJE uniform disclosure form (available at https://qims.amegroups.com/article/view/10.21037/qims-24-490/coif). The authors have no conflicts of interest to declare.
Ethical Statement: The authors are accountable for all aspects of the work in ensuring that questions related to the accuracy or integrity of any part of the work are appropriately investigated and resolved. The study was conducted in accordance with the Declaration of Helsinki (as revised in 2013). The study was approved by the Ethics Committee of the People’s Hospital of Liuyang (No. 2023-013) and the requirement for individual consent for this retrospective analysis was waived.
Open Access Statement: This is an Open Access article distributed in accordance with the Creative Commons Attribution-NonCommercial-NoDerivs 4.0 International License (CC BY-NC-ND 4.0), which permits the non-commercial replication and distribution of the article with the strict proviso that no changes or edits are made and the original work is properly cited (including links to both the formal publication through the relevant DOI and the license). See: https://creativecommons.org/licenses/by-nc-nd/4.0/.
References
- Vogel JD, Johnson EK, Morris AM, Paquette IM, Saclarides TJ, Feingold DL, Steele SR. Clinical Practice Guideline for the Management of Anorectal Abscess, Fistula-in-Ano, and Rectovaginal Fistula. Dis Colon Rectum 2016;59:1117-33. [Crossref] [PubMed]
- Mei Z, Li Y, Zhang Z, Zhou H, Liu S, Han Y, Du P, Qin X, Shao Z, Ge M, Wang Q, Yang W. Development of screening tools to predict the risk of recurrence and related complications following anal fistula surgery: protocol for a prospective cohort study. BMJ Open 2020;10:e035134. [Crossref] [PubMed]
- He Z, Du J, Wu K, Chen J, Wu B, Yang J, Xu Z, Fu Z, Pan L, Wen K, Wang X. Formation rate of secondary anal fistula after incision and drainage of perianal Sepsis and analysis of risk factors. BMC Surg 2020;20:94. [Crossref] [PubMed]
- Kummari S, Burra KG, Reddy VRK, Das S, Ramadugu R, Ramadugu S. The Role of Magnetic Resonance Imaging in Pre-operative Assessment of Anorectal Fistula With Surgical Correlation. Cureus 2024;16:e53237. [Crossref] [PubMed]
- Zhao J, Lu F, Wang Q, Lu H, Liu M, Zhang Z, Gong Z, Huang Y, Yang W, Zhan S, Yang S. Comparison of contrast-enhanced fat-suppressed T1-3D-VIBE and T1-TSE MRI in evaluating anal fistula. Abdom Radiol (NY) 2022;47:3688-97. [Crossref] [PubMed]
- Mullen R, Deveraj S, Suttie SA, Matthews AG, Yalamarthi S. MR imaging of fistula in ano: indications and contribution to surgical assessment. Acta Chir Belg 2011;111:393-7. [Crossref] [PubMed]
- Buchanan G, Halligan S, Williams A, Cohen CR, Tarroni D, Phillips RK, Bartram CI. Effect of MRI on clinical outcome of recurrent fistula-in-ano. Lancet 2002;360:1661-2. [Crossref] [PubMed]
- Liu X, Wang Z, Ren H, Ren A, Wang W, Yang X, Shi S. Evaluating postoperative anal fistula prognosis by diffusion-weighted MRI. Eur J Radiol 2020;132:109294. [Crossref] [PubMed]
- Anwar HA, Reddy MY, Kumar S, Durai K. V V, Kumar R. A study of the diagnostic efficacy of diffusion-weighted magnetic resonance imaging in the diagnosis of perianal fistula and its complications. Pol J Radiol 2023;88:e113-8. [Crossref] [PubMed]
- Aggarwal P, Malik R, Sarawagi R, Kumar A, Sharma J. Diffusion-Weighted MRI in Perianal Abscess: Role and Comparison With Contrast-Enhanced MRI. Cureus 2024;16:e59035. [Crossref] [PubMed]
- Das GC, Chakrabartty DK. Best non-contrast magnetic resonance imaging sequence and role of intravenous contrast administration in evaluation of perianal fistula with surgical correlation. Abdom Radiol (NY) 2021;46:469-75. [Crossref] [PubMed]
- Cerit MN, Öner AY, Yıldız A, Cindil E, Şendur HN, Leventoğlu S. Perianal fistula mapping at 3 T: volumetric versus conventional MRI sequences. Clin Radiol 2020;75:563.e1-9. [Crossref] [PubMed]
- Zhang L, Zhou N, Yang H, Jin M, Wei X, Huang G, Zhang S. Re-evaluating the value of preoperative magnetic resonance imaging for anal fistula with an analysis for misdiagnosis and missed diagnosis of in a large cohort. Minerva Med 2024;115:400-3. [Crossref] [PubMed]
- Rofsky NM, Lee VS, Laub G, Pollack MA, Krinsky GA, Thomasson D, Ambrosino MM, Weinreb JC. Abdominal MR imaging with a volumetric interpolated breath-hold examination. Radiology 1999;212:876-84. [Crossref] [PubMed]
- AlObaidy M, Ramalho M, Busireddy KK, Liu B, Burke LM, Altun E, Dale BM, Semelka RC. High-resolution 3D-GRE imaging of the abdomen using controlled aliasing acceleration technique - a feasibility study. Eur Radiol 2015;25:3596-605. [Crossref] [PubMed]
- Parks AG, Gordon PH, Hardcastle JD. A classification of fistula-in-ano. Br J Surg 1976;63:1-12. [Crossref] [PubMed]
- Torkzad MR, Ahlström H, Karlbom U. Comparison of different magnetic resonance imaging sequences for assessment of fistula-in-ano. World J Radiol 2014;6:203-9. [Crossref] [PubMed]
- Erden A. MRI of anal canal: normal anatomy, imaging protocol, and perianal fistulas: Part 1. Abdom Radiol (NY) 2018;43:1334-52. [Crossref] [PubMed]
- Hori M, Oto A, Orrin S, Suzuki K, Baron RL. Diffusion-weighted MRI: a new tool for the diagnosis of fistula in ano. J Magn Reson Imaging 2009;30:1021-6. [Crossref] [PubMed]
- Landis JR, Koch GG. An application of hierarchical kappa-type statistics in the assessment of majority agreement among multiple observers. Biometrics 1977;33:363-74.
- Baik J, Kim SH, Lee Y, Yoon JH. Comparison of T2-weighted imaging, diffusion-weighted imaging and contrast-enhanced T1-weighted MR imaging for evaluating perianal fistulas. Clin Imaging 2017;44:16-21. [Crossref] [PubMed]
- Boruah DK, Hazarika K, Ahmed H, Borah KK, Borah S, Malakar S, Hajoari N. Role of Diffusion-Weighted Imaging in the Evaluation of Perianal Fistulae. Indian J Radiol Imaging 2021;31:91-101. [Crossref] [PubMed]
- Liu X, Wang Z, Ren H, Wang Z, Li J. Accuracy of magnetic resonance imaging in defining dentate line in anal fistula. BMC Med Imaging 2022;22:201. [Crossref] [PubMed]
- Jit I. Anatomy of the rectum and anal canal of the rhesus monkey (Macaca mulatta). J Anat 1974;117:271-9.
- Kataoka M, Ueda H, Koyama T, Umeoka S, Togashi K, Asato R, Tanaka S, Ito J. Contrast-enhanced volumetric interpolated breath-hold examination compared with spin-echo T1-weighted imaging of head and neck tumors. AJR Am J Roentgenol 2005;184:313-9. [Crossref] [PubMed]
- Li CX, Tang HH, Guo HL, Chen DW, Ran XW. Optimal protocol of MR contrast imaging in diabetic foot. Sichuan Da Xue Xue Bao Yi Xue Ban 2012;43:752-6.
- Hutan M, Hutan M Jr, Satko M, Dimov A. Significance of MRI in the treatment of perianal fistula. Bratisl Lek Listy 2009;110:162-5.
- Iqbal N, Tozer PJ, Fletcher J, Lightner AL, Sackitey C, Corr A, Patel U, Ilangovan R, Lung P. Getting the most out of MRI in perianal fistula: update on surgical techniques and radiological features that define surgical options. Clin Radiol 2021;76:784.e17-25. [Crossref] [PubMed]
- Bakhtawar N, Usman M. Factors Increasing the Risk of Recurrence in Fistula-in-ano. Cureus 2019;11:e4200. [Crossref] [PubMed]
- Tatsumoto S, Itoh T, Takahama J, Marugami N, Minamiguchi K, Tanaka T, Koyama F, Morita K, Kichikawa K. Accuracy of magnetic resonance imaging in predicting dentate line invasion in low rectal cancer. Jpn J Radiol 2020;38:539-46. [Crossref] [PubMed]